Abstract
Intravenous fluids are a core therapy in critical care and perioperative practice. Although most fluids that we use today are very similar to those of the mid 20 th Century, our understanding of the physiology of the cardiovascular system, the microcirculation and the extravascular space has evolved considerably. In modern practice, thought should be given to the distribution of stressed and unstressed blood volume, the glycocalyx and the gelatinous interstitial matrix. All of these components change dynamically in volume depleted states.
In the dehydrated patient, if given slowly, most intravenous fluid remains in the circulation. However, if delivered rapidly, much of the it extravasates into the interstitium causing hydraulic damage and oedema.
In general, isotonic balanced crystalloid solutions should be used in preference to 0.9% saline for resuscitation, and hypotonic crystalloid solutions, containing generous quantities of potassium, should be used for maintenance. There is minimal indication for colloidal products in modern medicine, with the exception of human albumin solution in liver disease. Blood products are indicated as the primary resuscitation tool in haemorrhagic shock.
After reading this article, you should be able to:
- •
understand fluid distribution within body compartments, the stressed and unstressed blood volume and the body’s response to volume loss
- •
understand the interaction between the microcirculation and the interstitial space, fluid extravasation, tissue oedema and transcapillary refill
- •
understand the current status of colloid solutions, such as gelatins and human albumin solution, in critical care and hepatology
- •
understand blood products, their contents and modern damage control transfusion strategies
Introduction
The administration of intravenous fluids and blood products remains a core component of anaesthesia, perioperative medicine and critical care. It is also a highly controversial field of practice. For more than 100 years, crystalloids have been delivered to patients intravenously, with the objective of reversing shock, replacing fluid losses, maintaining homeostasis, and administering drugs to patients. Many of the intravenous fluids that we use today are near identical to those used in the mid 20 th century. They can be classified as isotonic fluids, that replace extracellular fluid losses, hypotonic fluids, that maintain body water and deliver glucose and electrolytes, and hypertonic fluids that are used principally in hyponatremia or traumatic brain injury. Blood products, including red cell concentrate, plasma, platelets and fibrinogen, are given to replace blood loss and to treat coagulation deficits.
To appreciate the purpose and value of intravenous fluids in the 2020s, it is important to consider the current understanding of the circulation, the endothelium and the extracellular space.
Body “water”
The human body is made up principally of water, into which is dissolved organic and mineral salts, and compounds bundled into and around cellular structures built on an exoskeleton. Males contain relatively more water (on average 60%) than females (on average 50%); neonates have very high total body water (TBW) (70%–80%); elderly patients have relatively lower TBW (52% for males, 45% for females). Body water is neither liquid nor easily accessible. It is divided between two major compartments: intracellular (ICF), containing two-thirds of TBW and extracellular (ECF), containing one-third. Of ECF: one-quarter is located within the intravascular compartment, and three-quarters is extravascular or interstitial. The intravascular compartment contains plasma into which various proteins and cells are suspended. Leucocytes and red cells make up 45% or more of whole blood; their contents are intracellular fluid.
The fluid and electrolyte balance in the body is controlled by gastrointestinal intake, the neurohormonal system and the kidneys. Patients can lose blood and extracellular fluid by a variety of mechanisms and are often unable to take food, fluids and electrolytes enterally. This results in depletion of water, glucose and electrolytes.
The macrocirculation
Blood flows through the body via two circulations, the pulmonary circulation, that receives the entire cardiac output, and the systemic circulation that consists of two compartments: a fast compartment that distributes blood to the brain and extremities and a slow compartment that perfuses splanchnic organs. These are the bowel, spleen, kidneys and the liver. The head and neck holds 15% of blood volume, the thorax (including the heart and lungs), 25%; the abdomen and pelvis, 30%; the arms 10% and the legs 20%. On average, the heart and lungs contain 850 ml of blood, the arteries, arterioles and capillaries contain approximately 1000 ml of blood, and the remaining 3150 ml is in the veins: two-thirds located in the extremities and one-third in the splanchnic circulation.
Of the entire systemic circulation, only 1250 ml is in the “stressed” state, the volume that stretches vessel walls, propelling blood forward from and back to the heart. The remainder is “unstressed”. This is blood that acts as a reservoir for autotransfusion in situations of blood loss.
When there is loss of circulating blood volume, such as in hypovolemic shock, the body prioritises blood flow to the heart and brain. The stress response (ASR) results in high levels of circulating catecholamines, vasopressin and cortisol, and there is widespread arterial and venous vasoconstriction. The unstressed blood volume is mobilized from the extremities and splanchnic circulation resulting, clinically, in a reduced capillary refill time, inactivated bowel transit, reduced glomerular filtration, and impaired hepatic processing capability, particularly for lactate. At levels of volume loss up to 30%, the blood pressure and stroke volume remain within normal limits, but there is increased stroke volume variability with respiration, a lactic acidosis and increased arterial-venous extraction of oxygen, illustrated as a reduction in the mixed venous oxygen saturation (SvO 2 ). This represents an oxygen debt that increases as volume loss escalates. Blood loss beyond 30% results in hypotension. Profound hypovolaemia ultimately manifests with impaired levels of consciousness, hyperventilation, mottling of the extremities, damage to the intestinal mucosa and bacterial translocation.
The microcirculation
The microcirculation is the largest single organ in the body and likely the most misunderstood, largely due to the persistence of the flawed “Starling” model of microcirculatory activity. Data accumulated over the past 30 years have demonstrated the presence and importance of the capillary glycocalyx – a thin gelatinous layer consisting of glycoproteins, glycolipids and proteoglycans that lines the inner surface of blood vessel walls, particularly the capillaries. This layer has a variety of complex physiological roles that include: providing a selective barrier to the passage of molecules and ions through the vascular wall into the interstitium, regulation of vascular permeability to fluid and gas, control of clotting and inflammation, and as a sensory organ that senses stress and redistributes blood flow. Very little fluid actually leaves the circulation. Capillaries filter only 5.5 ml/minute, 1 ml of fluid extravasates for every 100 ml of plasma flow, 8000 ml per day. Albumin tends to be adsorbed within the glycocalyx – and is transported through pores in the endothelial cells rather than between those cells.
Virtually all fluid and albumin that enters the interstitium and is not taken up by cells is reabsorbed by lymphatics. Extremity oedema should be considered “lymphatic overload”.
The interstitium
The interstitial space contains a large volume of water, but is not liquid. Instead, it a gelatinous matrix exists that provides a scaffold for cells. It consists of collagen, elastin, glycosaminoglycans, proteoglycans, various other glycoproteins and cells (macrophages, mesenchymal fibroblasts and leucocytes). The interstitium should be considered an active organ that acts as a transport centre for nutrients (glucose and oxygen), water (molecule by molecule), nitrogenous waste products and carbon dioxide. It is essential for immune function and tissue repair, and it helps regulate cellular behaviour. The interstitial structure also changes dynamically in response to changes in circulating volume and hydration.
At rest the interstitial pressure is negative and the tissue is gelatinous. When a patient becomes hypovolaemic, from bleeding or dehydration, the interstitium becomes minimally compliant. Albumin is compressed into the subcapillary space, obliterating tissue oncotic pressure and the positive pressure applied by the compressed interstitium prevents fluid filtration.
In overhydrated states, there is an increase in the filtration of fluid, but the corresponding rise in interstitial pressure eventually reduces filtration, and the liquid is rapidly removed by lymphatics. The interstitium is highly vulnerable to hydrostatic damage. This hydraulic fracturing (“fracking”) of the matrix associated with fluid overload reduces tissue elastance. As more and more fluid enters the space the interstitium becomes progressively more compliant, pockets of liquid collect and oedema forms. Although it is widely believed that crystalloid expands the interstitium in a ratio of 1:3 or 1:2, this is only the case with initial resuscitation: with persistent over-resuscitation or replacement of blood with crystalloid, that ratio escalates geometrically up to 8:1. Widespread oedema forms when the interstitial volume increases by more than 30%.
Volume kinetics and the interstitium
While administration of intravenous fluids is certainly beneficial in most clinical settings, it has no teleological basis and defeats biological controls of fluid and electrolyte balance. The positive or negative impact of those fluids is determined by 1. Hydration status, 2. Speed and volume of administration, 3. Osmolality, 4. Electrolyte composition.
With severe dehydration or volume loss (such as bleeding), adrenergic and neuroendocrine responses activate and mobilize the unstressed blood volume, encourage transcapillary refill and prevent fluid elimination and redistribution. The interstitium is non-compliant. When intravenous fluid is administered at moderate rates, the immediate impact is to restore the unstressed volume. Most of the initial fluid is maintained in the vascular system, particularly while the infusion is continuing, and fluid redistribution to the interstitium is delayed.
With normovolaemia or mild hypovolemia, the response to administration of intravenous fluid various depends on whether the patient is undergoing an ASR or not. For example, a volunteer receiving intravenous fluids will excrete most of the fluid with minimal redistribution to the interstitium. Conversely, during surgery, or when the patient is undergoing an ASR, such as sepsis or trauma, fluid is retained in the body and much of it is redistributed to the interstitium. Although, theoretically, the distribution of fluid across the extracellular space is 25% intravascular and 75% extravascular (1:3) in reality a proportion of the interstitium is completely non compliant (e.g. bones, the skeleton, capsules etc) so the ratio is likely 1:2. Although isotonic fluid should distribute, assuming minimal urinary elimination, in this ratio of 1:2 – the reality differs depending of on the clinical situation (state of hydration), the speed of fluid delivery and the volume administered.
Studies of “volume kinetics” using haemoglobin dilution by Robert Hahn have enhanced our knowledge of what happens to intravenous fluids in volunteers and in patients during surgery. Hahn introduced the concept of the “half life (tf 1/2 )” of infused fluids. The tf 1/2 is the time required to eliminate 50% of the infused volume. The longer the tf 1/2 , the longer the expansion of the intravascular and interstitial spaces. In awake volunteers the crystalloid tf 1/2 is 8 minutes. Under anaesthesia, during surgery, while there is rapid expansion of both the intravascular space and the interstitium. The higher the mean arterial pressure the more rapid the elimination of fluid from the body, and the lower the volume expansion of the interstitium, the lower the tf 1/2 . As extravascular fluid accumulation may result in pulmonary oedema, pleural effusions, wound complications, ileus, dysnatraemia and other postoperative problems, there appears to be little argument for treating perioperative iatrogenic hypotension with fluid versus vasopressors. Indeed, accumulating data in sepsis appears to support earlier administration of vasopressors in septic shock versus fluids.
The rate of administration of intravenous fluids also dramatically impacts the quality of intravascular resuscitation versus interstitial accumulation. Large volumes of fluid infused quickly, using rapid infusers or by squeezing bags, result in disproportionately higher extravascation of fluid resulting in longer tf 1/2 . It is unclear whether this is a pressure or a volume effect but, regardless, the lymphatics are overwhelmed and fluid accumulation occurs. Hence if a fluid bolus is considered appropriate, it is more effective to infuse 250 ml over 20 minutes rather than 1000 ml over 10 minutes.
Intraoperatively administered fluid may linger in the interstitium for up to 72 hours. Once surgery ends, 30 minutes or so following emergence from anaesthesia, blood pressure normalises and the tendency to sequester fluid recedes. Urinary output in response to fluid administration increases and much of what is subsequently administered is eliminated. Nevertheless care taken with the choice of fluids administered in the postoperative period. Isotonic crystalloids, designed to be facsimiles of protein free plasma, contain inadequate quantities of potassium, phosphate, calcium, glucose and magnesium to maintain homeostasis. Consequently, persistent administration of these fluids into the postoperative period results in hypokalemia, hypomagnesemia, hypophosphatemia and ketosis.
The composition of intravenous fluids also determines the elimination tf 1/2 of fluids delivered in the perioperative period. Hypotonic fluids are rarely administered in the operating room, but 0.9% Saline has a significantly longer tf 1/2 than sodium lactate solutions (Hartmann’s, lactated Ringer’s etc). It is unclear whether this is as a result of the high Chloride content or the higher osmolality. Regardless, a shorter tf 1/2 is preferable, presuming that larger volumes that persist in the interstitial space for longer result in more complications.
Finally, it is important to recognize that fluid distribution and fluid elimination tf 1/2 is different in critical care than in perioperative medicine (POM). The ASR in critical care is longer, resulting in prolonged duration (days) of fluid sequestration. This results in significant fluid accumulation, with potentially devastating complications including hypoxic respiratory failure, acute kidney injury, ileus and abdominal compartment syndrome. Although significant volume resuscitation may be required early in critical illness, albeit this remains controversial, blood loss should be replaced with blood products and crystalloid resuscitation limited. Urinary output is often limited due to large circulating quantities of stress hormones, and patients rapidly develop a positive fluid balance. Unavoidably, fluid is administered to deliver antibiotics, sedatives, electrolytes, intravenous nutrition and other therapies. Simultaneous “maintenance” infusion of isotonic fluids is usually unnecessary in that setting.
The clinician should be aware of the large quantities of solute that is being administered. These solutes will ultimately need to be eliminated by the body: the maximum daily excretion potential of the kidney is between 10 g and 15 g of NaCl (each litre of 0.9% saline contains 9 g). Patients undergoing large volume resuscitation may receive 60 g of salt (1.3 moles of Na + and Cl − ) in the first day, with minimal elimination. Consequently, due to the osmotic effect of this solute, fluid overload may be prolonged and deresuscitation difficult. There is clear evidence that fluid and solute overload worsens outcomes in critical illness. Care should be taken to replace NaCl in electrolyte replacement bags with, for example, dextrose, and to double concentrate antibiotics and pressors where possible.
Crystalloids
Crystalloid solutions are universally used for fluid resuscitation, in hypovolaemic states, fluid replacement in redistributive states, as carriers for electrolyte replacement therapy and total parenteral nutrition, and for maintenance of hydration and electrolytes in patients unable to take water and food orally. They should be seen as “Resuscitation” fluids that are isotonic, and “Maintenance” fluids that are ultimately hypotonic with respect to plasma.
Crystalloid solutions are intravenous fluids that contain electrolytes with or without dextrose dissolved in sterile water. Crystalloid solutions may be hypotonic, isotonic or hypertonic. The hypertonic crystalloids currently licensed for human use are 3% NaCl and 8.4% sodium bicarbonate. Isotonic crystalloids include 0.9% NaCl and a variety of “Balanced (Salt) Solutions (BS)” – sodium lactate solutions (Hartmann’s, lactated Ringer’s), sodium acetate solutions and fully balanced Plasmalyte-148 ( Table 1 ). In general these should be labelled “Resuscitation Fluids.” Hypotonic fluids may be diluted saline or balanced, and may or may not contain dextrose ( Table 2 ). In general these should be labelled as “Maintenance Fluids.”
Na + (mmol/l) | K + (mmol/l) | Cl − (mmol/l) | Ca 2+ (mmol/l) | Mg 2+ (mmol/l) | Lactate (mmol/l) | Acetate (mmol/l) | Gluconate (mmol/l) | Osmolality mOsm/kg | |
---|---|---|---|---|---|---|---|---|---|
NaCl 0.9% | 154 | – | 154 | – | – | – | 308 | ||
Normosol R | 140 | 5 | 98 | – | 3 | – | 27 | 23 | 296 |
Plasmalyte 148 | 140 | 5 | 98 | – | 3 | 27 | 23 | 296 | |
Hartmann’s solution (UK) | 131 | 5 | 111 | 2 | – | 29 | – | – | 278 |
Hartmann’s solution (Aus) | 131 | 5 | 112 | 2 | – | 28 | – | – | 278 |
Ringer’s lactate | 130 | 4 | 109 | 1.5 | – | 28 | – | – | 273 |
Ringer’s acetate (Fresnius Kabi) | 131 | 4 | 112 | 2 | 1 | 30 | – | 270 | |
Ringer’s solution | 147 | 4 | 155.5 | 2.25 | – | – | – | – | 311 |
Na + (mmol/l) | K + (mmol/l) | Cl − (mmol/l) | Glucose (mmol/l) | Mg 2+ (mmol/l) | Acetate (mmol/l) | Osmolality mOsm/kg | |
---|---|---|---|---|---|---|---|
Dextrose 5% | – | – | – | 278 | – | – | 278 |
NaCl 0.45% | 77 | – | 77 | – | – | 144 | |
NaCl 0.45% + dextrose 5% | 77 | – | 77 | 278 | – | – | 422 |
NaCl 0.45% + 5% dextrose + 40 mmol/l KCl | 77 | 40 | 117 | 278 | – | – | 512 |
Maintelyte | 40 | 40 | 20 | 278 | 1.5 | 23 | 402 |
Solution 18 (1.8% NaCl + 4% dextrose) | 30 | – | 30 | 222 | – | – | 284 |
Resuscitation fluids can be conveniently delineated into “Saline” solutions – that contain only Na + and Cl − and are relatively isotonic or hypertonic, and “Balanced” Solutions (BS), that aim to replicate, where possible, the osmolality and electrolyte content of extracellular fluid. There are several problems that all crystalloid resuscitation fluids have in common: 1. Crystalloids do not improve oxygen delivery to the tissues, and while they may restore circulating volume in haemorrhagic shock, they may not resolve it. 2. Crystalloids dilute coagulation factors and disrupt clot formation by multiple mechanisms. Allied with elevated hydrostatic pressures within the vasculature associated with resuscitation, this may increase the risk of devastating further bleeding. 3. Crystalloids “leak” or redistribute into the interstitium at escalating ratios in response to repletion of blood loss. This results in hydraulic fracturing of the interstitium, oedema formation and transudation of fluid into “third” spaces – the alveoli, pleura, abdominal cavity etc., resulting in adverse outcomes.
NaCl 0.9% (“normal saline”)
“Normal saline” (NS) is an equimolar solution of sodium (154 mEq/litre) and chloride (154 mEq/litre). It contains 9 g of NaCl. The solution has an osmolality of 308 mOsm, slightly hypertonic to plasma. As the sodium content is 10 mmol/litre higher than the plasma equivalents and the chloride content 50 mmol/litre higher, administration of moderate to large quantities of this fluid is associated with mild hypernatremia and progressive hyperchloremia. That results in a progressive hyperchloremic metabolic acidosis.
NS continues to be widely used in hospital practice, particularly in medicine, principally out of habit. It is also used in neurosurgery as a component of osmotic therapy. The slight hypertonicity of NS with respect to plasma is believed to prevent intravenous fluid crossing the blood brain barrier and worsening cerebral oedema.
NS is widely used as a carrier fluid for antibiotics, sedative infusions in the intensive care unit and for delivering electrolyte replacement therapy – potassium, phosphate, magnesium etc. Accumulating data strongly indicates that iatrogenic hyperchloraemia (plasma Cl − in excess of 110 mmol/litre), triggered by large volume NS administration, is associated with an elevated risk of acute kidney injury and potentially death. The traditional use of NS in acute diabetic ketoacidosis is also being questioned, with multiple studies demonstrating that this fluid prolongs acidosis (principally by inducing hypercholoremia) and prolongs duration of stay, versus balanced solutions. A persistent, mistaken view, is that NS is safer than balanced solutions in chronic kidney disease due to the absence of K + in the standard formulation. In fact, NS is more likely to cause hyperkalaemia than balanced solutions due to the development of acidaemia.
Hypertonic saline (3%) is used therapeutically to expand plasma volume by osmotically recruiting water from the intracellular and extracellular space. It can be used as an alternative to mannitol in the treatment of cerebral oedema. It is also used to treat neurological complications in acute hypotonic hyponatraemia.
Another hypertonic fluid that is widely available in clinical practice is 8.4% sodium bicarbonate (NaHCO 3 ). This is constituted in a 100 ml bottle, containing 100 mmol of Na + and 100 mmol of HCO 3 − . Sodium bicarbonate is used to temporarily treat acidosis in the setting of iatrogenic hyperchloremia, renal tubular acidosis and acute kidney injury (AKI). Care should be taken in patients with malnutrition or chronic hypotonic hyponatraemia as it may precipitate osmotic demyelination syndrome.
Sodium lactate and sodium acetate solutions
The most widely used balanced salt solution was introduced into clinical practice in 1932 by an American, Alexis Hartmann. Confusingly, in his home country, this fluid is known as “lactated Ringer’s” solution (Hartmann named the fluid after Sidney Ringer, an early proponent of intravenous fluid therapy), and elsewhere in the world it is known as “Hartmann’s Solution.” More confusingly, the formulation of what I will term “Sodium Lactate Solutions (SLS)” is slightly different between the USA, Europe and Australia ( Table 1 ).
Although SLS is considered a balanced solution, it is neither isotonic nor fully balanced. The sodium concentration is lower than plasma, the chloride, and (in Europe and Australia) potassium concentrations are higher. To achieve electrochemical balance, rather than using bicarbonate, which is unstable in mixed solutions, lactate is used as buffer. This makes sense, as the body produces more than 1 mol of lactate per day which is eventually metabolized in the liver to produce carbon dioxide and water. SLS is an excellent choice for fluid therapy in POM. Although its chloride level is relatively high, only modest acidosis occurs due to simultaneous albumin dilution. One major concern that limits the use of SLS as a universal fluid is its relative hypotonicity. Clinicians have tended to avoid SLS in situations where cerebral oedema may be problematic (neurosurgery, traumatic brain injury, diabetic ketoacidosis). Concerns regarding the presence of Lactate in this fluid in the setting of lactic acidosis, in liver dysfunction and in children reflect a misunderstanding of the role of lactate in biochemistry. It is a universal substrate for bioenergetics in the body.
Plasmalyte-148 (and Normosol R)
To resolve potential problems with SLS, ‘fully balanced’ solutions were introduced in the late 1990s. The most widely used product is Plasmalyte-148 (Normosol R is identical). This contains 140 mmol/litre of sodium, 98 mmol/litre of chloride, 5 mmol of potassium, 3 mmol/litre of magnesium, no calcium, and the fluid is ‘balanced’ with a combination of acetate and gluconate. Acetate is easily metabolized. The fate of gluconate in the body is less certain: it is probably converted to glucose via the pentose phosphate pathway and then to HCO 3 − via the Kreb’s cycle. This fluid is now widely used in neuroanaesthesia and neuro critical care.
The absence of calcium in Plasmalyte likely makes it an ideal resuscitation fluid where co-administration of blood products is required. Concerns regarding the potassium concentration (5 mmol/litre) again represent a misunderstanding of physiology – the basal potassium requirement is 1 mmol/kg per day, and in the setting of fluid and electrolyte loss it may be significantly higher.
Several studies have demonstrated that Plasmalyte is superior to NS in the management of DKA, by minimizing HCA and shortening duration of stay.
Infusion strategy
Although anaesthesia textbooks provide suggested infusion strategies for intraoperative crystalloid (based on weight, surgical extent and blood loss) none can be recommended based on best available evidence. The use of pulse pressure variability (PPV) or stroke volume variability (SVV) to predict fluid responsiveness (in mechanically ventilated patients) has a strong evidence base: if PPV/SVV is greater than 12% and 9% respectively, the patient is considered “fluid responsive.” The greater the variability in response to inspiratory–expiratory transition, the more likely the patient’s cardiac output will respond to fluid boluses.
Hypotonic crystalloids (maintenance fluids)
Patients who cannot take oral fluid and calories require intravenous fluid therapy that matches their water and electrolyte requirements. Patients require at least 20 g of glucose per day to prevent ketosis, but 2–4 g/kg is a reasonable estimate of overall requirement. Each day, patients also need 30 ml/kg of water, 1.5 mmol/kg each of sodium, chloride and potassium, 0.5 mmol/kg of phosphate and 0.15 mmol/kg each of magnesium and calcium.
Water cannot be administered into the intravascular space as red cell lysis results. Dextrose as a 5% solution (D5%) is isosmotic with plasma, but rapidly becomes free water as the glucose content is metabolized. Hence, free water therapy has traditionally taken the form of D5% solutions. Dextrose is also prepared in 10%, 20% and 50% concentrations. In general, higher concentrations are used to treat hypoglycaemic states.
In Table 2 I have listed different products that are designed to provide maintenance fluid therapy. None of these has been evaluated in large randomized controlled trials. Care must be taken to avoid bolus infusions to treat hypotension or hypovolaemia in stressed patients (in the operating room or during acute critical illness) as this may result in acute life-threatening hypotonic hyponatraemia, particularly in children.
Hypotonic saline solutions are the most widely used maintenance fluids – usually 0.45% saline (77 mmol/litre of both Na + and Cl − ) with or without glucose. Maintelyte™ (Baxter) contains 40 mmol/litre of Na + and Cl − , 20 mmol/litre of K + , 5% dextrose (20 g/litre = 80 kCal), 1.5 mmol Mg 2+ and is ‘balanced’ by acetate 23 mmol/litre.
Depending on your geolocation, other intravenous fluid therapy bags may be available for specific therapeutic interventions or electrolyte replacement therapy, such as isotonic sodium bicarbonate solution and sodium/potassium phosphate solution. The latter, for example, manufactured by Fresnius (Polyfusor Phosphates), contains Na + 81 mmol, K + 9.5 mmol and PO 4 2− 50 mmol in a 500 ml bag (isotonic).
Colloids
For 50 years many clinicians believed that the fluids containing large molecular weight gelatins or starches could harness colloid-oncotic forces to limit extravasation of fluids into the interstitium, plug damage in the capillary wall (preventing “capillary leak”), recruit fluid from the interstitium into the vascular tree, and prevent tissue oedema formation. These products were considered plasma substitutes that could effectively restore plasma volume without the attendant complications of crystalloids or blood transfusion.
However, dozens of studies, conducted over decades, failed to demonstrate in humans that this ‘colloid’ effect actually occurred. Fluid recruitment and retention were disappointing and the colloidal products likely had more side effects than benefits. In particular, hydroxy-ethyl starches were found to cause coagulopathy and increase the risk of AKI. Moreover, the underlying science behind colloids has been strongly challenged. Currently the only colloidal products that are widely used in clinical practice are succinylated gelatins or human albumin solutions (HAS).
Human albumin solutions
Albumin is commercially available in concentrations of 5% (500 ml bottle) and 20% (50 ml bottle). These solutions are derived from pooled human blood, serum, or plasma and have been pasteurized at 60°C for 10 hours. They contain no clinically important antibodies and can be administered without regard to the recipient’s blood group. All commercial albumin products contain 130–160 mmol of sodium per litre. The 5% solution is iso-oncotic with respect to human plasma; the 20% albumin solution is four to five times more oncotically active than plasma – so this is often referred to as “hyperoncotic albumin.” HAS neither causes allergic reactions nor coagulopathy.
HAS is widely used in hepatology to restore volume following paracentesis, to prevent post-paracentesis circulatory dysfunction, and as part of the therapeutic pathway for spontaneous bacterial peritonitis It has no role in transfusion therapy for major haemorrhage, as plasma products contain an abundance of albumin. There is no clear role for HAS in the treatment of hypoalbuminaemia, as albumin loss in this setting is redistributive, and further redistribution is likely with transfusion. In critical care, the use of HAS is based more on belief in its capacity to minimize fluid overload, optimize resuscitation and enhance fluid diuresis, than on clinical evidence. Serum albumin levels rapidly fall in acute critical illness, from redistribution and hepatic prioritization towards manufacturing inflammatory globulins in the acute phase response. While this may have a clinical impact on the transport of ligands and on acid base status (it causes metabolic alkalosis), colloid osmotic pressure does not change. It is not known whether transfused HAS has the same biological capacity as endogenous albumin.
Currently two main arguments are used to justify the use of HAS in acute critical illness. The first is that, in severe sepsis, restoration of serum albumin levels may improve outcomes; this is not currently supported by evidence. The second it that albumin may have a ‘kidney helping’ effect, by improving the efficiency of intermittent hemodialysis and enhancing diuretic fluid removal. Neither claim is proven. Some data suggest that HAS may worsen outcome in traumatic brain injury (TBI), although this may have been a problem of the hypotonic carrier fluid rather than the albumin itself.
Gelatins
Gelatin solutions have been widely used in Europe. The colloidal compounds are derived from hydrolysis of bovine collagen. The three most common preparations are succinylated gelatin (Gelofusin™), presented in a carrier solution of Na + 154 mmol/litre and Cl − 120 mmol/litre, urea-linked gelatin (Hemaccel™), presented in a carrier solution of isotonic saline plus K + 5.1 mmol/litre and Ca 2+ 6.25 mmol/litre and Geloplasma™, presented in a balanced carrier solution (Na + 150 mmol/litre, Cl − 100 mmol/litre, K + 5 mmol/litre, lactate 30 mmol/litre, Osm 295 mOsm/kg). The gelatin component is anionic. Gelatins appear to expand intravascular volume reasonably well, at least initially, but are rapidly cleared from the circulation, usually by glomerular filtration. Therefore, the use of gelatins is limited to cases in which rapid volume expansion is required, but the source of the problem is rapidly reversible. An example of this situation would be acute vasoplegia associated with neuraxial anaesthesia. Gelatins appear to be reasonably safe in clinical practice, although, again, decades of research has failed to demonstrate any clear benefit from their use.
Blood products
Blood products are usually administered to patients who are actively bleeding, are clinically anaemic or are coagulopathic. Although concerns regarding acquisition of viruses and unexpected adverse reactions have been a problem in the past, contemporary blood transfusion therapy is extremely safe.
Red cell concentrate (RCC) is blood group and Rhesus specific and is typically packed in a 300 ml bag that contains 220 ml of red blood cells (reflecting a very high haematocrit), few or no white cells and 80 ml of plasma. Blood is anticoagulated with sodium citrate solution.
Plasma replacement products are derived from local donations and provided by the transfusion service (‘fresh frozen plasma’) or commercial products such as solvent-detergent treated plasma (Octaplas™). This is a commercial version of plasms that is treated to reduce the risk of transmitting lipid enveloped viruses (HIV, hepatitis B and C) and prions.
In general, clinicians determine “on the go” their own strategy for delivering blood products to the bleeding patient. However, modern ‘damage control’ strategies, particularly in trauma, emphasize earlier use of red-cell concentrate transfusion, earlier use of plasma and platelets, and early consideration of the use of fibrinogen. Most major hospitals now have a “massive transfusion protocol” – where blood products are rapidly mobilized in response to a ‘code red’. Plasma fibrinogen levels are checked and fibrinogen is administered when the level falls below a specific threshold or based on clinical expertise.
References
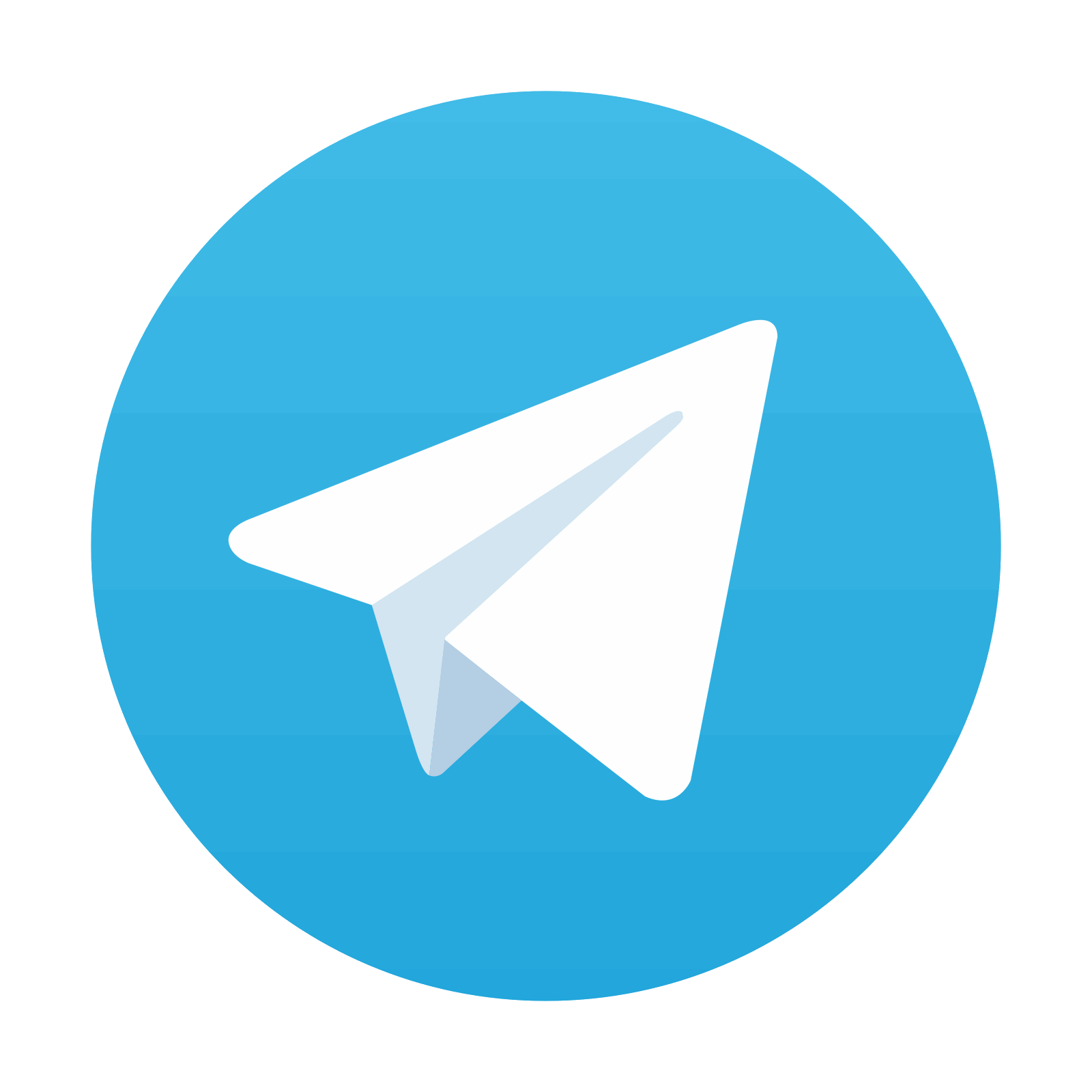
Stay updated, free articles. Join our Telegram channel

Full access? Get Clinical Tree
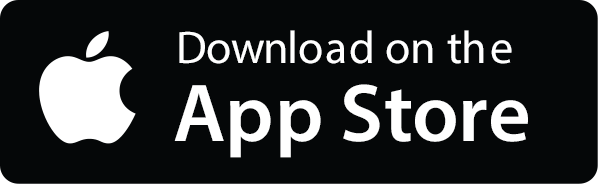
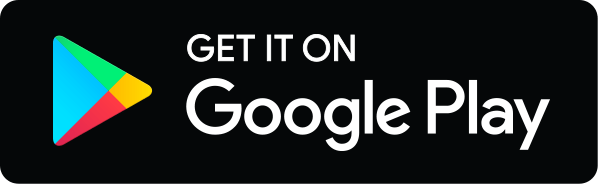
