Drug Dosing in Renal and Hepatic Failure: A Pharmacokinetic Approach to the Critically ILL Patient
Sonia Lin
Keith J. Foster
Ronald J. Debellis
Brian S. Smith
Estimates of the incidence of preventable adverse drug events in the intensive care unit (ICU) range from 10 up to 40 per 1,000 patient-days [1,2]. Patients in an ICU are approximately twice as likely to experience an adverse drug event when compared with patients in a general medicine unit. This increased risk is likely a result of the greater number of medical problems faced by patients in the ICU plus their wider range of drug exposures. Critically ill patients are also at increased risk for developing renal dysfunction, with acute kidney injury (AKI) occurring in 7% to 25% of all patients admitted to the ICU. AKI in the ICU is associated with a severalfold increase in mortality [3,4]. Renal injury is also a risk factor for adverse drug events. As many as 45% of patients with an estimated creatinine clearance less than 40 mL per minute receive medications that are dosed as much as 2.5 times higher than the maximum recommended dose [5]. In addition, adverse drug reactions occur in approximately 9% of patients with blood urea nitrogen less than 20 mg per dL versus 24% of patients with blood urea nitrogen greater than 40 mg per dL [6]. Adverse drug events not only place patients at increased risk for morbidity and mortality but also have a tremendous impact financially. It has been estimated that each adverse drug event increases hospital costs by $2,000 to $4,600 [7,8,9]. For all of these reasons, appropriate drug dosing in critically ill patients with kidney or liver injury is essential. The following review uses pharmacokinetic principles to discuss key concepts of drug dosing in critically ill patients with renal and hepatic dysfunction and provides drug dosage tables to assist clinicians with dosage adjustments in the setting of renal or hepatic disease (Tables 74.1 and 74.2).
Pharmacokinetic and Pharmacodynamic Principles
To design an effective and safe medication regimen, a clinician must have a general understanding of a drug’s pharmacokinetic and pharmacodynamic characteristics and be able to adjust for changes in the drug’s disposition that occur with critical illness, AKI, and hepatic dysfunction. Pharmacokinetics relates to the principles of drug absorption, distribution, metabolism, and excretion, whereas pharmacodynamics describes the pharmacologic response resulting from the drug at the site of action (receptor). Clinical pharmacokinetics is the application of knowledge of drug absorption, distribution, metabolism, and excretion to design patient-specific drug regimens with the goal of maximizing therapeutic outcomes and minimizing toxicity (Fig. 74.1).
Most drugs used in critically ill patients are metabolized with linear, or first-order, pharmacokinetics. This means that the drug is eliminated from plasma at a constant rate. As the plasma concentration increases or decreases, the amount of drug eliminated increases or decreases in a directly proportional relationship. Clinically, if a drug dose is increased, the plasma concentration increases proportionally, as does the amount eliminated (Fig. 74.2). If a drug’s plasma concentration is plotted versus time using a logarithmic scale, two different slopes are evident (Fig. 74.3): The upper portion is known as the alpha (or distribution) phase, which represents the process
of achieving equilibrium between the central and peripheral compartments. When monitoring serum drug concentrations, it is important to sample after the distribution phase is complete to avoid making decisions based on falsely elevated drug levels. The beta (or elimination) phase describes the section of the graph once distribution is completed. This phase represents drug elimination from the central compartment. The elimination rate constant (Kel) is obtained by calculating the slope of the line during the elimination phase, and it can be used to calculate a drug’s half-life (t1/2).
of achieving equilibrium between the central and peripheral compartments. When monitoring serum drug concentrations, it is important to sample after the distribution phase is complete to avoid making decisions based on falsely elevated drug levels. The beta (or elimination) phase describes the section of the graph once distribution is completed. This phase represents drug elimination from the central compartment. The elimination rate constant (Kel) is obtained by calculating the slope of the line during the elimination phase, and it can be used to calculate a drug’s half-life (t1/2).
Table 74.1 Guidelines for Drug Dosing in Critically ILL Patients with Renal Failure | |
---|---|
|
Table 74.2 Guidelines for Drug Dosing in Critically ILL Patients with Renal Failure | |
---|---|
|
![]() Figure 74.1. The relationship between pharmacokinetics and pharmacodynamics. [Adapted from Chernow B (ed): Critical Care Pharmacotherapy. Baltimore, MD, Williams & Wilkins, 1995, p 4.] |
Some drugs, such as phenytoin, follow zero-order or nonlinear kinetics. Zero-order, or Michaelis-Menten pharmacokinetics, refers to removal of a constant quantity of drug per unit of time. As the plasma concentration of the drug decreases or increases, the amount eliminated remains the same. This is the result of metabolism by a saturated enzyme system capable of eliminating drug only at a constant rate, regardless of the serum concentration. Clinically, this means small increases in the drug’s dose can lead to large increases in the plasma concentration; hence, the term nonlinear pharmacokinetics (Fig. 74.2).
Pharmacokinetic Terminology
The half-life of a medication is defined as the amount of time required for the concentration of the drug to decrease by 50% and is a function of drug metabolism and elimination. The half-life of a specific drug remains constant provided that the metabolizing and eliminating processes remain constant. If a patient’s renal or hepatic function declines, the half-life of the drug can be significantly prolonged.
The half-life of a medication can be used to determine the time required for a drug to reach steady state. Steady state is achieved when the amount of drug entering the body equals the amount eliminated, so plasma drug levels no longer increase. Steady-state conditions are achieved at a time approximately equal to four half-lives. A clinician should generally wait for steady state to be achieved before obtaining a drug serum
concentration or changing medication dose. Knowledge of a drug’s half-life may help estimate how long it should take for a pharmacologic or toxic effect to wear off. It is also important to be aware, however, that certain drugs (e.g., azithromycin) may be pharmacologically active longer than would be predicted from serum concentrations.
concentration or changing medication dose. Knowledge of a drug’s half-life may help estimate how long it should take for a pharmacologic or toxic effect to wear off. It is also important to be aware, however, that certain drugs (e.g., azithromycin) may be pharmacologically active longer than would be predicted from serum concentrations.
![]() Figure 74.3. Logarithm of plasma concentration (Cp) versus time plot for a drug after rapid intravenous injection, delineating the alpha distribution and beta elimination phases. |
The rate of drug elimination from the body is described as the Kel. With first-order elimination, a constant percentage of drug is removed from the plasma per unit of time and is often expressed as minutes– 1 or hours– 1. The Kel is also inversely proportional to the drug’s half-life. A drug’s Kel and half-life are constants and do not change unless the metabolizing or eliminating processes (or both) change.
Volume of distribution is not a physiologic volume but rather a theoretical volume that relates the plasma concentration to the administered dose. It is easiest to explain the concept of volume of distribution by providing an example. If a 700-mg dose of a drug administered as an intravenous bolus to 70-kg patient results in a calculated maximum plasma concentration of 7 mg per L, it appears as if the drug is dissolved in 100 L of fluid. The volume of distribution would be 100 L or 1.429 L per kg. Under normal physiologic conditions, however, a 70-kg adult does not have 100 L of body fluid. A large volume of distribution means that the amount of drug available to be measured in the plasma is reduced due to distribution among peripheral compartments or binding to plasma proteins. Medications that are hydrophilic and remain in the central (vascular) compartment, and without high affinity for plasma protein binding, tend to have a lower volume of distribution with a value that is closer to the intravascular volume. Drugs that are highly lipophilic and distribute to peripheral tissues, or are highly plasma protein bound, tend to have a very large volume of distribution. Clearance describes the volume of fluid cleared of drug over time. Clearance through an organ is determined by the product of blood flow to the organ and the extraction ratio for the organ. The extraction ratio is the percentage of medication removed from the blood as it passes through the eliminating organ: It depends not only on the blood flow rate but also on the free fraction of drug and the intrinsic ability of the organ to eliminate drug.
Changes in blood flow to the organ responsible for clearing the drug or any factor altering the extraction ratio of a drug can alter a drug’s clearance. For example, a patient experiencing septic or cardiogenic shock may have impaired blood flow to the liver or kidneys, hampering the clearance of a particular drug. In addition, if a pharmacologic vasopressor is added to the therapy, blood flow to the gastrointestinal tract may be compromised, resulting in a decreased absorption and transport of drug to the site of action.
Renal Drug Excretion
The primary organ of drug and drug metabolite clearance is the kidney. There are three major processes involved in renal drug clearance: glomerular filtration, tubular secretion, and tubular reabsorption. Both critical illness and renal dysfunction can alter any of these pathways individually or in combination. Studies evaluating the effect of renal impairment on drug elimination typically examine changes in total body clearance or serum concentration, since it is difficult to determine the specific impact on each pathway individually if multiple clearance routes are affected simultaneously.
Glomerular filtration is the most common pathway of renal medication excretion. The glomerular filtration rate (GFR) for an average healthy adult is between 100 and 125 mL per minute and represents approximately 20% of total plasma flow to the kidneys. Many physiologic factors affect glomerular filtration, including hydrostatic pressure and osmotic gradients. For drugs whose primary route of elimination is glomerular filtration, excretion occurs at a rate that is directly proportional to GFR (first-order process). The degree of plasma protein binding also affects filtration because only unbound drug is sufficiently small in size to be filtered across the glomerular capillaries. To estimate the possible impact of decreased filtration, it is important for the clinician to be aware of the fraction of renal drug elimination, in addition to the excretion method for any active or toxic metabolite. Tubular secretion refers to the active process of drug transport from the interstitial fluid surrounding the proximal tubule into the tubule’s lumen. The secretion rate depends on the intrinsic activity of the transporter, proximal tubule blood flow, and the percent of free or unbound drug. Tubular secretion can be an extremely efficient process with drug clearance rates exceeding filtration clearance [170]. Impaired renal function impacts tubular secretion because endogenous and exogenous organic acids and bases accumulate and compete for the transporters required for active secretion. It is difficult to predict if secretion will be increased or diminished, which may ultimately lead to drug toxicity or reduced efficacy [171]. Tubular reabsorption of drugs can be active or passive. Most of the ultrafiltrate passing through the nephron is reabsorbed. As the volume of fluid in the tubule decreases with this massive reabsorption, there can be a dramatic increase in drug concentration in the tubule, which promotes passive diffusion from inside the tubule into the plasma. Manipulation of urine pH can be used to decrease drug reabsorption and, therefore, increase excretion. Urine alkalization enhances the elimination of weak acids (e.g., barbiturates) by increasing the fraction of ionized drug.
Pharmacokinetic Changes in Critically ILL Patients with Renal Dysfunction
The pharmacokinetics of drugs used in critically ill patients can be altered as a function of the many dynamic physiologic changes that occur. Studies examining the pharmacokinetics of drugs used in the critically ill patient population are limited; most are performed in healthy volunteers or in relatively stable patients with a specific disease state. Patients with chronic kidney disease take multiple medications, and thus have an inherently increased risk of drug interactions, particularly in the context of altered pharmacokinetics associated with worsening renal dysfunction and critical illness. The next section of this chapter addresses some of the known pharmacokinetic changes and drug interactions that may occur in critically ill patients with renal impairment.
Absorption
Drug absorption in patients with renal dysfunction may be altered for many reasons. Gastrointestinal edema, nausea and vomiting due to uremia, and delayed gastric emptying all affect drug absorption in this patient population. In addition, patients may have comorbidities that contribute to changes in drug absorption, such as diabetic gastroparesis, diarrhea, and cardiovascular failure. Patients with chronic kidney disease and diabetic gastroparesis often are prescribed prokinetic agents (e.g., metoclopramide or erythromycin). The use of these agents may decrease enteral absorption of medications due to decreased gastric transit time, leading to decreased therapeutic effect or delayed onset of action [172]. Patients requiring phosphate-binding medications or antacids (aluminum or
calcium salts) are at risk for having these medications chelate or bind to other medications and decrease their absorption. To minimize chelation, certain medications administered enterally, such as ciprofloxacin, need to be spaced around the dosing of antacid/phosphate binders by at least 2 hours [173]. Changes in gastric pH from antacids or other acid-suppressing medications may impair the dissolution process of other enteral medications, leading to incomplete drug absorption. Bioavailability studies are lacking in critically ill patients, as most are conducted in healthy adults. In a majority of medications, however, the bioavailability in patients with impaired renal function is unchanged or increased [174].
calcium salts) are at risk for having these medications chelate or bind to other medications and decrease their absorption. To minimize chelation, certain medications administered enterally, such as ciprofloxacin, need to be spaced around the dosing of antacid/phosphate binders by at least 2 hours [173]. Changes in gastric pH from antacids or other acid-suppressing medications may impair the dissolution process of other enteral medications, leading to incomplete drug absorption. Bioavailability studies are lacking in critically ill patients, as most are conducted in healthy adults. In a majority of medications, however, the bioavailability in patients with impaired renal function is unchanged or increased [174].
Distribution
The distribution of drugs with high affinity for plasma protein binding can be significantly altered in critically ill patients with renal failure. Highly protein-bound drugs exist in a state of equilibrium between unbound (free) and bound drug (not free). Only the unbound drug is pharmacologically active. This means that if binding decreases, the amount of free drug available to exert a pharmacologic and toxic effect increases. Drug–drug interactions can occur when two highly plasma protein–bound drugs (> 90% bound to plasma proteins) compete for the same plasma protein. If drugs such as warfarin, phenytoin, valproic acid, and salicylates (all highly bound to albumin) are administered together, displacement-mediated drug interactions may occur [175]. Drug-binding interactions also occur in patients with poor renal function due to changes in the configuration of albumin [176,177]. For example, the pharmacodynamic effects of phenytoin and warfarin are increased in patients with renal failure due to changes in albumin.
Critically ill patients often have reduced albumin levels due to malnutrition or the metabolic stress of acute illness (or both), and this can lead to higher free fractions of drugs and potentially increase the risk of toxicity. If a patient taking warfarin rapidly develops hypoalbuminemia due to critical illness, the result is an increased availability of free drug, resulting in an elevated international normalized ratio and potential risk for bleeding.
The volume of distribution for drugs administered to critically ill patients with renal failure can fluctuate considerably as fluid status changes. This can affect the clearance of drugs, and also protein binding, by altering the amount of free drug available to be metabolized, eliminated, or both. Although it is very difficult, if not impossible, to predict these changes in drug distribution, it is important for the clinician to be aware of the risks and monitor for the signs of efficacy and toxicity so that the interactions are recognized and corrected.
Metabolism
The kidneys also actively metabolize medications, and impaired renal function can affect both renal and hepatic drug metabolism. Therefore, clinicians must potentially adjust drug dosages to account for diminished renal metabolism as well as decreased renal elimination [178,179]. Drugs that are oxidized by the cytochrome P450 2D6 isoenzyme are more likely affected than those metabolized by other isoenzymes [180]. The clinical significance of these effects in critically ill patients with renal disease remains to be determined and the true relevance is difficult to define, since critically ill patients often have impaired metabolic function from nonrenal causes, including hepatic damage, diminished hepatic blood flow (shock, elderly), and use of medications that act as enzyme inhibitors or inducers.
Elimination
Determining drug elimination in the critically ill patient population is challenging for many reasons. First, the majority of the studies to determine drug pharmacology and clearance are performed in critically ill patients undergoing anesthesia or in patients with chronic diseases limited to a single organ system. It is difficult to apply these data to a critically ill patient with unstable, multiple organ dysfunction. In addition, critically ill patients each have a unique combination of factors (i.e., liver failure, hemodynamic instability, malnutrition) that can affect renal drug clearance.
AKI is often accompanied by metabolic acidosis and respiratory alkalosis, which may affect the ionization of drug molecules and, therefore, affect tissue redistribution and clearance. A low serum albumin is often associated with AKI and can lead to an increase in filtration of free drug and increased clearance of drugs that are normally highly plasma protein bound.
Dysfunction of other organ systems can significantly alter renal drug clearance through various mechanisms. For example, low cardiac output from a cardiomyopathy or acute myocardial infarction or shunting of blood away from the kidney to the heart, brain, and muscle secondary to increased sympathetic nerve activity can lower renal perfusion. Both of these mechanisms decrease drug delivery to the glomeruli, thus reducing the clearance of drugs that are eliminated primarily by glomerular filtration. Retention of fluid may increase a drug’s volume of distribution and further reduce drug clearance. States of profound vasodilation, such as sepsis, systemic inflammatory response syndrome, pancreatitis, and liver failure, may impair renal drug elimination by decreasing GFR. Patients with mechanical ventilation may have reduced cardiac output (due to increased mean intrathoracic pressure), volume of distribution changes, and acid–base imbalance, which can affect renal drug disposition.
Assessing Renal Function
Assessment of kidney function in a critically ill patient is challenging but essential for appropriately dosing renally eliminated medications. There are many equations available to clinicians to estimate GFR. The Cockroft-Gault equation is the most commonly used in the clinical and research settings. The Cockroft-Gault equation generally overestimates the true GFR, thus appropriate clinical judgment should be exercised. The Modification of Diet in Renal Disease (MDRD) study equation is an alternative method and is the preferred equation for patients with chronic kidney disease [181]. Depending on the equation used to estimate GFR, discordance rates of between 12% and 36% of dose adjustment recommendations can be observed [182,183,184]. The clinician should be aware of the potential limitations of the currently available methods of GFR estimation and use clinical judgment to assess the level of renal function to use the medication dosage guidelines in Table 74.1 appropriately. A more detailed discussion regarding the assessment of renal function can be found in Chapter 73.
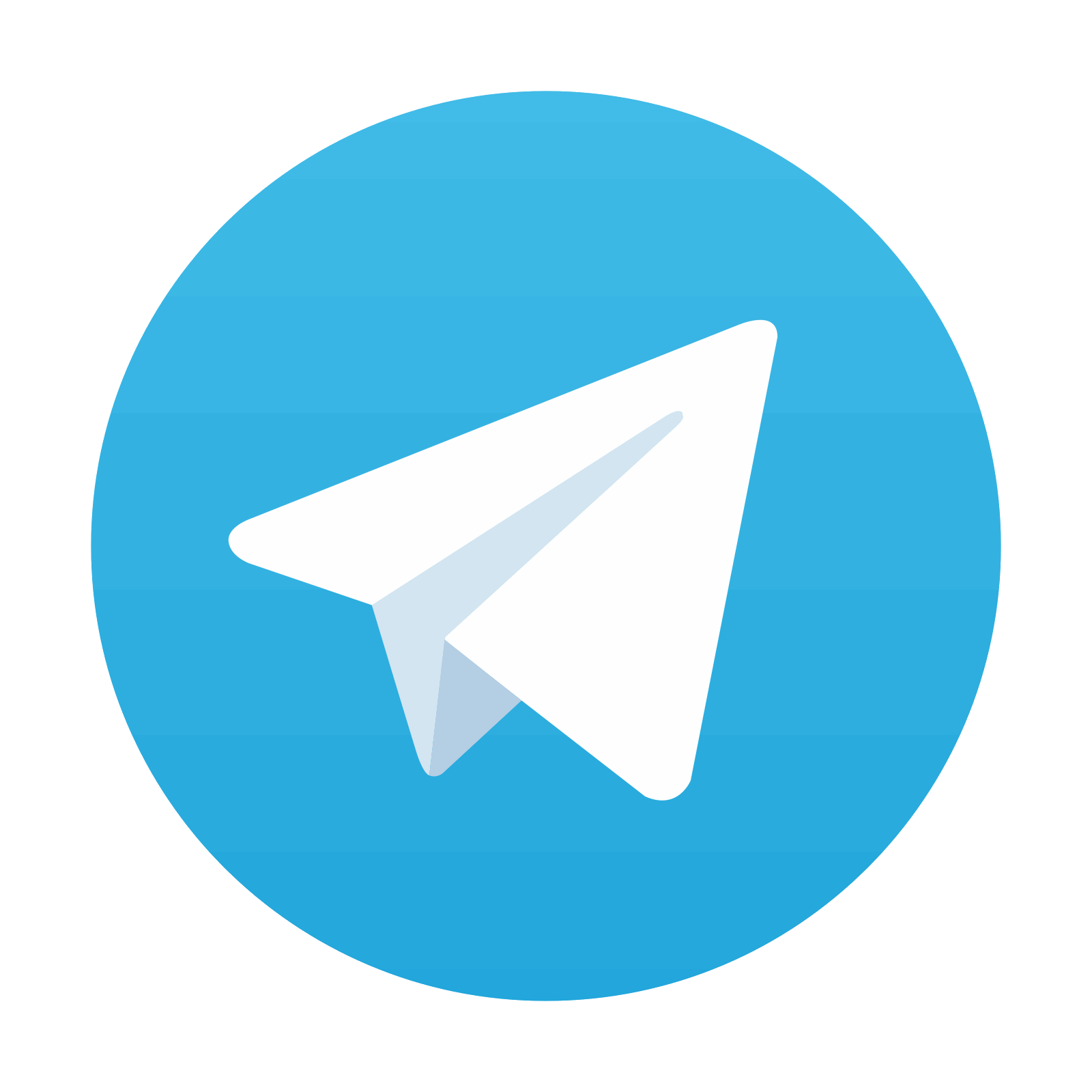
Stay updated, free articles. Join our Telegram channel

Full access? Get Clinical Tree
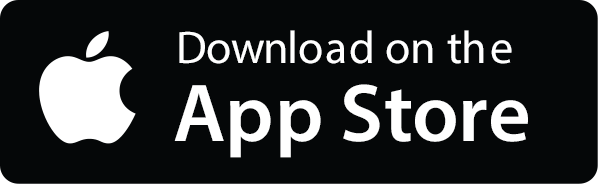
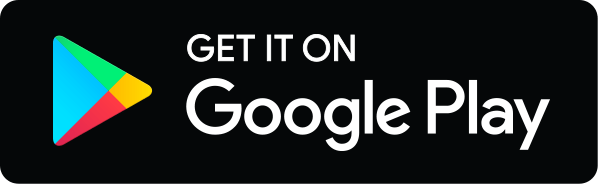