Critical Care of Acute Stroke
The intensivist is in a position to make substantial contributions to the care of stroke patients. The problem of clinically serious and anatomically large stroke has several aspects that must be considered simultaneously; the most pressing are restoration of blood flow and the emergence of a particularly aggressive form of brain edema and swelling that often has a fatal outcome. Also addressed in the intensive care unit (ICU) are treatments that potentially spare neurons from irreversible death, and a number of more mundane medical issues such as the prevention of aspiration.
GENERAL PRINCIPLES IN STROKE
Treatment of stroke traditionally has focused on anatomic localization, modification of risk factors, and secondary prevention after a transient ischemic attack (TIA) or minor stroke (1). Efforts to limit infarct size after an acute ischemic event have been hampered by the short interval between cessation of blood flow and the cascade of irreversible changes that lead to cell death. With complete global ischemia, this interval may be as short as 4 minutes, essentially precluding any possibility of clinical intervention (2), but the human cerebral circulation provides collateral pathways that allow residual perfusion in portions of the territory of an occluded vessel. Therefore, partial ischemia is probably common in typical stroke that arises from occlusion of large arteries. Experimental models of acute ischemic stroke suggest that partial ischemia affords a longer interval before irreversible ischemic changes occur.
Reduction in cerebral blood flow from normal levels of 50 to 100 mL/100 g per minute to less than 18 mL/100 g per minute results in cessation of brain electric activity, but important cellular functions that maintain neuronal viability may continue (3). When blood flow falls below 10 mL/100 g per minute, basic cellular functions can no longer be maintained, leading to a series of events that result in cell death (4,5). Clinical deficits appear at an early stage, but because irreversible changes may not yet have occurred, the ischemic process should be reversible by raising blood flow above the critical threshold. Both the absolute level of cerebral blood flow and the duration of ischemia determine the development of cerebral infarction (6). Restoration of blood flow within 1 to 3 hours reverses ischemia and reduces infarction in some animal models. In human strokes, the length of time reduced levels of blood flow can be tolerated is unknown and almost certainly varies from case to case as well as between focal and global ischemia. The clinical and biologic features of global ischemia are reviewed in Chapter 17.
Clinical experience shows that ischemic deficits occasionally improve rapidly, within minutes to hours after onset. Experimental and some clinical studies indicate that cellular functions may return toward normal levels after transient brain ischemia and that neuronal death is not determined for up to 24 to 48 hours. The thrust of modern stroke intervention is predicated on this potential reversibility (7,8). In addition to enhancing standard medical care, two innovative strategies have been pursued in this regard; decreasing
neuronal vulnerability to ischemia and improving blood flow.
neuronal vulnerability to ischemia and improving blood flow.
Maximizing Standard Medical Management
Maintenance of adequate blood pressure is a tenet of acute stroke care. In patients with fluctuating basilar or carotid artery syndromes, most neurologists have had the experience that rapid lowering of blood pressure leads to clinical deterioration in some patients. The problem of how to approach the larger group of patients with fixed stroke deficits and hypertension has been addressed by several studies. Wallace and Levy (9) found that 84% of 334 consecutive stroke patients had elevated pressure, only half with a hypertensive history, which declined an average of 20 mm Hg systolic and 10 mm Hg diastolic in the 10 days after stroke. Patients with cerebral hemorrhage had a more variable blood pressure course, as expected. A subsequent case-controlled study by Britton and colleagues (10) found that 69% of stroke patients and 36% of controls had acute hypertension after admission and that a spontaneous decline occurred over the first few days of hospitalization, similar to the Wallace and Levy study. Other studies (11, 12 and 13) have given similar results and have led to the admonition that all but the most extreme hypertension after acute stroke should be left untreated unless there is acute angina, hypertensive encephalopathy, or progressive renal failure (10). Rapid reductions in cerebral perfusion pressure in the setting of ischemia can markedly decrease blood flow and enlarge the ischemic area. We have generally used a threshold of systolic pressures above 200 mm Hg or diastolic pressures over 110 mm Hg before beginning treatment. Rapidly acting agents such as intravenous labetalol are useful, with angiotensin converting enzyme inhibitors favored for more long-acting therapy.
Present knowledge about the pathophysiology of infarction suggests that fever, seizure activity, and hyperglycemia are detrimental to stroke outcome. Human and animal data indicate that neuronal susceptibility to ischemic death is very temperature-dependent. Metabolic energy requirements and many of the enzyme systems that participate in cell dissolution are strictly temperature dependent. This is typified by patients suffering cold water drowning who sustain prolonged anoxia and arrest yet have good recovery of neurological function. Improved outcome in several animal studies has resulted from lowering brain temperature 4°C to 5°C, and profound hypothermia is used successfully in vascular neurosurgery to allow almost complete diversion of blood from the brain. Conversely, seizure activity increases the requirements for metabolic energy and, if sustained, promotes neuronal death even under normal metabolic circumstances. Studies in animals and clinical work also suggest that hyperglycemia may adversely affect infarct size; therefore, maintenance of blood glucose in the normal range has been suggested as an ancillary therapy for acute stroke.
Decreasing Neuronal Vulnerability
Ischemia initiates a series of biochemical and cellular events resulting in irreversible changes in essential cell functions and eventually cell death. Three particular biochemical events have been identified as potentially important in leading to ischemic death: (a) elevated intracellular calcium; (b) production of free radical compounds; and (c) lactic acidosis. During ischemia, diminishing energy supplies compromise the function of the Na+/K+ pump, leading to neuronal depolarization and indiscriminate release of all neurotransmitters. Depolarization and transmitter actions cause massive flux of ions and water; cells swell, extracellular K+ and intracellular Ca2+ rise, and pH falls.
Intracellular free calcium concentration is normally kept almost 10,000 times lower inside than outside neurons. The precipitous rise in intracellular calcium during ischemia may be an important factor in the cascade of metabolic derangements that eventuate in neuronal death. The endoplasmic reticulum
and mitochondria release calcium, causing an increase in intracellular Ca2+, in turn exacerbating calcium influx caused by membrane depolarization (14). High intracellular calcium further depletes energy stores by activation of energy-dependent ion exchanges that attempt to move calcium into mitochondria.
and mitochondria release calcium, causing an increase in intracellular Ca2+, in turn exacerbating calcium influx caused by membrane depolarization (14). High intracellular calcium further depletes energy stores by activation of energy-dependent ion exchanges that attempt to move calcium into mitochondria.
Strategies designed to limit free radical concentrations may also prove to be of benefit in patients with brain injury and cerebral edema. Increasing the levels of superoxide dismutase, a free radical scavenger, has been shown to limit infarction in animal stroke models (15). Newly developed modified steroid compounds, termed lazaroids, inactivate iron-related free radicals and have some protective effect in animal stroke models.
The interest in neuroprotection has led to dozens of clinical trials over the last decade, although no agent has yet been clearly demonstrated to improve neurological outcome following ischemic stroke. Various calcium antagonists, free radical scavengers and other novel agents have appeared much less effective in clinical trial than early work in animal models of stroke. There are many possible reasons to explain the early disappointing trial results, and also many reasons to be optimistic that the strategy will eventually prove beneficial (16).
Improving Cerebral Blood Flow
Induced Hypertension
Induced hypertension has been proposed as one strategy to increase cerebral blood flow in acute ischemic stroke. Although many patients have elevated blood pressures at presentation, others may benefit from pharmacologic elevation of pressure. The concept is similar to induced hypertension for ischemia related to vasospasm following subarachnoid hemorrhage. Induced hypertension in an animal model appeared to decrease lactate accumulation following middle cerebral artery occlusion, although edema formation may have been enhanced (17). Rordorf and coworkers (18) reported on a group of patients with acute stroke treated with induced hypertension and indicated that 10 of 30 patients had rapid and clinically apparent improvement in neurological deficits when the pressure was increased. The systolic pressure threshold for improvement ranged from 120 to 190 mm Hg, with a mean of 156 mm Hg. The treatment may be reasonable for those patients with relatively low pressures at presentation.
Thrombolysis
Acute revascularization with thrombolytic therapies has been demonstrated to improve outcome in stroke (19). The most commonly used strategy is intravenous systemic t-PA used within the first 3 hours after stroke symptom onset. Drug infusion frequently begins in the emergency department, but most patients are monitored and cared for in an ICU setting. An intraarterial strategy also has been pursued, and a recent randomized trial demonstrated improved outcome with a 6-hour treatment window (20). The intraarterial approach may be most useful with more proximal large vessel occlusions. A combination strategy is also under study, using an initial intravenous dose followed by angiography and intraarterial therapy as needed. Further clinical study will be needed to establish the optimal timing and route of administration of lytic therapy.
Stroke patients treated with thrombolytic therapy should remain in a monitored setting for at least 24 hours. Blood pressure should be carefully monitored and controlled as needed to maintain systolic pressures less than 185 mm Hg and diastolic pressures less than 110 mm Hg. Although one would not usually intervene at this pressure level, the risks of excessive hemorrhage lead to a more aggressive approach to blood pressure control in this group of patients. Serious systemic hemorrhages are uncommon, although they can be life threatening if not rapidly detected. Retroperitoneal hemorrhage often presents with lower abdominal pain, and must be treated with volume replacement. There is not a rapidly effective reversal of the coagulopathy
associated with thrombolytic agents, although some degree of hemostasis may be restored with the administration of cryoprecipitated clotting factors.
associated with thrombolytic agents, although some degree of hemostasis may be restored with the administration of cryoprecipitated clotting factors.
BRAIN EDEMA FOLLOWING LARGE HEMISPHERIC STROKE
Patients with large hemispheric stroke are at high risk for neurological deterioration and thus are frequently seen in the neurological intensive care unit (neuro-ICU). When the condition of a patient with a large hemispheric stroke worsens the initial evaluation should address both neurological and systemic factors that could cause such deterioration. The primary neurological causes of deterioration are cerebral edema, hemorrhage into the infarct and, rarely, recurrent stroke. Systemic causes include fever, infection, hypotension, hypoxia, and hypercarbia. Brain edema in patients with small strokes often has no or minimal impact on clinical status. On the other hand, edema following large hemispheric strokes can have devastating consequences. Considerable effort recently has been directed toward determining the best management of massive hemispheric swelling following ischemic stroke.
Infarction of the entire middle cerebral artery (MCA) territory is almost always caused by occlusion of the distal internal carotid artery or the proximal middle cerebral artery trunk. The presence of well-developed leptomeningeal collateral vessels may help limit infarct size. These strokes usually are caused by emboli from cardiac or arterial sources. About 10% to 20% of ischemic stroke patients suffer infarction of the complete MCA territory. Midline shift following MCA stroke begins during the first 3 days, peaks at 3 to 5 days, and subsides by 2 weeks.
Some patients with complete MCA territory stroke deteriorate dramatically over the next 24 to 72 hours and on repeat CT scanning demonstrate massive edema with severe midline shift and compression of the basal cisterns. This has been described as the “malignant MCA syndrome” with a reported historical mortality rate as high as 80% (21). More recent series suggest the mortality rate is closer to 50% (22).
There appears to be a subset of patients who develop very rapid and massive edema over the first day following stroke. This occurs frequently in patients who receive thrombolytics (23,24) and may be the result of reperfusion of a large area of already infarcted brain.
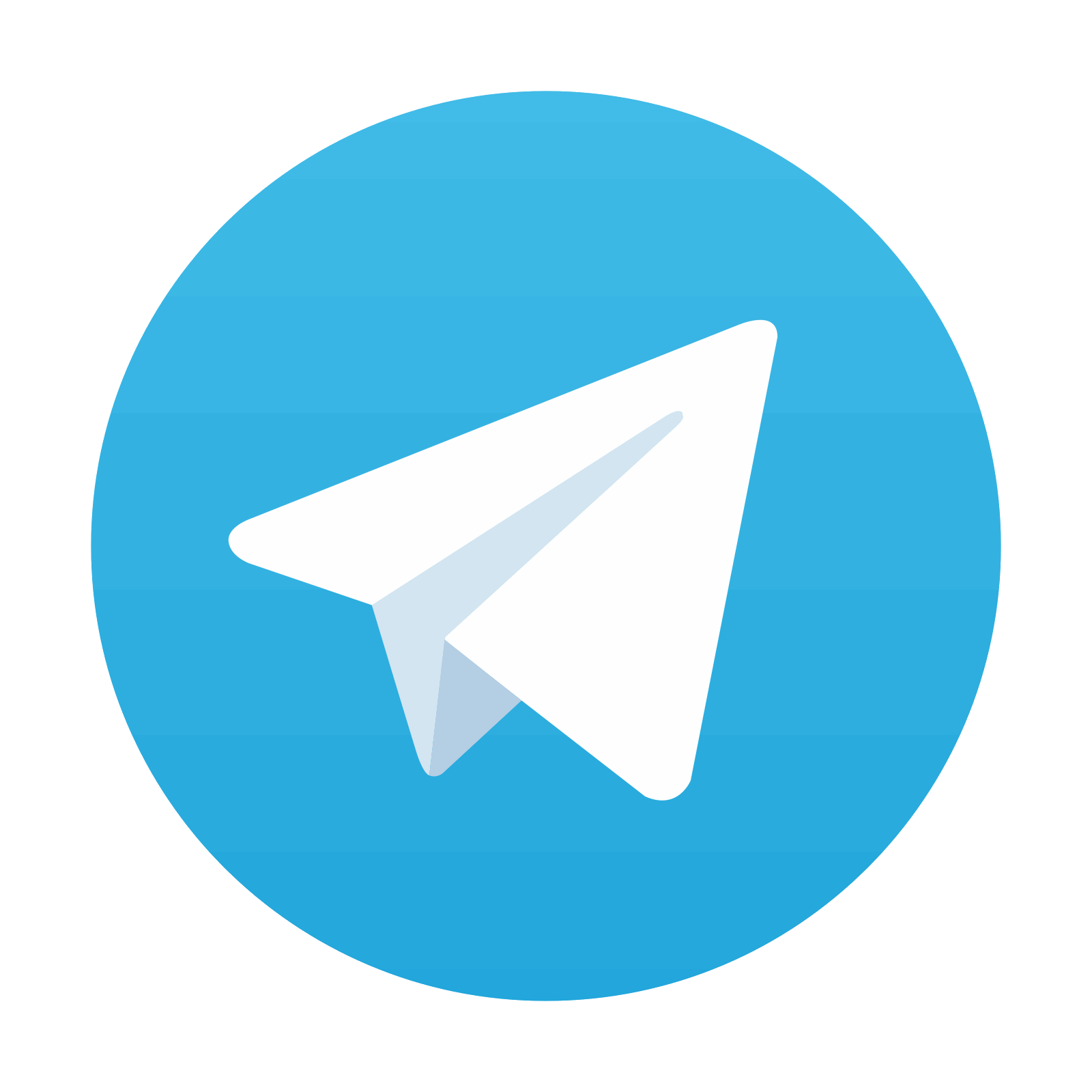
Stay updated, free articles. Join our Telegram channel

Full access? Get Clinical Tree
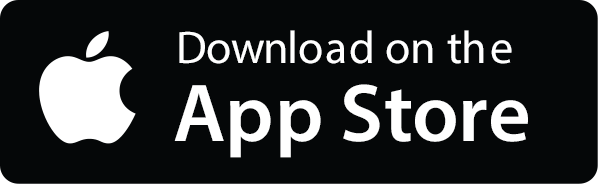
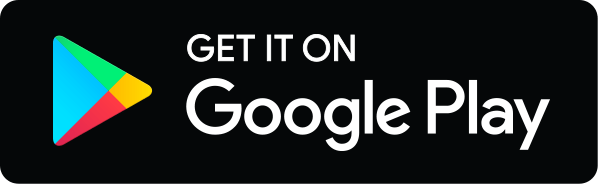