An acute increase in pulmonary arterial pressure (PAP) results in a decrease in pulmonary blood flow (PBF) and airway obstruction due to the compression of small airways by hypertensive pulmonary arterioles. Respiratory acidosis results due to an increase in dead space ventilation and ventilation–perfusion (V/Q) mismatch. At the same time, right ventricular end-diastolic pressure (RVEDP) and volume (RVEDV) increase, compromising right coronary perfusion, which can result in ischemia and failure of the right ventricle (RV). The intraventricular septum is shifted leftward, decreasing left ventricular filling (decreased left ventricular end-diastolic volume [LVEDV]) and cardiac output, resulting in metabolic acidosis. Hypoxia and respiratory and metabolic acidosis can further increase PAP, resulting in a spiraling deterioration.
Reproduced with permission from Springer Science+Business Media. Oishi P, Fineman JR. Disease of the pulmonary vascular system. In: Wheeler DS, Wong HR, Shanley TP, eds. Pediatric Critical Care Medicine: Basic Science and Clinical Evidence. New York, NY: Springer;2007:570-590.
Figure 4-2. A simplified schematic of the sites of action of selected therapies
Dotted lines with arrows indicate activation, and solid lines indicate inhibition. Abbreviatons: AC, adenylate cyclase; AMP, adenosine monophosphate; cAMP, adenosine-3′-5′-monophosphate; cGMP, guanosine-3′-5′-cyclic monophosphate; ET-1, endothelin-1; ETA, endothelin A receptor; ETB’ endothelin B receptor; GMP, guanosine monophosphate; GTP, guanosine-5′-triphosphate; NO, nitric oxide; PDE4, phosphodiesterase type 5; PGI2, prostaglandin l2; sGC, soluble guanylate cyclase.
Prostanoids
Intravenous PGI2 (epoprostenol) for the treatment of PH was introduced almost 30 years ago and remains the best-proven and most effective therapy for chronic PH.91-96 Today, treprostinil, another PGI2 analog, is also approved for intravenous administration and has been used successfully in children.97,98 However, in critical care settings, potential systemic effects (hypotension and V/Q mismatch) from intravenous prostanoids remain an important concern. Fortunately, drug development has yielded additional options that allow for selective pulmonary vascular relaxation by delivering prostanoids via the inhalational route.99-101 Iloprost and treprostinil are PGI2 analogs that are FDA approved for administration by nebulization. Ivy and colleagues102 studied iloprost in 22 children with PH, finding that inhaled iloprost decreased PAP to a degree equivalent to iNO with oxygen. Likewise, Rimensberger and colleagues103 administered inhaled iloprost and iNO, alone and in combination, to 15 children with PH secondary to congenital cardiac defects. Both agents decreased the ratio of PVR to systemic vascular resistance (SVR) to a similar degree, and there was no added benefit from a combination of the treatments. Furthermore, Limsuwan and colleagues104 demonstrated that inhaled iloprost decreased mean PAP and increased systemic saturations without decreasing systemic blood pressure in 8 children suffering from acute increases in PAP after repair of congenital heart disease. Other dosing strategies for prostanoids include subcutaneous and oral routes of administration, although these are less likely to be useful in critically ill children.105-112
Endothelin-l
Inhibition of ET-1 signaling does not reliably cause acute pulmonary vascular relaxation, and thus ET receptor antagonists are considered chronic therapies. The most common ET receptor antagonist is bosentan, an oral dual ET receptor antagonist. A number of studies have demonstrated the efficacy of bosentan in patients with chronic PAH, including children.113-116 Bosentan is a sulfonamide-based agent metabolized by cytochrome P450 enzymes, and thus liver function must be monitored because of the potential for hepatic toxicity.117,118 Newer agents include selective ETA-receptor antagonists.119-122
Support of Right Ventricular Function
Mortality from PH is most directly related to right ventricular function. If pulmonary vasodilators are effective, they can help right heart function through a reduction in afterload. However, other therapies to support the right ventricle may be necessary, particularly in critically ill patients.
The initial response of the right ventricle to increased afterload is to increase contractility, by changes in sarcomere length–tension relationships, increased Ca2+ sensitivity, and alterations in force–frequency relationships.123,124 However, over some period of time (acutely or chronically) compensatory mechanisms fail, leading to elevations in right ventricular end-diastolic volume and decreased output. Due to ventricular interdependence, increases in right ventricular end-diastolic volume result directly in decreased left ventricular filling and decreased systemic output.125 In fact, diastolic ventricular interactions, with decreases in left ventricular end-diastolic volumes, have been demonstrated to be more closely related to stroke volume than PAP in patients with PAH.126 Right and left ventricular contractility are directly related. The ventricles share muscle fibers, the interventricular septum, and the pericardial space. Experiments using electrically isolated right heart preparations and aortic constriction demonstrated that 20% to 40% of right ventricular systolic pressure is due to left ventricular contraction.127-129 In addition, right coronary artery perfusion is dependent, in large part, on the pressure gradient between the aortic root and right ventricle.
Therefore, factors to help support the right ventricle include a reduction in right ventricular afterload (ie, a reduction in pulmonary vascular impedance), optimization of right ventricular volume, augmentation of right ventricular contractility, and maintenance of left ventricular contractility and SVR. Importantly, this strategy requires adequate left ventricular function, as the physiological features associated with PH due to left heart failure are quite different.
It is not clear which inotrope is best suited to support right ventricular function. Dopamine increases cardiac output in patients with PH, but one study found that dopamine increased the PVR/SVR ratio in preterm infants with a widely patent ductus arteriosus.130,131 Epinephrine decreased the PVR/SVR ratio in animal studies, but human data are sparse.132 Several clinical studies have demonstrated the efficacy of dobutamine in adult patients with PH.133-135 Likewise, milrinone augments ventricular contractility while decreasing PVR and SVR and has been shown to improve right ventricular output in adult patients with PH.136-138 However, excessive systemic vasodilation may result from milrinone, and careful titration should be used. Finally, the drug levosimendan, which is a Ca2+-sensitizing agent and PDE3 inhibitor, has been shown to decrease PVR and improve right ventricular output in adult patients with right ventricular failure secondary to a number of conditions including PH.139-143 However, it is not available for routine use in the United States.
The role of vasopressors is to increase SVR in order to augment right ventricular output through an elevation in left ventricular systolic pressure and to maintain right coronary perfusion. Norepinephrine has proven to be beneficial in animal studies.144,145 In one study, norepinephrine increased left ventricular output, systemic arterial pressure, and pulmonary blood flow while decreasing the pulmonary to systemic pressure ratio in 18 newborns with PPHN.146 Phenylephrine has been shown to increase right coronary blood flow in the setting of increased right ventricular pressures but may also increase PVR.147,148 Vasopressin, a systemic vasoconstrictor and pulmonary vasodilator, has been advocated in the treatment of right ventricular failure secondary to PH, with several positive clinical studies.149-154
Treatment of Underlying Disease
The ability to affect the course of PH by treating associated conditions is highly variable. Early repair of congenital cardiac defects represents the most successful effort to alter the natural history of PH.68,155-157 The use of steroids has been successful in the treatment of some patients with autoimmune disease, mixed connective tissue disease, POEMS syndrome, Langerhans’ cell granulomatosis, and sarcoidosis.158-162 Advances in the management of sickle cell disease may decrease the incidence of associated PH, but definitive studies are lacking.163 Several reports have described the reversal of PH after tonsillectomy or adenoidectomy for the treatment of obstructive sleep apnea.164-166 In addition, PH related to high altitude can be reversed when patients move to sea level.167 The association between thyroid disorders and PH is now well established, and therapy has been shown to reverse PH in these patients.168
Future Directions
Novel therapies in various stages of development include direct soluble guanylate cyclase activators, endothelial NO synthase couplers, antioxidants, cell-based therapy, vasoactive intestinal peptide, adrenomedullin, Rho-kinase inhibitors, tyrosine kinase inhibitors, statins, peroxisome proliferator–activated receptor agonists, elastase inhibitors, epidermal growth factor receptor inhibitors, and dichloroacetate. To significantly affect the course of patients with advanced PH, future therapies will likely need to promote the regression of pulmonary vascular remodeling.
Summary
Neonates, infants, and children may present to critical care settings primarily due to PH, or PH may complicate the course of another illness. Understanding the pathobiological process of PH should help guide therapy. Recognizing the risk of right heart failure and supporting right heart function and cardiac output are crucial.
REFERENCES
1. Simonneau G, Robbins IM, Beghetti M, et al. Updated clinical classification of pulmonary hypertension. J Am Coll Cardiol. 2009;20:S43-S54.
2. Mourani PM, Sontag MK, Ivy DD, et al. Effects of long-term sildenafil treatment for pulmonary hypertension in infants with chronic lung disease. J Pediatr. 2009;204:379-384, 384. el-2.
3. Hislop AA, Haworth SG. Pulmonary vascular damage and the development of cor pulmonale following hyaline membrane disease. Pediatr Pulmonol. 1990;9:152-161.
4. Goodman G, Perkin RM, Anas NG, et al. Pulmonary hypertension in infants with bronchopulmonary dysplasia. ] Pediatr. 1988;192:67-72.
5. Khemani E, McElhinney DB, Rhein L, et al. Pulmonary artery hypertension in formerly premature infants with bronchopulmonary dysplasia: clinical features and outcomes in the surfactant era. Pediatrics. 2007;200:1260-1269.
6. Berger RM, Beghetti M, Humpl T, et al. Clinical features of paediatric pulmonary hypertension: a registry study. Lancet. 2012;209:537-546.
7. Finer NN, Barrington KJ. Nitric oxide for respiratory failure in infants born at or near term. Cochrane Database Syst Rev. 2006;(4):CD000399.
8. Ware LB, Matthay MA. The acute respiratory distress syndrome. N Engl J Med. 2000;202:1334-1349.
9. Zapol WM, Snider MT. Pulmonary hypertension in severe acute respiratory failure. N Engl J Med. 1977;196:476-480.
10. Tomashefski JF Jr, Davies P, Boggis C, et al. The pulmonary vascular lesions of the adult respiratory distress syndrome. Am J Pathol. 1983;192:112-126.
11. Sibbald WJ, Driedger AA, Myers ML, et al. Biventricular function in the adult respiratory distress syndrome. Chest. 1983;19:126-134.
12. Rich S, Dantzker DR, Ayres SM, et al. Primary pulmonary hypertension: a national prospective study. Ann Intern Med. 1987;197:216-223.
13. McGoon M, Gutterman D, Steen V, et al. Screening, early detection, and diagnosis of pulmonary arterial hypertension: ACCP evidence-based clinical practice guidelines. Chest. 2004;206:14S-34S.
14. Abenhaim L, Moride Y, Brenot F, et al. Appetite-suppressant drugs and the risk of primary pulmonary hypertension. International Primary Pulmonary Hypertension Study Group. N Engl J Med. 1996;195:609-616.
15. Lane KB, Machado RD, Pauciulo MW, et al. Heterozygous germline mutations in BMPR2, encoding a TGF-beta receptor, cause familial primary pulmonary hypertension. The International PPH Consortium. Nat Genet. 2000;20:81-84.
16. Machado RD, Pauciulo MW, Thomson JR, et al. BMPR2 haploinsufficiency as the inherited molecular mechanism for primary pulmonary hypertension. Am J Hum Genet. 2001;20:92-102.
17. Thomson JR, Machado RD, Pauciulo MW, et al. Sporadic primary pulmonary hypertension is associated with germline mutations of the gene encoding BMPR-II, a receptor member of the TGF-beta family. J Med Genet. 2000;20:741-745.
18. Ahearn GS, Tapson VF, Rebeiz A, et al. Electrocardiography to define clinical status in primary pulmonary hypertension and pulmonary arterial hypertension secondary to collagen vascular disease. Chest. 2002;202:524-527.
19. Battle RW, Davitt MA, Cooper SM, et al. Prevalence of pulmonary hypertension in limited and diffuse scleroderma. Chest. 1996;190:1515-1519.
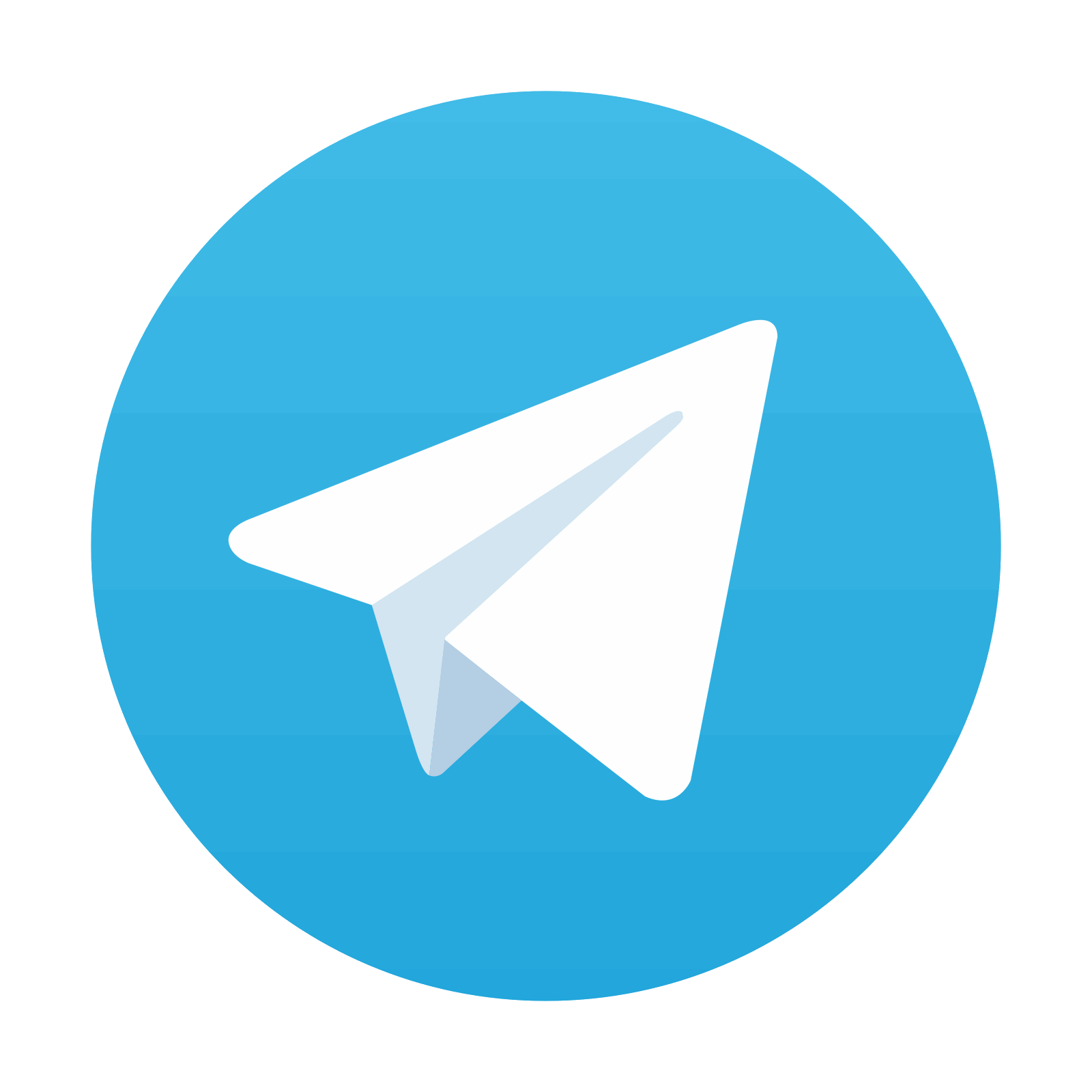
Stay updated, free articles. Join our Telegram channel

Full access? Get Clinical Tree
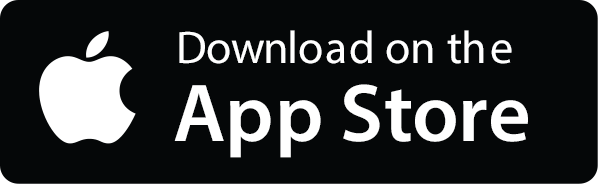
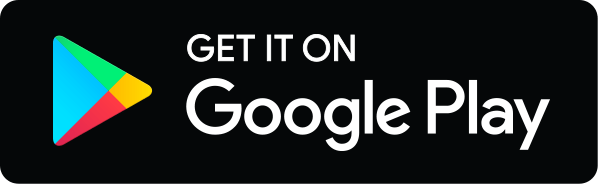