Key Clinical Questions
What is the difference between sodium and water balance and the regulation of each?
How is volume depletion assessed in patients with normal heart function and in those with underlying cardiac failure?
Which fluids are best for volume repletion?
How do diuretics work, and what are their side effects? How can resistance to their action be overcome?
How should hyponatremia and hypernatremia be assessed and safely corrected?
Introduction
Sodium and water disturbances are among the most commonly encountered disorders in hospitalized and critically ill patients. Sodium and water balance are independently regulated by mechanisms that are designed to maintain circulatory integrity and plasma osmolality, respectively. Sodium balance is regulated by changes in sodium intake and excretion, whereas plasma osmolality is regulated by changes in water intake and water excretion.
Water is the predominant constituent of the human body. In healthy individuals, it makes up 60% of a man’s body weight and 50% of a woman’s body weight. Body water is distributed between two compartments: the intracellular fluid compartment, containing 55% to 65%, and the extracellular fluid compartment, containing the remaining 35% to 45%. The extracellular fluid compartment is further subdivided into the interstitial space and the intravascular space. The interstitial space comprises approximately 75% of the extracellular fluid compartment, whereas the intravascular space contains 25%. Total body water diffuses freely between the intracellular space and the extracellular space in response to solute concentration gradients. Therefore, the amount of water in each compartment depends entirely on the quantity of solute in that compartment. The major extracellular solute is sodium, while potassium is the major intracellular solute. This solute distribution is maintained by active transport, via the Na+/K+-ATP-dependent pumps found on cell membranes.
Pathophysiology of Sodium Balance
The extracellular fluid compartment depends on the total body sodium content as well as the integrity of the mechanisms responsible for its maintenance. Sodium content is normally tightly regulated by modulating renal retention and excretion in situations of deficiency or excess extracellular fluid. The operative homeostatic mechanisms include an afferent sensing limb and an efferent effector limb. Disorders of either sensing or effector mechanisms can lead to failure to adjust renal sodium handling, resulting in hypertension and edema in positive sodium balance or hypotension and hypovolemia with negative sodium balance.
Effective arterial blood volume refers to the blood volume detected by baroreceptors in the arterial circulation. The effective arterial blood volume can change independently of the total extracellular fluid volume, leading to sodium and water retention in different clinical situations.
Afferent limb sensing sites include low-pressure cardiopulmonary receptors (atrial, ventricular, and pulmonary stretch receptors), high-pressure arterial baroreceptors (carotid, aortic arch, and renal sensors), and central nervous system and hepatic receptors. Activation of these afferent sites engages several effector mechanisms. Activation of the sympathetic nerves stimulates proximal tubular sodium reabsorption. Renin release from the renal juxtaglomerular apparatus stimulates the formation of angiotensin II, a potent vasoconstrictor. Angiotensin II also stimulates the adrenal gland to secrete aldosterone, which increases tubular sodium reabsorption. Other effector mechanisms include prostaglandins that modulate renal blood flow and sodium handling, natriuretic peptides that enhance renal sodium excretion in response to atrial stretching, and pituitary secretion of arginine vasopressin (AVP), a modest vasoconstrictor that also enhances renal water retention.
Osmoregulation is the regulation of water intake and excretion to maintain constant body osmolality (tonicity). Disorders of water homeostasis result in hypo- or hypernatremia, whereas disorders of total body sodium content result in extracellular volume depletion or overload. Since osmolality in all body fluids is essentially equal, it can be estimated by measuring the plasma osmolality. The normal plasma osmolality is 275 to 290 mOsm/kg. It is kept within this range by hypothalamic osmoreceptors, which are capable of sensing a 1% to 2% change in tonicity. Osmoreceptors influence thirst and the release of the antidiuretic hormone AVP. The combined effect of AVP stimulation and thirst results in water intake and retention, which lowers plasma osmolality and sodium concentration by dilution, whereas suppression of thirst and AVP secretion leads to the contrary.
Disorders of Sodium Balance
Extracellular fluid (ECF) volume contraction is caused by loss of sodium or water in excess of intake. These losses may be a result of renal or extrarenal losses or sequestration in potential spaces, such as the peritoneal cavity, retroperitoneum, pleural spaces, or muscles, which are not in hemodynamic equilibrium with the ECF (“third-spacing”).
The gastrointestinal tract is the most common extrarenal source of fluid loss. Vomiting or nasogastric suction may cause volume loss with metabolic alkalosis. Diarrhea may result in volume depletion with metabolic acidosis. Third-space sequestration, excessive sweat production, and loss of skin barrier from burns or exudative skin lesions may lead to significant ECF volume depletion. Hemorrhage, as from gastrointestinal bleeding or trauma, may lead to significant volume loss.
Diuretics may cause renal sodium wasting, volume contraction, and acid-base disturbances if abused or inappropriately prescribed. Genetic and acquired tubular disorders can result in renal sodium wasting and volume contraction. Mineralocorticoid deficiency and resistance often lead to renal sodium wasting. This may occur in the setting of primary adrenal insufficiency (Addison disease) or hyporeninemic hypoaldosteronism secondary to diabetes mellitus or other chronic interstitial renal diseases. Severe hyperglycemia or high levels of blood urea after relief of urinary tract obstruction can lead to obligatory renal sodium and water loss secondary to glucosuria or urea diuresis, respectively.
The symptoms of hypovolemia are nonspecific and can range from mild postural symptoms, thirst, muscle cramps, and weakness, to drowsiness and disturbed mentation with profound volume loss. Physical examination may reveal tachycardia, cold clammy skin, postural or recumbent hypotension, and reduced urine output depending on the degree of volume loss (Table 249-1). Rarely, diminished skin turgor may be seen. Reduced jugular venous pressure (JVP) noted at the base of the neck is a useful parameter of volume depletion and may roughly estimate the central venous pressure (CVP). However, an elevated CVP does not exclude hypovolemia in patients with underlying cardiac failure or pulmonary hypertension. The absence of symptoms or obvious physical findings does not preclude volume depletion in an appropriate clinical setting, and hemodynamic monitoring or administration of a fluid challenge may sometimes be necessary.
|
Laboratory findings consistent with volume depletion include hemoconcentration and increased serum albumin concentration. However, anemia or hypoalbuminemia may confound interpretation of these laboratory values. In healthy individuals, the ratio of blood urea nitrogen (BUN) (mg/dL) to serum creatinine (mg/dL) approximately equals 10. In volume depletion, this ratio increases because of a differential increase in the tubular reabsorption of urea. Several clinical conditions confound this ratio. Upper gastrointestinal hemorrhage and administration of corticosteroids increase urea production and the ratio of BUN to creatinine. Malnutrition and underlying liver disease diminish urea production, and thus the BUN-to-creatinine ratio is less useful in these clinical settings.
In the absence of renal salt wasting or diuretics, elevated urine osmolality and specific gravity may indicate hypovolemia. Hypovolemia normally triggers avid renal sodium reabsorption, resulting in low urine sodium concentration and low fractional excretion of sodium. The fractional excretion of sodium (FENa) is calculated using the following formula: FENa = [UNa × Pcreat/ Ucreat × PNa] × 100, where UNa and Ucreat are urinary sodium and creatinine concentrations, and PNa and Pcreat are plasma sodium and creatinine concentrations, respectively. Decreased FENa (less than 1%) is consistent with ECF volume depletion. However, arterial underfilling due to low cardiac output or systemic arterial vasodilatation, as in cirrhosis, is also associated with an FENa less than 1%, in spite of increased ECF and edema.
Volume depletion is treated by replacing fluid deficits and ongoing losses with a replacement fluid closely resembling the lost fluid. Clinical parameters help to distinguish mild to moderate from severe volume loss (Table 249-1), but invasive monitoring may be needed in some patients. Mild volume contraction can usually be corrected via the oral route. In hypovolemic shock with evidence of life-threatening circulatory collapse or organ dysfunction, intravenous fluid must be given as rapidly as possible until clinical parameters improve. A slow, more judicious approach is warranted in the elderly and those with underlying cardiac conditions in order to avoid overcorrection with subsequent pulmonary edema.
Crystalloid solutions are effective, as they distribute primarily in the ECF. Colloid-containing solutions, such as 5% and 25% albumin and hetastarch (6% hydroxyethyl starch), remain within the vascular compartment by virtue of their large molecular sizes. As studies have not shown an advantage for colloid-containing solutions in the treatment of hypovolemia, they are best reserved for hemodynamically unstable patients in whom rapid correction is needed, such as trauma and burn victims. Otherwise, isotonic saline is usually the initial replacement fluid of choice in volume-depleted patients.
Hypovolemic shock may be accompanied by lactic acidosis due to tissue hypoperfusion. Fluid resuscitation restores tissue oxygenation and decreases the production of lactate. Correction of acidosis with sodium bicarbonate has several potential adverse effects, including increasing tonicity, overexpanding ECF volume, and worsening intracellular acidosis. Therefore, its use to manage lactic acidosis in the setting of volume depletion is not recommended, unless the arterial pH is below 7.1.
Excess extracellular fluid accumulation usually results from sodium and water retention by the kidneys. Renal sodium retention may be primary or a compensatory response to reduced effective arterial blood volume. It manifests clinically with edema and weight gain, commonly in response to congestive heart failure (CHF), cirrhosis with ascites, or the nephrotic syndrome.
Renal sodium and water retention in CHF involves activation of the renin-angiotensin-aldosterone system (RAAS), as well as nonosmotic AVP stimulation, which drives the hyponatremia associated with CHF. Arterial underfilling is also responsible for water and sodium retention in cirrhosis. Unlike CHF and cirrhosis, in which the kidneys are structurally normal, the nephrotic syndrome is characterized by kidneys that are functionally impaired. Rarer causes of edema include drug-induced edema, which may be seen with ingestion of systemic vasodilators, such as minoxidil and diazoxide, dihydropyridine calcium channel blockers, and thiazolidinediones. Nonsteroidal anti-inflammatory drugs (NSAIDs) can exacerbate volume expansion in CHF and cirrhotic patients by decreasing vasoregulatory prostaglandins in the kidneys.
Patients with left heart failure may present with exertional dyspnea, orthopnea, and paroxysmal nocturnal dyspnea, whereas patients with predominant right heart failure or biventricular failure may exhibit weight gain and lower-limb swelling. Physical examination may reveal JVP elevation, pulmonary crackles, a third heart sound, or peripheral edema in the ankles or sacrum. Nephrotic patients classically present with periorbital edema because of their ability to lie flat during sleep. Patients with severe nephrotic syndrome may exhibit marked generalized edema with anasarca. Cirrhotic patients present with ascites and lower-limb edema consequent to portal hypertension and hypoalbuminemia. Physical examination may reveal stigmata of chronic liver disease, such as jaundice, spider angiomata, palmar erythema, Dupuytren contractures, and splenomegaly.
Management of ECF volume expansion consists of treating the underlying cause and achieving negative sodium balance with dietary sodium restriction and diuretics. Moderate dietary sodium restriction (2 to 3 g/day; 86 to 130 mmol/day) should be instituted. Restriction of total fluid intake is usually only necessary for hyponatremic patients. Medications that promote sodium retention, such as NSAIDs, corticosteroids, estrogens, and androgens, should be eliminated, if possible. Diuretics are the cornerstone of therapy to remove excess volume. Other measures can be used when the response to diuretics is inadequate. Extracorporeal fluid removal by ultrafiltration can be utilized in patients with acute decompensated heart failure with renal insufficiency or diuretic resistance. In cirrhosis, large-volume paracentesis with albumin infusion (8 grams per liter of ascitic fluid removed) can be employed to remove large volumes of ascitic fluid. Interventional maneuvers to shunt ascitic fluid to a central vein can also be considered in refractory ascites, and they may result in improvement of the GFR and sodium excretion.
Diuretics are the mainstay of therapy for edematous states. As ECF volume expansion in CHF and cirrhosis may be a compensatory mechanism for arterial underfilling, a judicious approach is necessary to avoid a precipitous fall in cardiac output and tissue perfusion. Diuretics can be classified into five classes, based on their predominant sites of actions along the nephron (Figure 249-1). They inhibit the reabsorption of sodium and an accompanying anion, usually chloride. The resultant natriuresis decreases the ECF. However, the time course of natriuresis is limited because renal mechanisms attenuate the sodium excretion.
The most serious adverse effects of diuretics are electrolyte and acid-base disturbances. Loop diuretics increase the excretion of potassium, magnesium, and calcium. Thiazide diuretics have similar effects on potassium and magnesium, but unlike loop diuretics, they decrease urinary calcium losses. Thiazide diuretics interfere with urine-diluting mechanisms, and may therefore pose a risk of hyponatremia. Patients on diuretic therapy require electrolyte monitoring, and may need oral supplementation of potassium and magnesium. Addition of a potassium-sparing diuretic may need to be considered in patients with severe or recurrent hypokalemia. The volume depletion caused by thiazides and loop diuretics leads to increased tubular urate reabsorption, promoting hyperuricemia and gout. Ototoxicity may occur with large intravenous doses of loop diuretics, particularly when an aminoglycoside is coadministered. Gynecomastia may develop with spironolactone therapy.
Long-term loop diuretic tolerance occurs as a consequence of hypertrophy of the distal nephron segment and enhanced sodium reabsorption from increased exposure to solutes not absorbed proximally. This can be addressed by combining loop and thiazide diuretics, as the latter blocks reabsorption distal to the loop of Henle.
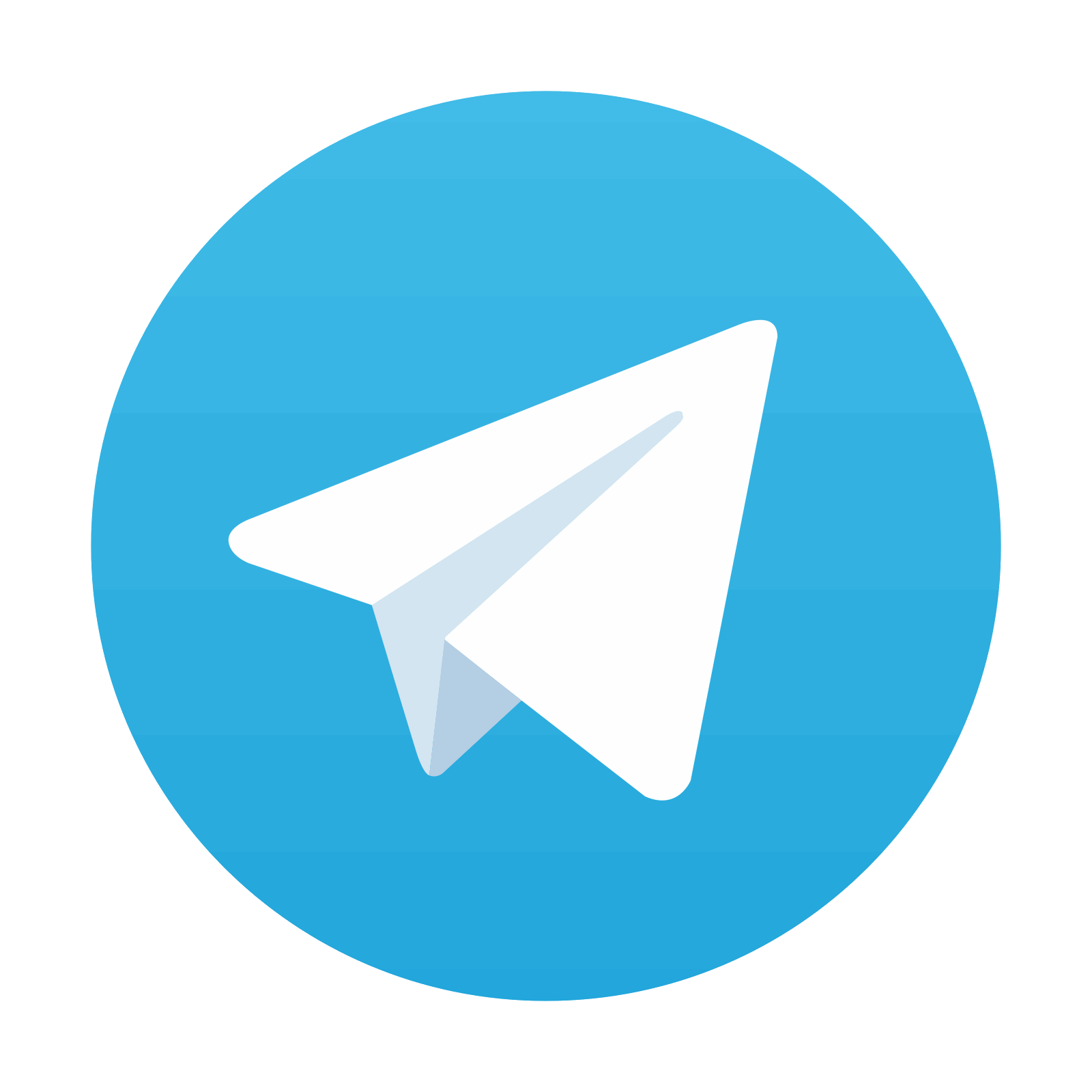
Stay updated, free articles. Join our Telegram channel

Full access? Get Clinical Tree
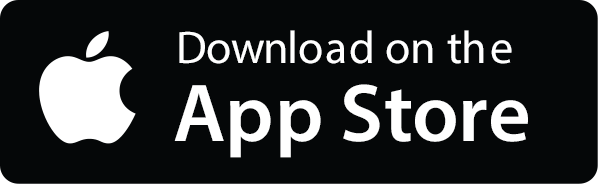
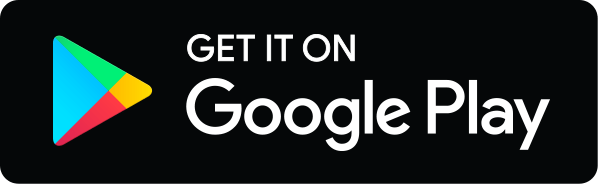