Questions
- 1.
- 2.
How is normal neuromuscular transmission accomplished?
- 3.
Describe the mechanism of action of succinylcholine.
- 4.
How is the action of succinylcholine terminated?
- 5.
Explain the potential side effects of succinylcholine administration.
- 6.
What are the contraindications to succinylcholine administration?
- 7.
What is the best and safest neuromuscular blocking drug for rapid-sequence induction?
- 8.
Are there any drugs that could replace succinylcholine in the future?
An otherwise healthy, 16-year-old boy weighing 60 kg is scheduled for urgent appendectomy. Following preoxygenation, rapid-sequence induction is carried out with propofol 130 mg, fentanyl 150 μg, and succinylcholine 80 mg intravenously. Cricoid pressure is maintained throughout induction. Direct laryngoscopy and tracheal intubation are easily accomplished. Ventilation is controlled and anesthesia is maintained with 2% sevoflurane in 80% oxygen with air. During surgery, the patient receives 500 mL of 0.9% saline and 1 g of cefazolin. Vital signs are stable throughout, and the procedure is completed 35 minutes after induction of anesthesia. As the skin is being closed, sevoflurane is discontinued, and 100% oxygen is administered. At 20 minutes after the end of the procedure, the end-tidal concentration of sevoflurane is 0.0%, heart rate and blood pressure are increasing, end-tidal carbon dioxide (ETCO 2 ) is 35 mm Hg, and there is no spontaneous respiration.
1
What is in the differential diagnosis for a healthy patient whose vital signs are stable but who is not “waking up” after general anesthesia?
Assuming stable and appropriate blood pressure, heart rate, arterial oxygen saturation, core temperature, and ETCO 2 , the differential diagnosis can be divided into three main categories: anesthetic drugs, metabolic disturbances, and neurologic problems.
It is important to consider every drug administered during the perioperative course, including opioids, benzodiazepines, potent inhaled anesthetics, neuromuscular blocking agents, and other drugs such as ketamine and dexmedetomidine. Did an opiate-naïve patient receive enough fentanyl to produce apnea after a short procedure? Was a long-acting neuromuscular blocking drug administered shortly before the end of a procedure? The metabolism of each drug must be considered as well. Is there something interfering with the metabolism of one or more agents? Are small amounts of many agents working additively to produce unconsciousness and apnea?
Metabolic disturbances in a patient with normal vital signs can also produce prolonged unconsciousness and apnea. Hyponatremia, which can be produced by plasma dilution during transurethral prostate surgery, can lead to severe mental status changes. Hypoglycemia, which may follow insulin administration in a fasting patient, can also produce unconsciousness. Hypocalcemia, which may follow administration of multiple units of banked blood products, can lead to such profound weakness as to produce apnea, especially in malnourished and frail patients.
Finally, prolonged hypotension and hypoperfusion can result in global neurologic sequelae. Misuse and misinterpretation of blood pressure monitoring devices can lead to this type of disaster. Documented cases exist of a patient undergoing surgery and general anesthesia in the sitting position, with a noninvasive blood pressure cuff on the calf. The pressure gradient between the brain and the lower extremities can be significant. Prolonged hypoperfusion of the brain has resulted in adverse outcomes despite “normal” blood pressure readings that were measured in a dependent lower extremity.
2
How is normal neuromuscular transmission accomplished?
As the action potential of a motor neuron depolarizes its terminal, an influx of calcium ions causes vesicles containing acetylcholine to fuse with the terminal membrane, releasing acetylcholine into the synaptic cleft. Acetylcholine molecules diffuse across the synaptic cleft and bind to nicotinic cholinergic receptors on the motor end plate ( Figure 20-1 ). The binding of acetylcholine to these receptors produces conformational changes in transmembrane ion channels that allow sodium and calcium ions to flow into the muscle cell, generating an end plate potential. If enough receptors are bound by acetylcholine, the motor end plate potential will be strong enough to depolarize the perijunctional membrane of the muscle fiber, and voltage-controlled sodium channels within this portion of the muscle membrane will open, producing the beginning of an action potential, which is propagated along the muscle fiber.

3
Describe the mechanism of action of succinylcholine.
Succinylcholine is the only depolarizing neuromuscular blocking drug in clinical use. Its blocking properties are due to the chemical mimicry of acetylcholine. Succinylcholine acts as an agonist at the nicotinic cholinergic receptors at the motor end plate, producing a muscle action potential and the muscle fasciculations associated with succinylcholine administration. However, in contrast to acetylcholine, succinylcholine is not metabolized by acetylcholinesterase, and the concentration of succinylcholine in the synaptic cleft remains relatively high for a period of time. As long as succinylcholine remains in the synaptic cleft, it binds to nicotinic cholinergic receptors there. The result is continuous motor end plate depolarization and neuromuscular blockade.
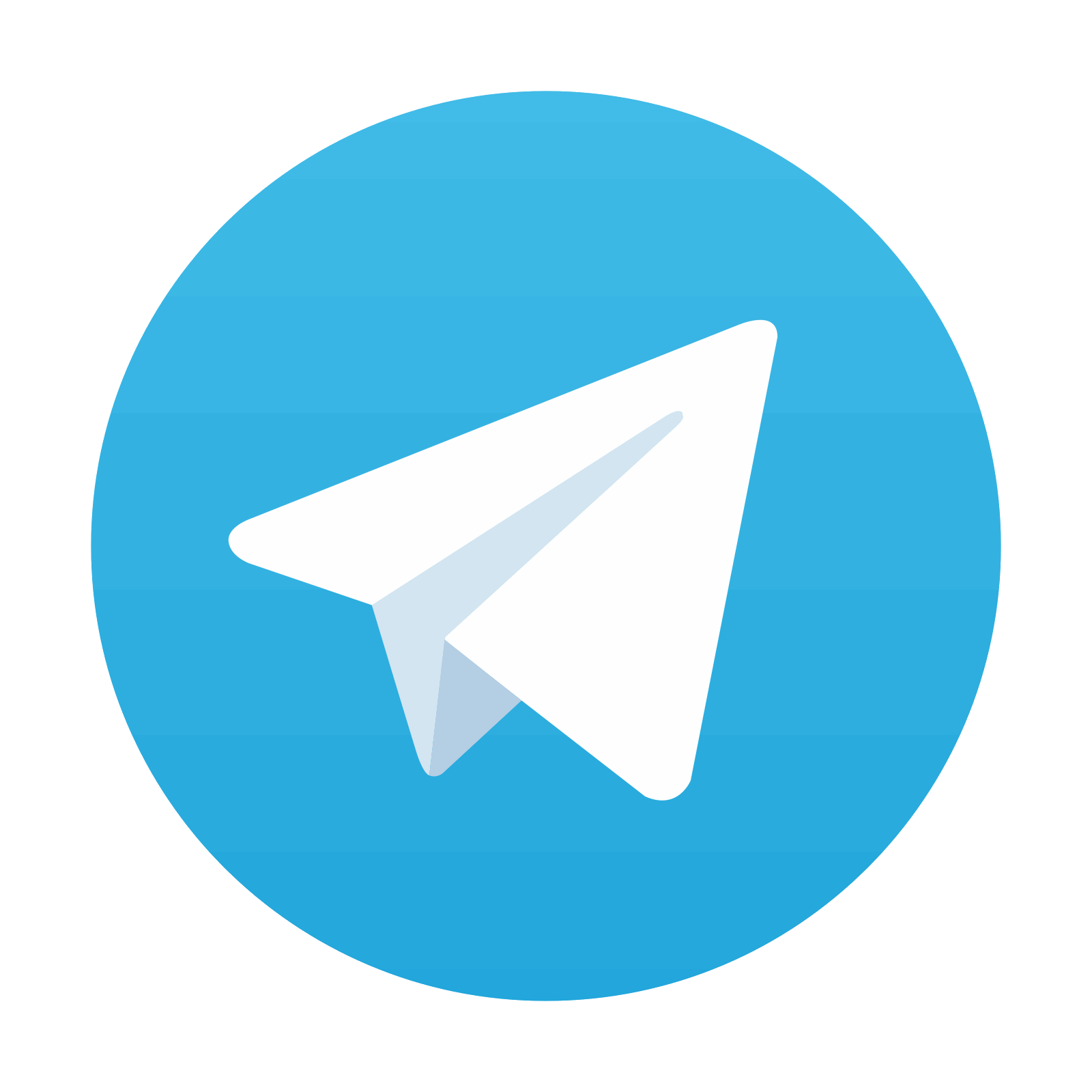
Stay updated, free articles. Join our Telegram channel

Full access? Get Clinical Tree
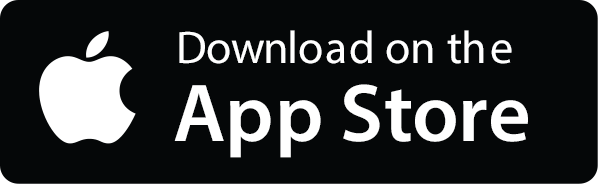
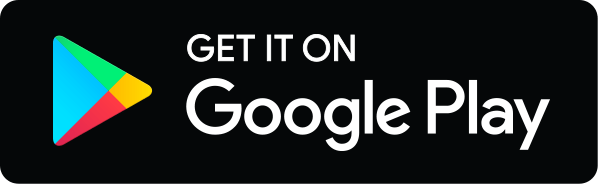