Disorders of Plasma Sodium and Plasma Potassium
Robert M. Black
Disorders of Plasma Sodium
Hyponatremia and hypernatremia are conditions commonly observed in the intensive care unit. They are defined as plasma Na+ concentration below 135 mEq per L and above 145 mEq per L, respectively. The correct management of patients with these disorders depends on an understanding of normal salt (NaCl) and water (H2O) physiology.
It is important to appreciate that hyponatremia represents a disorder of water balance; the plasma sodium concentration reflects the ratio of water to sodium in the body. By itself, however, the presence of hypo- or hypernatremia cannot be used to assess the volume status of a patient. Furthermore, the plasma sodium concentration has little relationship to the urinary sodium concentration.
Hypothalamic osmoreceptors influence thirst and the release of antidiuretic hormone (ADH). The latter increases UOsm, causing water retention by enhancing the permeability of the collecting tubules to water. ADH is also released in response to effective volume depletion (hypovolemia). Although water retention causes extracellular volume expansion, this is slight, as approximately two thirds of the water enters the cells. As a result, volume-mediated ADH release can occur even in states of hyponatremia (see following discussion).
Relationship Between Plasma Na+ and Plasma Osmolality
The osmolality of plasma (POsm) is determined by the sum of the osmolar contributions of the individual osmotically active substances. In plasma, Na+ salts, glucose, and urea (blood urea nitrogen [BUN]) are the major determinants of osmolality. Therefore, the POsm can be estimated by the following formula:

Using this equation,1 it is evident that the major determinant of the POsm in healthy individuals is the plasma Na+ concentration.
The ability of a solute (such as sodium) to promote shifts of water between the intracellular and extracellular compartments depends not only on its capacity to increase the POsm but also on its exclusion from one of these compartments. Because urea can cross almost all cell membranes readily, it cannot promote the movement of water between the intracellular and extracellular spaces. As such, urea is referred to as an ineffective osmole. A rise in the BUN is detected as an increase in the measured (by the laboratory) and calculated POsm, but there is no change in the plasma Na+ concentration because urea does not obligate water movement from the intracellular to the extracellular space. Because urea is an ineffective osmole and because glucose normally contributes less than 8 mOsm per kg, the effective POsm correlates best with the plasma Na+. Thus, effective POsm can be described as follows:

Sodium is confined primarily to the extracellular fluid by the Na-K antiporter present in most cells. This pump also maintains a high (approximately 130 mEq per L) intracellular K+ concentration; thus, potassium is the principal effective osmole inside cells. The ability of water to cross almost all cell membranes indicates that the POsm must be in equilibrium with the intracellular osmolality. In fact, osmolality is equal throughout all body compartments, explaining the need for only one osmoreceptor.
Because osmotic equilibrium exists throughout body water, calculation of water deficits or excesses, when dealing with a hyponatremic or hypernatremic individual, respectively, must be based on total body water (TBW) and not merely on the extracellular fluid volume. Moreover, loss of potassium from the body, as might occur with diuretic administration, affects the plasma Na+ concentration.
Two processes participate in the reduction in plasma Na+ concentration induced by potassium losses. Sodium movement into cells to maintain electroneutrality lowers the plasma Na+, and loss of potassium from the gastrointestinal tract or kidneys causes a fall in the plasma potassium with a larger fall in the intracellular potassium. The result is a reduction in the intracellular osmolality that leads to water movement from cells to the extracellular compartment.
Finally, although plasma hypoosmolality is always associated with hyponatremia, a high POsm can occur in the absence of hypernatremia. Other ineffective osmoles (in addition to urea) have the ability to raise the measured POsm without affecting water shifts. The most important of these are the alcohols: ethanol, ethylene glycol, and methanol. By contrast, severe hyperglycemia can induce hyponatremia by pulling water out of cells, but the POsm will be elevated. This phenomenon, seen most often in diabetics, is referred to as hyperosmolar hyponatremia.
Regulation of Plasma Osmolality
Maintenance of the plasma Na+ concentration within narrow limits (285 to 292 mOsm per kg) depends on the ability of the kidneys to excrete water (to prevent and on a normal thirst mechanism with access to water). Under normal conditions, the quantity of water that can be excreted in the urine far exceeds the amount ingested.
Renal water excretion is determined by two factors: urinary solute excretion and the ability to generate a maximally dilute
urine (UOsm < 100 mOsm per kg) [1]. The typical American diet affords a solute intake between 600 and 1,200 mOsm—average 900 mOsm—per day. Assuming an output that approximates intake, the daily urinary solute excretion of a typical adult would also average 900 mOsm. Dietary NaCl, KCl, and protein, which is broken down to urea, make up most of this solute load. The individual who excretes 900 mOsm of solute per day and who can dilute urine maximally (down to 50 mOsm per kg) has the capacity to excrete up to 18 of water in a 24-hour period:
urine (UOsm < 100 mOsm per kg) [1]. The typical American diet affords a solute intake between 600 and 1,200 mOsm—average 900 mOsm—per day. Assuming an output that approximates intake, the daily urinary solute excretion of a typical adult would also average 900 mOsm. Dietary NaCl, KCl, and protein, which is broken down to urea, make up most of this solute load. The individual who excretes 900 mOsm of solute per day and who can dilute urine maximally (down to 50 mOsm per kg) has the capacity to excrete up to 18 of water in a 24-hour period:

The capacity to dilute urine begins in the loop of Henle and continues to the collecting tubule. This portion of the nephron, which is impermeable to water, is often referred to as the diluting segment (Fig. 72.1). As filtrate passes through the loop of Henle, solute is removed by the Na-K-2Cl transporter located in the cells of thick ascending limb and by the NaCl carrier in the distal tubule. Filtrate leaving the diluting segment and entering the early collecting tubule characteristically is very dilute, with a urinary osmolality (UOsm) of less than 100 mOsm per kg.
A maximally dilute urine cannot be excreted if the removal of salt by the pumps and carriers in the diluting segment is impaired or if the collecting tubule is rendered permeable to water by the presence of ADH (see following discussion). Inability to dilute the urine may have serious consequences. For example, for a patient unable to achieve urinary dilution below an osmolality of 300 mOsm per kg, the amount of water that can be excreted on a normal diet is reduced to 3 L:

As discussed earlier, solute excretion is normally determined by dietary intake. A reduction in dietary sodium and protein intake, as is seen in the patient on a “tea-and-toast” diet, limits the capacity to excrete water. If solute intake falls to 150 mOsm per day, for instance, water excretion is limited to approximately 3 L even when urinary dilution is normal:

It is easy to see that the combination of impaired diluting ability with a concomitant reduction in solute intake is more likely to impair water excretion and result in hyponatremia than either disturbance alone.
Regulation of Antidiuretic Hormone
Healthy adults are able to excrete very large or very small volumes of urine, the concentration of which varies according to the POsm. The primary hormone regulating water excretion in health is ADH. ADH (called arginine vasopressin in humans) is synthesized in the supraoptic and paraventricular nuclei of the hypothalamus. Hypothalamic osmoreceptors for ADH release are stimulated by hyperosmolality and inhibited by hypoosmolality. A change in osmoreceptor cell volume is probably the factor that modifies ADH secretion in response to changes in the POsm. The concentration of urea, an ineffective osmole incapable of altering cell volume, neither promotes nor inhibits ADH release.
A 1% to 2% reduction in POsm (POsm < 280 mOsm per kg) maximally inhibits ADH release, leading to a UOsm that is less than 100 mOsm per kg [2]. By contrast, a 1% to 2% increase in POsm above normal, or a 7% to 10% decrease in blood pressure or volume (even in the presence of plasma hypoosmolality), stimulates ADH release [1]. In the presence of ADH, the luminal membranes of the cortical and medullary collecting tubules become permeable as water channels (aquaporins) are generated. This change permits water reabsorption, increasing the final urine osmolality.
Hyponatremia
In most settings, the development of hyponatremia with hypoosmolality represents the retention of ingested or administered water. Thus, the causes of hyponatremia can be divided into those in which water excretion is abnormal and those in which water excretion is normal but water ingestion is considerably increased.
Table 72.1 Causes of Hyponatremia | ||
---|---|---|
|
Hypoosmolar Disorders with Impaired Water Excretion
The UOsm is typically greater than 100 mOsm per kg in patients with reduced water excretion (Table 72.1). An exception to this rule occurs when solute intake is markedly reduced, as in the patient subsisting on a solute-poor diet.
Hypovolemic Hyponatremia
A fall in effective perfusion pressure stimulates release of ADH [3]. Hypovolemic hyponatremia can occur in states of volume depletion or in edematous individuals with congestive heart failure (CHF) or advanced liver disease because each of these conditions is associated with a reduced effective arterial blood volume. As discussed previously, the resulting increase in the UOsm (e.g., to 600 mOsm per kg) would limit renal water excretion on a 900 mOsm per day diet to 1.5 L, assuming all of the ingested solute were excreted (900 mOsm per kg 600). Solute excretion tends to be reduced in these settings, which are characterized by enhanced tubular salt reabsorption. In addition, most stimuli that activate angiotensin II release also stimulate thirst and lead to increased water ingestion, despite concurrent hypoosmolality.
Diuretic-Induced Hyponatremia
The ability to excrete a dilute urine is impaired by diuretics, whether they act in the thick ascending limb of Henle (loop diuretics) or in the distal tubule (thiazide-type diuretics) (Fig. 72.2). Each class reduces salt transport out of the diluting segment, thus raising the minimum achievable UOsm. Raising the minimum UOsm, however, does not usually lead to hyponatremia because a large volume of urine can still be excreted by most patients.
Almost all cases of diuretic-induced hyponatremia in otherwise healthy individuals have been caused by thiazide-type, rather than loop, diuretics [4]. This observation is attributable, in part, to different sites of the action within the renal tubule (Fig. 72.2). Loop diuretics, which act in the outer medulla, reduce the solute concentration in the renal medullary interstitium. Although ADH permits water reabsorption from the collecting tubule, medullary osmolality drives the process. A fall in the interstitial osmolality from 1,200 to 300 mOsm per kg, for instance, would limit the maximum UOsm that can be generated from 1,200 to 300 mOsm per kg. By comparison, thiazide diuretics, which act in the cortex, impair diluting capacity but have a lesser effect on concentrating ability. Thus, the UOsm may be 600 mOsm per kg in the thiazide-treated individual; if the urinary osmoles are primarily NaCl and KCl, urinary electrolyte losses can exceed those contained in an equal volume of plasma. In these patients, therefore, the plasma Na+ may actually fall in the absence of ongoing water intake. For reasons that are not well understood, however, most individuals with thiazide-induced hyponatremia gain weight, indicating that the hyponatremia is at least in part a result of increased water intake [4]. This disorder occurs more frequently in women, typically occurs early in therapy (within 1 to 4 weeks), and is more likely to be observed in elderly individuals [5]. As discussed previously, accompanying hypokalemia also may contribute to the fall in POsm.
Advanced Chronic Kidney Disease
The normal glomerular filtration rate (GFR) is approximately 180 L per day. As renal function decreases, the ability to excrete water also decreases. The limitation in water excretion occurs for two reasons: tubular dysfunction leads to an inability to dilute the urine maximally, even in the absence of ADH, and the drop in GFR, particularly when severe, reduces daily solute excretion.
Endocrine Deficiency
Hypothyroidism and hypocortisolism can impair water excretion. Both may reduce cardiac output or
stroke volume, leading to increased ADH release. The resulting fall in GFR adversely affects free water excretion by diminishing delivery of filtrate to the diluting segments. Decreased delivery may be particularly important in patients with myxedema in whom hyponatremia may develop despite appropriate suppression of ADH release [6].
stroke volume, leading to increased ADH release. The resulting fall in GFR adversely affects free water excretion by diminishing delivery of filtrate to the diluting segments. Decreased delivery may be particularly important in patients with myxedema in whom hyponatremia may develop despite appropriate suppression of ADH release [6].
Another factor contributing to the hyponatremia of hypocortisolism is that corticotropin-releasing factor promotes the corelease of adrenocorticotropic hormone (ACTH) and ADH, although the reason for the concomitant release of these hormones is not known [7]. It is important to note that adrenocortical dysfunction (as in Addison’s disease) leads to reduced cortisol and aldosterone levels, the latter predisposing to hyperkalemia. The presence of a low cortisol level alone, due to either pituitary or hypothalamic disease, or the abrupt withdrawal from prolonged exogenous corticosteroid administration may cause hyponatremia but should not alter potassium homeostasis, because aldosterone release is normal.
Syndrome of Inappropriate Antidiuretic Hormone Secretion
The syndrome of inappropriate ADH secretion (SIADH) is characterized by the following: plasma hypoosmolality; UOsm more than 100 to 150 mOsm per kg (because ADH should be absent in the hypoosmolar state); urinary Na+ concentration of more than 20 mEq per L, reflecting normal renal perfusion; normal adrenal, renal, and thyroid function; and normal potassium and acid–base balance. The UOsm does not need to exceed the POsm to make this diagnosis. SIADH may be caused by enhanced hypothalamic ADH secretion, ectopic hormone production (usually by cancer), or administration of medications with ADH activity (Table 72.2).
In approximately one third of patients, the SIADH is associated with resetting of the hypothalamic osmostat. This disorder has been described in patients with hypovolemia, psychosis, and chronic malnutrition and in normal pregnancy (in which the plasma Na+ concentration decreases by the second trimester from 140 to 135 mEq per L). ADH release is not suppressed until the POsm falls well below normal in this disorder. As a result, the POsm may vary between 240 and 250 mOsm per kg (plasma Na+ approximately 120 to 125 mEq per L), compared with the normal value of approximately 285 to 292 mOsm per kg. In contrast to the classic form of the SIADH, in which nonsuppressible ADH release is seen, ADH secretion ceases when the POsm falls below this new, reset level. Because suppression of ADH prevents a further fall in the plasma Na+ concentration, the severity of hyponatremia is limited in this condition.
An increasingly common cause of hyponatremia is symptomatic human immunodeficiency virus (HIV) infection [8]. Although hyponatremia in HIV-infected patients may result from volume deficiency or adrenal insufficiency, many patients have SIADH. Pneumonia due to Pneumocystis jiroveci or other organisms, central nervous system infections, and malignant disease are most often responsible in this setting.
Elevated ADH levels have been reported with the use of medications and various recreational drugs. The association of hyponatremia with the use of 3,4-methylenedioxymethamphetamine (Ecstasy) has been reported. The cause has been reported to be related to increased water drinking and the inappropriate presence of ADH [2]. Similar effects may be seen with the commonly prescribed selective serotonin reuptake inhibitors (SSRIs) used for the treatment of depression [9].
Cerebral Salt Wasting
Cerebral salt wasting is a rare disorder characterized by a low POsm, a UOsm above 100 to 150 mOsm per kg, and a urine Na+ concentration greater than 20 mEq per L [10]. Unlike SIADH, however, evidence of volume depletion (including low central filling pressures) is present. In affected individuals, therefore, the high urinary Na+ represents inappropriate salt wasting rather than a response to normal tissue perfusion (as in SIADH patients).
Table 72.2 Major Causes of the Syndrome of Inappropriate Antidiuretic Hormone (ADH) Secretion | ||||||||||||||||||||||
---|---|---|---|---|---|---|---|---|---|---|---|---|---|---|---|---|---|---|---|---|---|---|
|
The cause of this putative syndrome is unclear. It has been proposed that there may be increased release of natriuretic peptide from hormone-producing neurons in the brain that are activated by central nervous system dysfunction [11]. Both hypouricemia and renal tubular dysfunction have been reported. Mineralocorticoid replacement therapy with fludrocortisone acetate has been effective in some patients.
Reduced Solute Intake
As discussed previously, a reduction in salt and protein intake can lead to hypoosmolality if water intake exceeds output. Severely reduced solute intake, as occurs with a tea-and-toast diet, can cause hyponatremia even with normal degrees of water intake. “Beer drinkers’ hyponatremia” occurs for a similar reason; the limited amount of solute in beer relative to its water content may be inadequate to permit excretion of the ingested water. In both conditions, the UOsm should be maximally dilute (UOsm < 100 mOsm per kg). The absence of polyuria and the development of hyponatremia with normal or slightly above normal fluid intake distinguish these
individuals from those with primary polydipsia (see following discussion).
individuals from those with primary polydipsia (see following discussion).
Hypoosmolar Disorders with Normal Water Excretion
Psychiatric patients, particularly those with schizophrenia, often have abnormalities in water balance. Evaluation of psychotic patients has revealed that a variety of defects in water handling can occur that affect thirst, the release of ADH, and the renal response to ADH. Depending on the abnormality that is present, the patient may present with polydipsia and polyuria or hyponatremia.
Hyponatremia has been reported in as many as 13% of marathon runners and may occasionally be fatal. Although the exact mechanism has not been elucidated, risk factors for developing low serum sodium levels include weight gain during the race, female sex, racing time, and lower body mass index [12].
Primary Polydipsia
These individuals may have psychiatric disorders or may intentionally drink large volumes of water for social (dietary) or health reasons. This may be manifested clinically by exaggerated weight gain during the day associated with a transient reduction in the plasma sodium concentration [13].
In some individuals, a central defect in thirst regulation plays an important role in the pathogenesis of polydipsia. For example, the osmotic threshold for thirst may be reduced below the threshold for the release of ADH [14]. These patients continue to drink until the POsm is less than the threshold level. This implies truly prodigious water intake, as ADH secretion is suppressed by the fall in POsm, resulting in rapid excretion of the excess water and continued stimulation of thirst. The osmotic regulation of thirst differs from that of healthy subjects, in whom the thirst threshold is roughly equal to or a few milliosmoles per kilogram higher than the threshold for ADH [15]. The mechanism responsible for abnormal thirst regulation in this setting is unclear [16]. Drug therapy may contribute to the increase in water intake if the medication induces the sensation of a dry mouth.
Because people with normally functioning kidneys and regulation of ADH secretion are capable of excreting more than 10 to 15 L of urine per day, hyponatremia due to polydipsia is unusual. Despite this, there are rare patients in whom severe, and potentially fatal, hyponatremia has developed even though the UOsm was appropriately dilute. More commonly, however, polydipsic patients manifesting hyponatremia have a concurrent abnormality in ADH release or response [17]. Concurrent thiazide diuretic therapy for systemic hypertension can lead to a marked and symptomatic reduction in the plasma sodium concentration in these patients.
There is no proven specific therapy for primary polydipsia with or without hyponatremia in psychotic patients. Limiting water intake rapidly raises the plasma sodium concentration because the excess water is readily excreted in a dilute urine. The risk of inducing osmotic demyelination (see later) in this setting is unclear; it has been suggested that patients with primary polydipsia and repeated episodes of acute hyponatremia are generally resistant to neurologic injury induced by rapid correction (see following discussion).
Over time, limiting the use of drugs that cause dry mouth, restricting fluid intake, and frequent weighing (to detect water retention) may be helpful. Antagonists to ADH are not likely to be useful if the urine is already maximally dilute but may help in patients with primary polydipsia and concomitant SIADH.
Hyponatremia Without Hypoosmolality
Hyponatremia may occur without plasma hypoosmolality. An increase in the plasma concentration of proteins (as immunoglobulins in multiple myeloma) or lipids (primarily triglycerides in lipemic plasma) can reduce the plasma Na+ concentration. Lipids and proteins displace water from a given volume of plasma but do not affect the Na+ concentration in the water phase of plasma [18]. As a result, the measured POsm is normal in this condition, which is called pseudohyponatremia. Because the sodium concentration in the aqueous component of plasma is normal, this form of hyponatremia is not of pathophysiologic consequence. The methods of electrolyte determination currently used in many laboratories (ion-selective electrodes) are not affected by plasma lipids or proteins.
An unusual form of hyponatremia, sometimes associated with a normal POsm but occasionally with hypoosmolality, can be observed after lithotripsy, uterine irrigation after endometrial ablation, or with transurethral prostatectomy [19,20,21], which often requires the use of as much as 20 to 30 L of nonconductive flushing solutions containing glycine, sorbitol, or mannitol. Some patients absorb 3 L or more of fluid through the exposed mucosal vascular plexus, leading to a dilutional reduction in the plasma sodium concentration that may fall below 100 mEq per L. In one prospective study of 100 patients, the incidence of hyponatremia after transurethral prostatectomy was 7%; there was one death [22]. Even when POsm is not notably reduced, confusion, disorientation, twitching, seizures, and hypotension may occur. Several methods have been devised to attempt to monitor the amount of fluid absorbed so that patients at risk for severe hyponatremia can be detected. Frequent determinations of the plasma sodium concentration are important.
There are instances in which patients with plasma hyperosmolality may develop hyponatremia (hyperosmolar hyponatremia). This most commonly occurs with severe hyperglycemia or when mannitol is given to patients with renal failure, resulting in an osmotic shift of water from cells into the extracellular fluid, diluting the plasma Na+ concentration. In contrast to hypoosmolar hyponatremia, treatment is directed at correcting the high glucose concentration with insulin and free water repletion because cellular dehydration is present. For every 100 mg per dL rise in the blood sugar, the plasma Na+ concentration falls by approximately 1.6 mEq per L [23], although this estimate varies with body size, falling more in a smaller individual.
Symptoms of Hypoosmolality
The neurologic manifestations of hyponatremia appear to be entirely due to the consequences of plasma hypoosmolality. A fall in POsm causes water movement from the extracellular space into cells. The resulting increase in cell water, which is of particular importance in the central nervous system, can lead to brain swelling. A variety of symptoms may be found, including lethargy, confusion, nausea, vomiting, and, in severe cases, seizures and coma. Focal neurologic symptoms are uncommon. Hyponatremic encephalopathy is generally reversible, although permanent neurologic damage or death has been reported, chiefly in premenopausal women [24]. Hyponatremic women may progress rapidly from minimal symptoms (such as headache and nausea) to respiratory arrest. Cerebral edema and herniation have been found in those women who died, suggesting a possible hormonally mediated decrease in the efficiency of the osmotic adaptation (see following discussion). The reason for the higher morbidity in this patient population is not well understood.
The likelihood that symptoms will develop is related to the level of hyponatremia and the rapidity with which it develops [25]. For example, a rapid decline in the plasma Na+ concentration during several hours or days (e.g., from 140 to 115 mEq per L) may be associated with severe neurologic findings.
In comparison, a similar fall in plasma sodium occurring during 1 week or more may not cause any symptoms. In the latter circumstance, the degree of cerebral edema is much less [26]. This protective response, which begins on the first day and is complete within several days, occurs in two major steps:
In comparison, a similar fall in plasma sodium occurring during 1 week or more may not cause any symptoms. In the latter circumstance, the degree of cerebral edema is much less [26]. This protective response, which begins on the first day and is complete within several days, occurs in two major steps:
The initial cerebral edema elevates the interstitial hydraulic pressure, creating a gradient for extracellular fluid movement out of the brain into the cerebrospinal fluid.
The brain cells lose solutes, leading to the osmotic movement of water out of the cells and less brain swelling [27]. The volume regulatory response begins with the movement of potassium and sodium salts out of the cells, followed by organic solutes, particularly the amino acids glutamine, glutamate, and taurine, and, to a lesser degree, the carbohydrate inositol. Electrolyte movement occurs quickly because it is mediated by the activation of quiescent cation channels in the cell membrane; organic solute loss occurs later because it requires synthesis of new transporters.
The organic solutes (called osmolytes) account for approximately one third of the cellular solute loss in chronic hyponatremia. Changes in the concentration of these solutes offer the advantage of restoring cell volume without interfering with protein function; in comparison, a potentially deleterious effect on protein function would occur if the volume adaptation were mediated entirely by changes in the cell cation (potassium plus sodium) concentration.
This adaptation is so efficient that it is not uncommon to see patients with heart failure or SIADH who are asymptomatic despite a plasma sodium concentration of 115 to 120 mEq per L. The occurrence of symptoms in patients with chronic hyponatremia usually signifies a profoundly low serum sodium concentration, less than 110 to 115 mEq per L.
Diagnosis of Hyponatremia
Three laboratory findings provide important information in the differential diagnosis of hyponatremia: POsm, UOsm, and urinary sodium concentration.
Plasma Osmolality
Because POsm is mainly determined by the plasma sodium concentration, it is reduced in most hyponatremic patients. In some cases, however, the POsm is either normal (as in pseudohyponatremia) or elevated (as in hyperosmolar hyponatremia) [18].
Urine Osmolality
In patients with hypoosmolar hyponatremia, the UOsm can be used to distinguish between patients with impaired water excretion, accounting for most cases, and primary polydipsia, in which water excretion is normal but intake is so high that it exceeds excretory capacity. Hyponatremia caused by primary polydipsia should completely suppress ADH secretion, resulting in the excretion of a maximally dilute urine with an osmolality less than 100 mOsm per kg and a specific gravity less than 1.003. A higher UOsm indicates an inability to excrete free water normally, which suggests continued secretion of ADH.
Urinary Sodium Concentration
The two major causes of hyponatremia are hypovolemia and SIADH. These disorders can usually be distinguished by measuring the urinary sodium concentration. The urinary sodium concentration of patients with hypovolemia is typically less than 20 mEq per L, assuming the patient is not receiving diuretics. Because patients with SIADH have normal renal perfusion, unless they are on a very low sodium intake, the urinary sodium concentration is greater than 40 mEq per L. Patients with the SIADH can conserve urinary sodium normally and raise the urine osmolality further if intravascular volume depletion occurs.
Evaluation of acid–base and potassium balance may aid in the diagnosis in some hyponatremic patients. As examples, metabolic alkalosis and hypokalemia suggest diuretic use or vomiting, metabolic acidosis and hypokalemia suggest diarrhea or laxative abuse, and metabolic acidosis and hyperkalemia suggest adrenal insufficiency. On the contrary, plasma bicarbonate and potassium concentrations are typically normal in patients with SIADH. Although water retention tends to lower these values by dilution, as it does the plasma sodium and chloride concentrations, normal levels are restored by the factors that normally regulate acid–base and potassium balance.
The initial water retention and volume expansion in patients with SIADH are typically associated with hypouricemia (plasma uric acid concentration of ≤3 mg per dL) due to increased uric acid excretion in urine [28]. Also, urinary urea losses may cause a fall in the BUN to less than 5 mg per dL. These findings are the opposite of what is typically seen in volume depletion and thiazide-induced hyponatremia.
All of the findings seen in SIADH also have been described in the controversial syndrome of cerebral salt wasting, a disorder in which the high urinary sodium concentration occurs as a result of defective tubular reabsorption, and the elevation in ADH and subsequent development of hyponatremia are due to the associated volume depletion. Hypouricemia also may be present, which presumably is another manifestation of impaired renal tubular function (see previous discussion).
Fractional Excretion of Sodium
The fractional excretion of sodium (FENa) is a more accurate assessment of volume status than the urinary sodium concentration in patients with acute kidney injury; an FENa less than 1% suggests effective volume depletion. This observation has led many physicians to use the FENa in any situation in which the urinary sodium concentration might be helpful. However, the FENa may be misleading in patients with relatively normal renal function because the expected value to differentiate volume depletion from euvolemia varies with the GFR. A value of less than 1% is not the correct dividing line in this setting because this value may occur in euvolemic patients (such as those with SIADH) who have a urinary sodium concentration above 50 mEq per L and who excrete more than 100 mEq of sodium per day. As a result, the random urinary sodium concentration is more accurate for assessing the volume status in patients with hyponatremia with a normal plasma creatinine concentration. The FENa should generally not be used in this setting.
Treatment of Hyponatremia
Saline or Water Restriction
In general, the plasma sodium concentration can be raised by giving patients salt (either as saline or salt tablets) or by restricting their water intake to below the level of excretion. The choice of therapy is primarily governed by the cause of the hyponatremia. The more recent additions of antagonists to the ADH receptor in the collecting tubule (vaptans) will also be discussed.
Salt administration, usually as isotonic saline, is appropriate in those with true volume depletion; diuretic therapy, provided the diuretic is no longer acting; or adrenal insufficiency, in which cortisol replacement is also indicated. Water restriction is used in patients without neurologic symptoms who have
edematous states such as heart failure and hepatic cirrhosis and in those with SIADH, primary polydipsia, or advanced chronic kidney disease.
edematous states such as heart failure and hepatic cirrhosis and in those with SIADH, primary polydipsia, or advanced chronic kidney disease.
In a state of true volume depletion, isotonic saline corrects the hyponatremia by two mechanisms. Each liter of saline infused raises the plasma sodium by 1 to 2 mEq per L because saline has a higher sodium concentration (154 mEq per L) than plasma. By eventually causing volume repletion, it also removes the stimulus to ADH release, thereby allowing the water surfeit to be excreted. At this time, the plasma sodium concentration may return rapidly toward normal.
Isotonic saline should be considered in patients with mild-to-moderate or asymptomatic hyponatremia. In contrast, symptomatic patients or those with a plasma sodium less than 115 mEq per L usually require initial therapy with hypertonic saline. It must be emphasized, however, that careful monitoring is essential because overly rapid correction carries the risk of inducing iatrogenic neurologic complications (see following discussion) [3].
In primary polydipsia, the initiation of water restriction may result in a dramatic rise in the plasma sodium concentration. There is, however, some evidence suggesting that these patients may be less predisposed to osmotic demyelination because their hyponatremia often is of rapid onset, with less brain cell adaptation apt to occur [29].
The optimal rate of correction of hyponatremia varies with the clinical state of the patient. The following represents a reasonable approach, given the information currently available [3,30]. In asymptomatic patients, who are more likely to have chronic hyponatremia, the plasma sodium concentration should be raised at a maximum rate of approximately 0.5 mEq per L per hour and less than 12 mEq per L per day. A more rapid elevation can increase the risk of osmotic demyelination.
More rapid initial correction is indicated in patients with symptomatic hyponatremia, particularly those presenting with seizures or other severe neurologic manifestations, which primarily result from cerebral edema induced by acute (developing during 2 to 3 days) hyponatremia [30]. Here, the plasma sodium concentration can be raised at an initial rate of 1.5 to 2.0 mEq per L per hour for the first 3 to 4 hours (or longer, if the patient remains symptomatic) because the risk of persistent severe hyponatremia outweighs that of overly rapid correction. This appears to be particularly important in premenopausal women, who may progress from minimal symptoms (headache and nausea) to coma and respiratory arrest; furthermore, irreversible neurologic damage or death is relatively common in younger women with symptomatic hyponatremia, even if the hyponatremia is corrected at an appropriate rate. In comparison, men are at much less risk of symptomatic hyponatremia and of permanent neurologic injury. After the initial 3 to 4 hours of rapid correction, the rate should be slowed down so that the total rise in plasma sodium does not exceed approximately 12 mEq during the initial 24 hours.
The quantity of sodium required to achieve the desired elevation in the plasma sodium concentration in patients with true volume depletion can be estimated from the product of the plasma sodium deficit per liter and the TBW, which represents the osmotic space of distribution of the plasma sodium concentration. Normal values for the TBW are 0.5 and 0.6 times the lean body weight in women and men, respectively. If the initial aim in an asymptomatic hyponatremic 60-kg woman is to raise the plasma sodium concentration from 110 to 120 mEq per L, then

Thus, 600 mL of 3% hypertonic saline (which contains roughly 1 mEq of sodium per 2 mL, 500 mEq Na, and 500 mEq Cl per L) should be given during 20 hours at a rate of 30 mL per hour. This regimen should raise the plasma sodium concentration at the desired rate of 0.5 mEq per L per hour, and serial monitoring of the plasma sodium concentration (beginning at 2 to 3 hours) is still required.
The preceding formula may be less accurate in patients with SIADH [3]. In this setting, the administered salt in the hypertonic 3% saline is excreted because plasma volume expansion is present. Therefore, the rise in plasma sodium is not due to sodium retention. Because there are approximately 1,000 mEq of solute (Na and Cl) in a liter of 3% saline, the fluid in the renal collecting tubule is relatively hyperosmotic. This causes water to be retained in the urine and excreted simultaneously with the salt load. If, for example, the UOsm were 500 mOsm per kg, the 1,000 mEq of salt excreted would obligate the elimination of 2 L of urine (a net loss of 1 L of free water, because 1 L of water was administered with the hypertonic saline). The result would be no change in total body sodium; the plasma sodium concentration would increase because of the loss of 1 L of water. Administration of the same hypertonic saline solution to an individual with SIADH and a UOsm of 250 mOsm per kg would result in the loss of 4 L of urine (a net water loss of 3 L). The serum sodium would increase by a greater amount than it did in the person with the higher UOsm. As a consequence of the excretion of the salt load and varying levels of UOsm in patients with SIADH, the sodium replacement formula is frequently misleading in this setting.
Effect of Potassium
Potassium is as osmotically active as sodium, and giving potassium can raise the plasma sodium concentration and osmolality in a hyponatremic subject. As most of the excess potassium goes into the cells, electroneutrality is maintained in one of three ways, each of which raises the plasma sodium concentration: (a) intracellular sodium moves into the extracellular fluid; (b) extracellular chloride moves into the cells with potassium; the increase in cell osmolality promotes free water entry into the cells; and (c) intracellular hydrogen moves into the extracellular fluid. These hydrogen ions are buffered by extracellular bicarbonate and, to a much lesser degree, by plasma proteins. This buffering renders the hydrogen ions osmotically inactive; the ensuing fall in extracellular osmolality leads to water movement into the cells.
Thus, any administration of potassium must be taken into account when calculating the sodium deficit. This relationship becomes clinically important in the patient with severe diuretic or vomiting-induced hyponatremia who is also hypokalemic.
Risk of Osmotic Demyelination
Severe hyponatremia, especially if acute in onset, can lead to cerebral edema, potentially irreversible neurologic damage, and death [24]. This most often occurs when large volumes of hypotonic fluids are given to postoperative patients who have pain-induced ADH release that impairs the ability of the kidneys to excrete water or to patients with acute thiazide-induced hyponatremia. Within 24 hours, however, the brain begins to lose extracellular water into the cerebrospinal fluid and loses intracellular water by extruding sodium and potassium salts and osmolytes, thereby lowering the brain volume toward normal (see previous discussion).
The effect is that hyponatremia that develops slowly (i.e., during > 2 to 3 days) is associated with a lesser likelihood of neurologic symptoms. In this setting, in which brain volume has fallen toward normal, rapid correction of severe hyponatremia may lead within 1 to several days to the development of a neurologic disorder called osmotic demyelination or central pontine myelinolysis. These lesions are detectable by cerebral computed tomography or magnetic resonance imaging. Results
of these diagnostic tests may not become positive for as long as 4 weeks, however [31].
of these diagnostic tests may not become positive for as long as 4 weeks, however [31].
It has been suggested that there are differences in individual susceptibility to osmotic demyelination. For example, observations indicate that psychiatric patients with primary polydipsia are relatively resistant to osmotic demyelination despite having repeated episodes of hyponatremia and, due to normal water excretory capacity, rapid correction of the plasma sodium concentration [29]. The reasons for this are not known, but this resistance to demyelination may not characterize the polydipsia of chronic alcoholics [32].
The mechanisms responsible for osmotic demyelination are not completely understood. Rapid elevation in the plasma sodium concentration leads to water movement out of the brain, which can lower the brain volume below normal. Such osmotically induced shrinkage in axons could sever their connections with surrounding myelin sheaths. Alternatively, the initial brain cell response to brain shrinkage may be the uptake of potassium and sodium from the extracellular fluid; this elevation in cell cation concentration could be toxic to the cells.
The manifestations of osmotic demyelination, which may be irreversible, include mental status changes, dysarthria, dysphagia, paraparesis or quadriparesis, and coma; seizures may occur but are less common. Patients in whom the plasma sodium concentration is raised to more than 20 mEq per L in the first 24 hours or is overcorrected to greater than 140 mEq per L are at greatest risk. Other putative risk factors for osmotic demyelination include chronic alcoholism, malnutrition, prolonged diuretic use, liver failure and transplantation, and burns [33]. On the contrary, late neurologic deterioration is rare if the hyponatremia is corrected at an average rate equal to or less than 0.5 mEq per L per hour [3,30].
Studies in experimental animals indicate that the total rate of correction during the first 24 hours is more important than the maximum rate in any given hour [34]. Demyelinating lesions are most common when the plasma sodium concentration in severe hyponatremia is raised to more than 20 mEq per L per day and are rare at a rate less than 10 to 12 mEq per L per day. This is similar to the safe average rate of correction of 0.5 mEq per L per hour observed in humans.
Recommendations
The preferred rate at which the plasma sodium concentration should be elevated varies with the clinical presentation. Due to the cerebral adaptation previously described, patients with chronic asymptomatic hyponatremia are generally at little risk for neurologic symptoms. In this setting, rapid correction is not indicated and may be harmful. Although the optimal rate of correction is not clearly proven, the current recommendation in asymptomatic patients is that the plasma sodium concentration be raised at a maximum rate of 12 mEq per L per day (which represents an average correction of 0.5 mEq per L per hour). Although it may be safe to increase the plasma sodium concentration at a rate of more than 12 mEq per day, there is no reason to correct it more rapidly in the absence of symptoms.
It is not known whether there is a potential benefit to administering water to previously hypoosmolar patients whose hyponatremia has been corrected much too rapidly. In rodents, a marked reduction in the incidence and severity of brain lesions was demonstrated if overly rapid correction (30 mEq per L or more during several hours) was partially reversed so that the net daily elevation in the plasma sodium concentration was less than 20 mEq per L [35]. This improvement was seen if therapy was begun before the onset of neurologic symptoms; benefit was much less likely in animals with symptomatic demyelination. The applicability of these findings to humans is uncertain.
More aggressive initial correction, at a rate of 1.5 to 2.0 mEq per L per hour, is indicated for the first 3 to 4 hours (or until the symptoms resolve) in patients who present with seizures or other severe neurologic abnormalities due to untreated and usually acute hyponatremia. The primary problem in these patients is cerebral edema, and the risk of delayed therapy is greater than the potential risk of too rapid correction. Even in this setting, however, the plasma sodium concentration should probably not be raised by more than 12 mEq per L in the first 24 hours because partial cerebral adaptation has already occurred. It is usually not necessary to continue hypertonic saline once the plasma sodium concentration is greater than 120 mEq per L.
Treatment of Hyponatremia in the Syndrome of Inappropriate Antidiuretic Hormone Secretion
Hyponatremia in SIADH results primarily from ADH-induced retention of ingested water. Appropriate therapy in this disorder depends on the severity of the hyponatremia and on the fact that, although water excretion is impaired, sodium handling is intact because there is no abnormality in volume-regulating mechanisms such as the renin–angiotensin–aldosterone system. Water restriction is the mainstay of therapy in asymptomatic hyponatremia of chronic SIADH. The associated negative water balance raises the plasma sodium concentration toward normal.
Severe, symptomatic, or resistant hyponatremia often requires the administration of salt. If the plasma sodium concentration is to be elevated, the osmolality of the fluid given must exceed that of the urine. This can be illustrated by a simple example (Table 72.3). Suppose a patient with SIADH and hyponatremia has a UOsm that cannot be reduced below 616 mOsm per kg. If 1,000 mL of isotonic saline is given (containing 154 mEq each of Na and Cl or 308 mOsm), all of the salt is excreted (because sodium handling is intact), but in only 500 mL of water (308 mOsm in 500 mL of water equals 616 mOsm per kg). The retention of half of the administered water leads to a further reduction in the plasma sodium concentration. As a result, correction of the hyponatremia in these cases requires the administration of hypertonic 3% saline intravenously or salt tablets orally, preferably in combination with a drug that lowers the UOsm and increases water excretion by impairing the renal responsiveness to ADH. A loop diuretic is most often used for this purpose.
Demeclocycline and lithium act on the collecting tubule cell to diminish its responsiveness to ADH, thereby increasing water excretion. These drugs tend to be too toxic or ineffective in most patients. Specific antagonists to the ADH-V2 (antidiuretic) receptor in the cortical collecting tubule have shown promise and two have now been approved for clinical use. Two of these have been approved by the Food and Drug Administration (FDA) at the time of this writing.
Table 72.3 Mechanism of Normal Saline-Induced Worsening of Hyponatremia in the Syndrome of Inappropriate Antidiuretic Hormone Secretiona | |||||||||||||||
---|---|---|---|---|---|---|---|---|---|---|---|---|---|---|---|
|
Conivaptan, an antagonist to both the V1 (vascular) and V2 (ADH) receptors, was the first. It must be given intravenously in the hospital and has the propensity to cause phlebitis. Recent data suggest that a single 20 mg bolus intravenously over 30 minutes results in a sustained water diuresis and may avoid vascular injury. It is not suitable for chronic use. Tolvaptan is an oral ADH antagonist which is specific for the V2 receptor. It must also be initiated in the hospital but can be administered in the outpatient setting after that. Tolvaptan has been shown to decrease body weight in chronic heart failure patients in a single controlled study [36].
The choice of initial therapy in symptomatic patients is usually 3% saline and this is the preferred treatment with severe neuropathology (such as seizures). In less symptomatic individuals, a vaptan may be effective. However, as there are no controlled prospective studies comparing hypertonic saline with the vaptans, it is difficult to recommend the latter in the SIADH as first-line agents, particularly when cost is considered. When they are administered, it is important to relax fluid restriction during the initial titration with these agents to avoid an excessive rise in the serum sodium concentration.
Reset Osmostat
Hyponatremia due to a reset osmostat may occur in association with any of the causes of SIADH and accounts for between 25% and 30% of cases overall. Downward resetting of the osmostat can also occur in hypovolemic states in which the baroreceptor stimulus to ADH release is superimposed on osmoreceptor function; quadriplegia, in which effective volume depletion may result from venous pooling in the legs; psychosis; tuberculosis; and chronic malnutrition. The plasma sodium concentration also falls by approximately 5 mEq per L in normal pregnancy. How this occurs is incompletely understood, but human chorionic gonadotropin may play an important role [37].
The presence of a reset osmostat should be suspected in any patient with apparent SIADH who has mild hyponatremia (usually between 125 and 135 mEq per L) that is stable over many days despite variations in sodium and water intake. The diagnosis can be confirmed clinically by observing the response to a water load (10 to 15 mL per kg given orally or intravenously during 30 minutes). Healthy subjects and those with a reset osmostat should excrete more than 80% within 4 hours, whereas excretion is impaired in classic SIADH.
Identification of a reset osmostat is important because the therapeutic recommendations for SIADH discussed here do not apply in this setting. These patients generally have mild, asymptomatic hyponatremia due to downward resetting of the threshold for both ADH release and thirst. Because osmoregulatory function is normal around the new baseline, attempting to raise the plasma sodium concentration increases ADH levels and makes the patient very thirsty, a response that is similar to that seen with water restriction in healthy subjects. Thus, efforts to raise the plasma sodium concentration are both unnecessary and likely to be ineffective. Treatment should be primarily directed at the underlying disease.
Treatment of Hyponatremia in Edematous States
Raising the plasma sodium concentration in patients with edema may be more difficult than in those conditions described earlier. Most of these individuals have advanced CHF or liver disease. Consequently, sodium administration is generally contraindicated.
Congestive Heart Failure
Restricting water intake is the mainstay of therapy in hyponatremic patients with heart failure, although this is often not tolerable because of the intense stimulation of thirst. The combination of an angiotensin-converting enzyme (ACE) inhibitor (or angiotensin receptor blocker [ARB]) and a loop diuretic may induce an elevation in the plasma sodium concentration [38,39]. Tolvaptan may be useful in some patients (see above) but must be initiated in the hospital. Conivaptan is not indicated for treatment of hyponatremia in this setting.
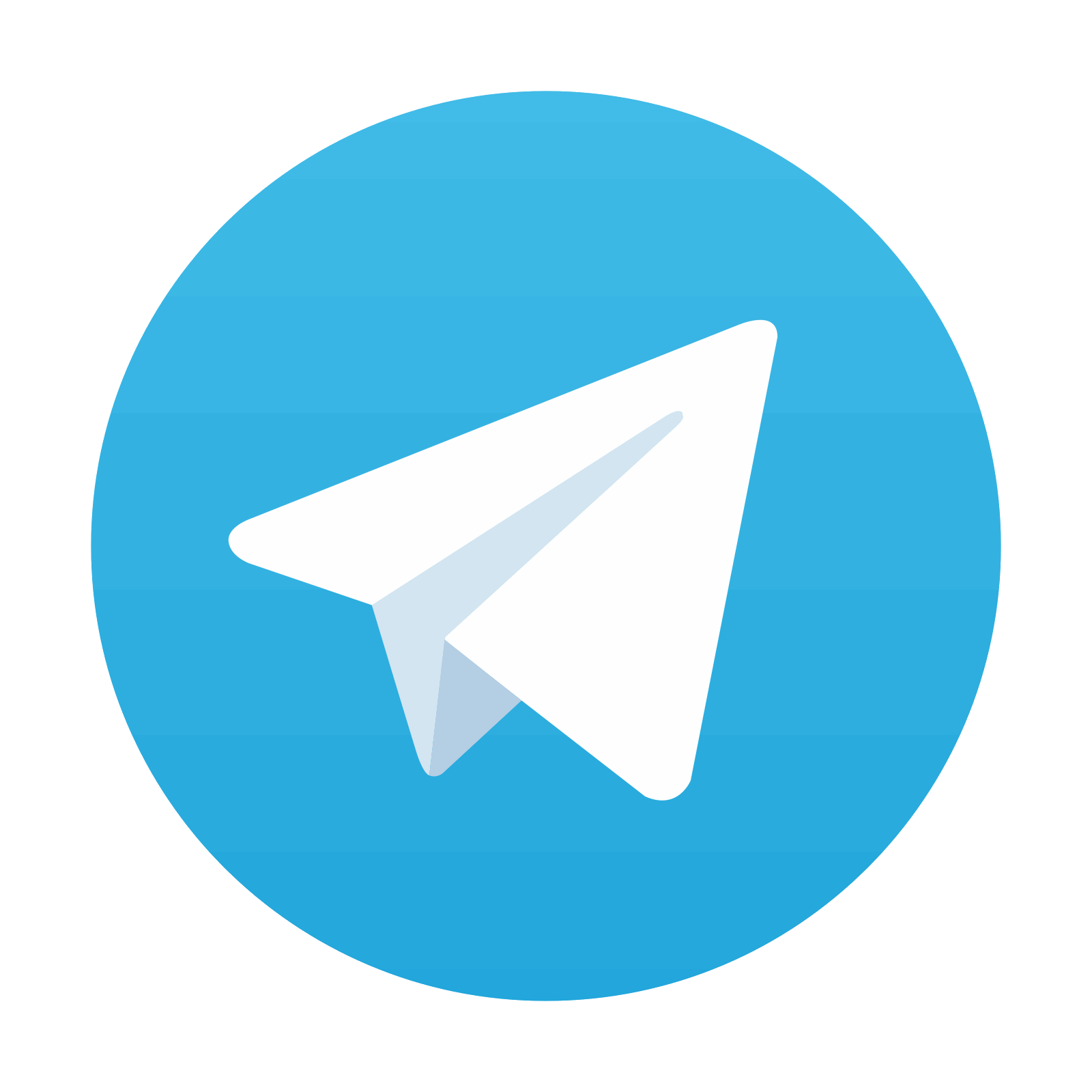
Stay updated, free articles. Join our Telegram channel

Full access? Get Clinical Tree
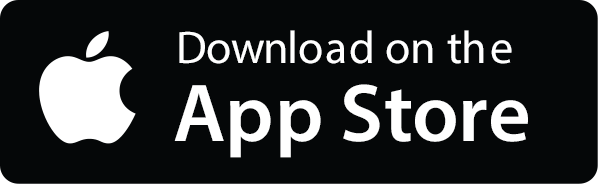
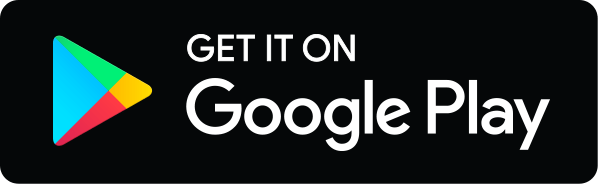
