The editors and publisher would like to thank Drs. Lundy Campbell and Michael Gropper for contributing to this chapter in the previous edition of this work. It has served as the foundation for the current chapter.
From the late 20th century to the present, critical care medicine has evolved as a dynamic, multidisciplinary field focused on the care of patients with life-threatening diseases. Anesthesia providers can play an important role in the care of the critically ill patient both in the operating room and intensive care unit (ICU). A few key topics in critical care with which the practicing anesthesia provider should be familiar include respiratory failure, shock, renal failure, and management of pain and sedation.
Respiratory Failure
Respiratory failure remains a primary indication for admission to an ICU. The type of respiratory failure can be categorized based on the acuity of the process (e.g., acute vs. chronic) and the physiologic perturbation present (e.g., hypercapnia vs. hypoxemia). Such distinctions help to direct decision making for various treatment options. However, multiple processes can occur simultaneously. For example, a patient may have an acute and chronic respiratory failure with the presence of both hypoxemia and hypercapnia.
Hypoxemic respiratory failure generally occurs because of ventilation/perfusion ( <SPAN role=presentation tabIndex=0 id=MathJax-Element-1-Frame class=MathJax style="POSITION: relative" data-mathml='V˙/Q˙’>V˙/Q˙V˙/Q˙
V ˙ / Q ˙
) mismatch leading to a large alveolar-arterial (A-a) gradient. Causes include trauma, acute respiratory distress syndrome (ARDS), sepsis, pneumonia, pulmonary embolism, cardiogenic pulmonary edema, and obstructive lung disease. Other physiologic causes of hypoxemia include intrapulmonary shunt, hypoventilation, and increased O 2 extraction (also see Chapter 5 ).
Causes of hypercapnic respiratory failure include hypoventilation, as may occur from a drug intoxication or neuromuscular weakness, or increased dead space, which occurs with chronic obstructive pulmonary disease (COPD) or asthma. Hypercapnia may also be present in severe forms of an infiltrative pulmonary process, such as ARDS. Both hypercapnic and hypoxemic respiratory failure may require initiation of mechanical ventilator support.
Mechanical Ventilation
In modern ICUs, mechanical ventilation is performed entirely via positive-pressure ventilation. It may be accomplished through a noninvasive approach (via face mask or nasal mask) or an invasive approach (via endotracheal tube [ETT] or tracheostomy). The goals of mechanical ventilation include (1) decreasing the work of breathing; (2) improving oxygen delivery; (3) facilitating carbon dioxide removal; and (4) minimizing ventilator-associated lung injury. The settings for mechanical ventilation describe how the ventilator interacts with the patient ( Table 41.1 ).
Mode | Control | Limit | Cycle |
---|---|---|---|
AC | Volume | Volume | Volume |
Pressure | Pressure | Time | |
SIMV | Volume | Volume | Volume |
Pressure | Pressure | Time | |
PS | Pressure | Flow |
Modes
Assist Control
In assist control (AC) mode, the ventilator is set to deliver a minimum number of breaths per minute, while allowing the patient to initiate breaths as well. All mandatory and spontaneous breaths are fully supported to the same degree. So, if tidal volume is set at 500 mL, then all breaths (i.e., mandatory and spontaneous) will receive a tidal volume of 500 mL.
Synchronized Intermittent Mandatory Ventilation
With synchronized intermittent mandatory ventilation (SIMV), the ventilator attempts to synchronize the mandatory mechanical breaths with the patient’s spontaneous breaths in order to decrease ventilator dyssynchrony. If there are no spontaneous breaths within the preset time interval, then the ventilator will deliver the mandatory breath. The breaths in between the mandatory breaths are not fully supported, unlike the AC mode. For these nonmandatory breaths, the ventilator can be set to deliver pressure support (PS), as described next.
Pressure Support
PS mode is used only with spontaneously breathing patients, as all breaths are triggered by patient effort. The driving pressure (ΔP), positive end-expiratory pressure (PEEP), and fraction of inspired oxygen (F io 2 ) are the only variables set in this mode. Inspiratory flows are based on patient demand. The ventilator ends inspiration when the flow rate has decreased to a predetermined level (usually 25% of the peak flow rate). There is no backup respiratory rate in PS mode unless it is combined with SIMV.
Other Modes
The sophisticated microprocessors in current ventilators enable novel modes such as adaptive support ventilation, airway pressure release ventilation, and proportional assist ventilation. These modes offer potential physiologic benefits but have not been subject to large clinical trials with enough power to demonstrate improved mortality rate.
Limits
With AC or SIMV mode, the limit or control needs to be specified. With volume control (VC), a preset tidal volume is delivered during inspiration. With pressure control (PC), a preset inspiratory pressure is delivered by the ventilator.
Volume Control
Sample mechanical ventilation orders for AC and SIMV with VC are listed in Table 41.2 . The tidal volume, rate, PEEP, and F io 2 must be specified. The inspiratory flow rate is not typically part of a standard ventilator order set and is set by the respiratory therapist. A typical inspiratory flow rate is 60 L/min. By increasing inspiratory flow, the set tidal volume is delivered in a shorter time, which allows more time for exhalation. This strategy may be beneficial for an asthmatic patient in respiratory distress to increase expiratory time. The flow waveform in VC can be decelerating or constant (so-called square wave).
Example | Ventilator Orders Written | Additional Settings That Can Be Ordered | Explanation |
---|---|---|---|
Example 1: Assist control–volume control (AC-VC) | Mode AC/VC Rate 10 V t 500 mL PEEP 5 cm H 2 O F io 2 1.0 | Flow rate: typically 60 L/min Trigger: flow or pressure | Ventilator will deliver the preset tidal volume of 500 mL 10 times a minute; if the patient’s respiratory rate is greater than 10, each breath will also be 500 mL |
Example 2: Assist control–pressure control (AC-PC) | Mode AC/PC Rate 10 PIP 20 cm H 2 O PEEP 5 cm H 2 O F io 2 1.0 | I:E ratio: typically 1:2 Inspiratory time Trigger: flow or pressure | Ventilator will deliver 10 breaths per minute; each breath will reach a peak pressure of 20 cm H 2 O; if the patient’s respiratory rate is greater than 10, each breath will also reach peak pressure of 20 cm H 2 O |
Example 3: Synchronized intermittent mandatory ventilation–volume control (SIMV-VC) | Mode SIMV-VC Rate 10 V t 500 mL Pressure support 5 cm H 2 O PEEP 5 cm H 2 O F io 2 0.5 | Flow rate: typically 60 L/min Trigger: flow or pressure (this applies to all the breaths, SIMV, or pressure support) | Ventilator will deliver 10 breaths per minute with tidal volume 500 mL; if the patient’s respiratory rate is greater than 10, those nonmandatory breaths will receive inspiratory pressure support to peak pressure 5 cm H 2 O above the PEEP of 5 cm H 2 O |
Example 4: Pressure support ventilation (PSV) | Mode PSV Driving pressure 8 cm H 2 OPEEP 5 cm H 2 O F io 2 0.5 | Trigger: flow or pressure | Patient must be breathing spontaneously; each breath will receive inspiratory pressure support to peak pressure 8 cm H 2 O above the PEEP of 5 cm H 2 O |
Pressure Control
In AC or SIMV mode with PC, a driving pressure must be specified. Additionally, an inspiratory time or inspiratory-expiratory time (I:E) ratio is set. The peak flows in PC are variable and based on demand. By default, the flow waveform must be decelerating in order to maintain a constant peak inspiratory pressure. Tidal volumes are not guaranteed and if lung compliance changes quickly, vigilance is necessary to make sure minute ventilation does not drop rapidly.
Dual Control
The choice between VC or PC is not supported by definitive evidence. Modern ventilators can combine the features of both, targeting specified tidal volumes, but delivering each breath in a PC mode with decelerating flows. If pulmonary compliance changes, then the ventilator automatically adjusts the pressure gradually over a few breaths to maintain the targeted tidal volumes. Many proprietary names exist, such as pressure control ventilation-volume guaranteed, pressure-regulated volume control, or volume control plus .
Cycle
The cycle determines how the ventilator switches from inspiration to expiration. For AC-VC or SIMV-VC modes, volume determines the ventilator cycle. Inspiration is complete when the tidal volume is delivered (see Table 41.1 ). For AC-PC or SIMV-PC, inspiration is complete when the inspiratory time has ended. With PS mode, a decrease in the inspiratory flow rate determines the end of the inspiratory cycle. Knowledge of how the ventilator cycles can allow better understanding of patient/ventilator dyssynchrony. For example, a patient in respiratory distress receiving mechanical ventilation in the AC-VC mode may find the set tidal volume too small and “double stack” (i.e., take a second breath during the start of the ventilator’s exhalation phase). Or, a patient receiving AC-PC ventilation may begin exhalation prior to the end of the set inspiratory time.
Other Settings
Positive End-Expiratory Pressure
PEEP is constant positive airway pressure that is applied throughout the respiratory cycle. PEEP is generated by a pressure relief valve on the expiratory limb of the ventilator circuit. The use of PEEP leads to an increased mean airway pressure, which decreases atelectasis and improves oxygenation. PEEP also increases functional residual capacity and can improve pulmonary compliance.
If PEEP is too large, alveolar overdistention can occur, which may lead to barotrauma. Excessive PEEP can also reduce preload and cause hypotension. Auto-PEEP occurs when a buildup of end-expiratory pressure results from insufficient exhalation time. Emergent treatment of auto-PEEP entails disconnecting the patient from the ventilator to release the PEEP. Treatment of auto-PEEP requires increasing expiratory time (i.e., changing the ratio of the duration of inspiration to the duration of expiration, or I:E ratio).
Trigger
The trigger refers to the manner by which the ventilator detects patient inspiration and delivers positive pressure in synchrony with the patient’s efforts. The trigger variable, which can be based on flow or pressure, is not part of a typical ventilator order set and is often managed by respiratory therapists. Usual triggers are a change in flow of 2 L/min or a change in pressure of 2 cm H 2 O. Smaller or larger triggers can be set based on the clinical situation. For example, patients with bronchopleural fistulas may constantly trigger mechanical ventilation breaths if the flow trigger is too sensitive.
Noninvasive Positive-Pressure Ventilation
Noninvasive positive-pressure ventilation (NIPPV) delivers positive-pressure breaths via a face mask, nasal pillows, or helmet without an ETT present. For patients with COPD and acute hypercapnic respiratory failure (AHRF), appropriate use of NIPPV can reduce mortality rate, avoid endotracheal intubation, improve dyspnea, and reduce hospital length of stay. Other established indications for NIPPV include acute cardiogenic pulmonary edema, postoperative respiratory failure, and hypoxic respiratory failure in immunocompromised patients (e.g., organ and bone marrow transplant recipients).
The data for NIPPV are often impressive, but the patients chosen for these studies were judiciously selected in trials with close clinical observation. NIPPV is most beneficial in patients who have a potentially rapidly reversible pulmonary process that requires some ventilator support. Delays in endotracheal intubation can lead to an emergent event that is more prone to complications. Contraindications for using NIPPV are listed in Box 41.1 . The most commonly used ventilator mode for NIPPV is PS.
- •
Impaired neurologic state (coma, seizures, encephalopathy)
- •
Respiratory arrest or upper airway obstruction
- •
Shock or severe cardiovascular instability
- •
Severe upper gastrointestinal bleeding
- •
Recent gastroesophageal surgery
- •
Vomiting
- •
Excessive airway secretions
- •
Facial lesions that prevent proper fit of nasal or facial masks
High-Flow Nasal Cannula
Use of high-flow nasal cannula (HFNC) has emerged as an alternative to NIPPV. HFNC uses heated and humidified oxygen that is delivered at high flow rates through nasal cannula. This delivery system provides a small amount of positive airway pressure and reduces dead space by flushing expired carbon dioxide from the upper airways. Most patients find HFNC to be more comfortable and easier to tolerate than NIPPV via a face mask. A 2015 multicenter trial in patients with acute hypoxemic, nonhypercarbic respiratory failure showed that high-flow oxygen therapy, as compared with standard oxygen therapy or noninvasive ventilation, resulted in reduced mortality rate in the ICU and at 90 days, although there was no difference in the rate of tracheal intubation.
Weaning From Mechanical Ventilation and Tracheal Extubation
Weaning may account for more than 40% of the patient’s time on mechanical ventilation depending on the definition of when weaning commences. To decrease the risk of ventilator-associated pneumonia (VAP), patients should be weaned from mechanical ventilation as soon as they have recovered from the process that originally required mechanical ventilator support.
The average rate of failed tracheal extubation (i.e., inadequate ventilation following extubation of the trachea) in surgical ICUs is 5% to 8%, whereas in medical and neurologic ICUs the rate is 17%. Although many criteria are listed in the following section, no one algorithm can accurately predict successful tracheal extubation. A cautiously applied aggressive approach to weaning from mechanical ventilation and tracheal extubation results in fewer ICU-related complications, although definitive data from randomized trials are lacking.
Criteria for Weaning Trial
A-a Gradient
The patient should have adequate oxygenation, usually defined as Pa o 2 /F io 2 more than 150 mm Hg with PEEP less than 8 cm H 2 O. This amount of oxygen is chosen because this level can be reliably delivered via face mask or nasal cannula. An oxygen requirement greater than this denotes that the patient still has a large shunt fraction and the underlying pulmonary process may not have resolved adequately. In the end, these criteria are just guidelines. The final decision regarding what is an appropriate A-a gradient is often based on clinical judgment and experience.
Respiratory Mechanics
Rapid Shallow Breathing Index
Rapid shallow breathing index (RSBI) is the ratio of respiratory rate (breaths/min) to tidal volume (in liters). This index is the most extensively studied and commonly used weaning predictor. A RSBI less than 105 breaths/min/L (i.e., positive RSBI) is associated with weaning success, but a negative RSBI (RSBI more than 105 breaths/min/L) is likely better at identifying patients who will fail than a positive RSBI is at identifying patients who can succeed.
Maximum Inspiratory Force
Patients must have the respiratory muscle strength to generate an adequate tidal volume. One attempt to measure this is via the maximum inspiratory force (MIF). For weaning, a MIF of at least −20 cm H 2 O is preferable. A normal MIF indicates little or no increase in the probability of weaning success, but a small MIF predicts a small increase in the probability of weaning failure. One reason for the poor predictive ability of the MIF is the challenge of obtaining an accurate measurement in a spontaneously breathing patient. In many ICUs, MIF is not routinely measured prior to weaning from ventilation. However, if a patient is not progressing in the weaning process, measurement of a MIF may suggest a cause such as muscle weakness or deconditioning.
Other Criteria
Other respiratory criteria may impact success of weaning from ventilation, including the nature and amount of airway secretions and the ability to clear secretions, which involves the gag reflex and cough strength. The presence of upper airway edema may promote airway obstruction and hypoxemia after tracheal extubation. The cuff leak test is one method to assess for airway edema. The ETT cuff is deflated and positive pressure is delivered through the ETT until an air leak is heard. A leak pressure of less than 10 cm H 2 O suggests the absence of airway edema. In contrast, a leak pressure greater than 20 cm H 2 O may indicate significant airway edema and should be considered prior to the decision for tracheal extubation.
Other patient factors that impact weaning include mental status and hemodynamics. Patients should have an adequate level of consciousness to protect their airway from aspiration of gastric contents. In addition, patients should be hemodynamically stable, because discontinuation of positive-pressure ventilation can lead to increased work of breathing and alter left ventricular preload and afterload.
Weaning Strategies
Regardless of the weaning strategy used in ICUs, early identification of patients who are able to breathe spontaneously results in better outcomes. A common strategy is for the mechanically ventilated patient to undergo a daily assessment of readiness. If the patient is deemed ready, a spontaneous breathing trial (SBT) is performed. If the factors described previously (respiratory mechanics, mental status, hemodynamics) remain adequate throughout the SBT, a decision for tracheal extubation can be made. Protocol-based weaning by nurses and respiratory therapists allows more rapid tracheal extubation compared to physician-directed weaning.
The SBT can be conducted with different ventilation modes, including PS ventilation or T-piece trial. There is no definitive evidence of a superior mode associated with more frequent weaning success, less need for tracheal reintubation, or lower ICU fatality. However, for an individual patient, a specific mode may have clinical advantages. For example, in patients with heart failure and reduced cardiac ejection fraction, the change from positive-pressure ventilation to negative-pressure ventilation can increase left ventricular afterload and worsen cardiovascular strain. This patient may benefit from T-piece trial for the SBT, because even low levels of positive pressure and PEEP may provide afterload reduction. If the patient does not develop signs of pulmonary edema during the T-piece trial, the decision for tracheal extubation can proceed.
The optimal duration of an SBT is unknown, but most range from 30 minutes to 2 hours. Longer periods may be required for patients with chronic respiratory failure whose tracheas have been intubated for an extended duration or for patients who fail their initial SBT. In select patients, weaning strategies that include NIPPV can reduce the rate of mortality, VAP, and weaning failure without increasing the risk of tracheal reintubation. This approach may be considered in patients who do not have difficult to manage airways, excessive secretions, or an impaired mental status and should be coupled with an early decision regarding tracheal reintubation if the patient remains tachypneic or in distress. Tracheal reintubation, especially if delayed, is associated with increased mortality rate, longer hospital stay, and lower likelihood of returning home.
Automated closed-loop systems (e.g., SmartCare/PS, adaptive support ventilation, proportional assist ventilation, volume support ventilation) were developed to adapt ventilation in response to real-time changes with the patient’s respiratory mechanics. A recent meta-analysis showed that closed-loop systems reduced the duration of ventilation and ICU length of stay in mixed (combined medical-surgical ICUs) or medical ICU patients; however, more randomized controlled trials are needed for surgical ICU patients and patients using other automated systems. Currently, this area of research is in its infancy.
Acute Respiratory Distress Syndrome
ARDS, characterized as a diffuse, inflammatory injury of the lung, results in the development of noncardiogenic pulmonary edema with resultant <SPAN role=presentation tabIndex=0 id=MathJax-Element-2-Frame class=MathJax style="POSITION: relative" data-mathml='V˙/Q˙’>V˙/Q˙V˙/Q˙
V ˙ / Q ˙
mismatch, hypoxemia, and decreased pulmonary compliance. ARDS typically follows an inciting event that can lead to direct or indirect lung injury ( Table 41.3 ). Although the underlying cause of lung injury may predict outcome, patient-specific factors such as age, immunocompromised status, and organ dysfunction are stronger predictors for survival. In some patients, ARDS resolves following an acute phase but others experience a chronic alveolitis leading to pulmonary fibrosis. Such patients often experience continued hypoxemia, increased physiologic dead space, and decreased compliance with chronic ventilator dependence.
Causes of Direct Lung Injury | Causes of Indirect Lung Injury |
---|---|
Pneumonia | Sepsis |
Aspiration of stomach contents | Severe trauma |
Pulmonary contusion | Cardiopulmonary bypass |
Reperfusion pulmonary edema | Drug overdose |
Amniotic fluid embolus | Acute pancreatitis |
Inhalational injury | Near-drowning, transfusion-related acute lung injury |
Although classically defined by an increased A-a gradient in the setting of diffuse bilateral noncardiogenic pulmonary infiltrates, the clinical definition of ARDS continues to evolve—most recently with the Berlin criteria ( Table 41.4 ). In this new definition, the clinical distinction between ARDS and acute lung injury disappears and is replaced by categories of severity (i.e., mild, moderate, and severe). Additionally, the requirement for pulmonary artery occlusion pressure (PAOP) measurement no longer exists. In the absence of a known cardiac event, objective data such as echocardiography are required to rule out cardiogenic pulmonary edema as the cause of bilateral infiltrates.
AECC Definition | Berlin Definition | |
---|---|---|
Timing | Acute onset | Within 1 week of a known clinical insult or new or worsening respiratory symptoms |
Oxygenation | ALI : PaO 2 /FiO 2 ≤ 300 mm Hg ARDS : PaO 2 /FiO 2 ≤ 200 mm Hg | Mild : 200 mm Hg < PaO 2 /FiO 2 ≤ 300 mm Hg with PEEP or CPAP ≥ 5 cmH 2 O Moderate : 100 mm Hg < PaO 2 /FiO 2 ≤ 200 mm Hg with PEEP ≥ 5 cmH 2 O Severe : PaO 2 /FiO 2 ≤ 100 mm Hg with PEEP ≥ 5 cmH 2 O |
Chest radiograph | Bilateral infiltrates | Bilateral opacities not fully explained by effusions, lobar/lung collapse, or nodules |
Edema | PAWP ≤ 18 mm Hg when measured or no clinical evidence of left atrial hypertension | Respiratory failure not fully explained by cardiac failure or fluid overload |
Risk factor | Not included in definition | If no risk factor for lung injury identified, then need objective assessment such as echocardiography to exclude hydrostatic edema |
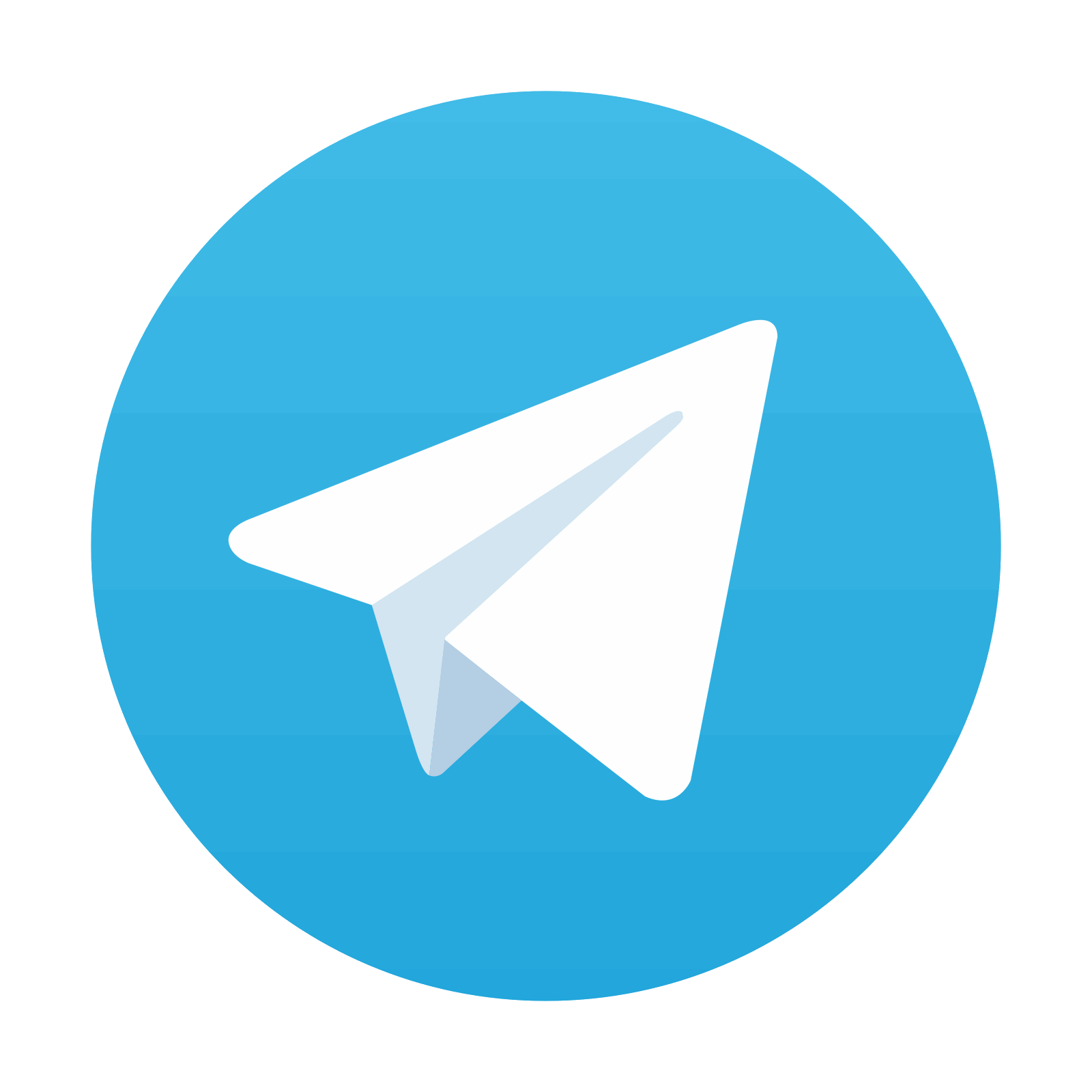
Stay updated, free articles. Join our Telegram channel

Full access? Get Clinical Tree
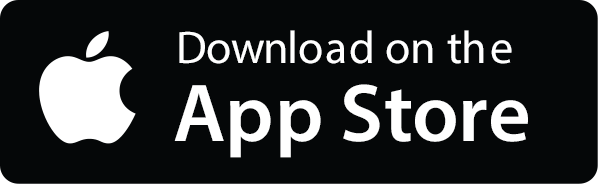
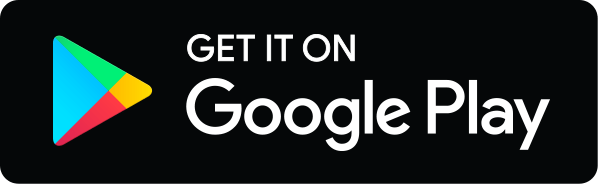
