1.Compassion
2. Communication (with patient and family)
3. Consideration (to patients, relatives, and colleagues) and avoidance of conflict
4. Comfort: prevention of suffering
5. Carefulness (avoidance of injury)
6. Consistency
7. Closure (ethics and withdrawal of care)
Patients are admitted to intensive care unit usually with one or more of the following problems: cardiac problems (postsurgical or hemodynamic insufficiency), respiratory problems (postsurgical or failure), neurological issues (postsurgical neurotrauma), or abnormalities of fluid and electrolytes and sepsis.
A well-structured critical care unit is essential for providing care and treatment to critically ill patients. An intensive care unit is a place that has a very high nurse to patient ratio, where patients are usually invasively monitored, and life-sustaining therapies are utilized (mechanical and pharmacological). Therefore, proper functioning of a critical care unit consists of teamwork, evidence-based practice and cost-effectiveness, and very strong pharmacy and infectious disease backup. This chapter discusses various concepts of critical care medicine and care of critically ill patients.
Shock
Shock is defined as acute circulatory failure leading to inadequate tissue perfusion and end organ injury. There is a drop in blood pressure with accompanying hemodynamic changes. Blood pressure is a factor of cardiac output and systemic vascular resistance, and cardiac output, in turn, is a factor of heart rate and stroke volume. The three factors heart rate, stroke volume, and peripheral resistance exist in equilibrium. Normally, if there is disruption of one of them, then the other two components compensate. Therefore, hypotension is an indication of an abnormality of heart rate, stroke volume, or peripheral resistance and a failure of these to compensate each other.
Progression of Shock
Shock typically progresses through four stages as described below. The pathophysiology of shock is depicted in Fig. 40.1.


Fig. 40.1
Pathophysiology of shock
Stage I (Initial)
Hypoperfusion of the tissues leads to hypoxia (lack of oxygen), which leads to anaerobic metabolism promoting the formation of lactic acid. Additionally, the lack of oxygen slows down the Krebs cycle causing more pyruvate to be available, which is converted to lactate by the cells.
Stage II (Compensatory)
In this stage the body tries to compensate the hypoxia and the acidosis through various physiological mechanisms. To rid the body of the excess CO2 (acidosis), the person hyperventilates. The arterial baroreceptors sense the low blood pressure and cause increased sympathetic response and catecholamines to be released. The increased sympathetic response causes vasoconstriction and an increase in the heart rate, contractility, and cardiac output leading to an increase in the blood pressure. Blood is preferentially diverted to the arterial system and the vital organs (autotransfusion).
Additionally, because of reduced renal blood flow, the renin-angiotensin axis is activated causing release of the angiotensin, aldosterone, and antidiuretic hormone (ADH). These hormones act on the kidneys to conserve fluid (vasoconstriction, salt, and water retention). All these hormones preferentially act to divert blood to the heart, the lungs, and the brain. Conservation of fluid leads to a drop in the urine output.
Stage III (Progressive)
If the shock is not corrected, the compensatory mechanisms begin to fail, and the progressive stage ensues. As hypoperfusion and anaerobic metabolism continue, the acidosis worsens. Due to acidosis, the arteriolar smooth muscle dilates to cause pooling of blood in the capillaries. This increases local hydrostatic pressure causing exudation of fluid and protein into the surrounding tissues. Due to loss of fluid, the blood becomes more viscous causing sludging of the microcirculation and accumulation of toxic substances. The prolonged vasoconstriction also causes the vital organs to be compromised due to reduced perfusion.
Stage IV (Refractory)
Because of lack of oxygen, cellular ATP supply is irreversibly depleted. Adenosine perfuses out into the surrounding tissues and is not available to undergo phosphorylation into ATP. The vital organs begin to fail, and eventually cell death and brain damage ensues.
Causes and Classification of Shock (Fig. 40.2)

Fig. 40.2
Classification of shock
Shock can be classified as:
Cardiogenic, malfunction of the pump (Fig. 40.3)
Fig. 40.3
Cardiovascular system showing the pump, the distribution system, and the fluid compartments
Distributive or vasodilatory, malfunction of the vasculature (tubing)
Hypovolemic, problems with loss of fluid
Cardiogenic shock results from failure of the cardiac muscle (ischemia, inflammation, fibrosis-myocarditis, and volume overload) and cardiac outflow obstruction (pulmonary embolism and aortic stenosis) or inflow obstruction (cardiac tamponade). Distributive shock occurs due to loss of sympathetic tone and release of vasoactive metabolites causing a decrease in peripheral vascular resistance, as in sepsis or anaphylaxis. The abnormal vasodilatation leads to a relative hypovolemia; the amount of fluid is the same, but the tubing becomes larger. There is redistribution of blood away from the vital organs to the periphery. Finally, hypovolemia shock may be due to dehydration, hemorrhage/exogenous fluid loss, or fluid redistribution (third space loss). Tables 40.2, 40.3, and 40.4 list the clinical signs of various types of shock.
Table 40.2
Clinical signs of cardiogenic shock
• BP—hypotension because of decreased cardiac output |
• Heart rate—rapid, weak, thready pulse |
• Respiratory rate—rapid and deep respirations (hyperventilation) due to sympathetic nervous system stimulation and acidosis |
• Skin—cool, clammy, and mottled skin due to vasoconstriction and hypoperfusion |
• Mental status—anxiety, restlessness, altered mental state due to decreased cerebral perfusion |
• Urine output—oliguria due to insufficient renal perfusion |
• Pulmonary edema—due to fluid backup in the lungs |
• Jugular veins—distended, due to increased jugular venous pressure |
• Hepatic congestion due to insufficient pumping of the heart |
Table 40.3
Clinical signs of distributive (vasodilatory) shock
• Initial—BP normal, cardiac output maintained (by increasing stroke volume and heart rate) |
• Late—metabolic acidosis and worsening myocardial perfusion cause a decrease in cardiac output leading to cardiac failure |
• Heart rate—rapid |
• Skin—initially warm, later cold and poorly perfused |
Table 40.4
Clinical signs of hypovolemic shock
Stage | Blood loss | Clinical signs |
---|---|---|
Stage I | 15 % (750 ml) | • BP—maintained • Heart rate—normal • Respiratory rate—normal • Skin pallor—normal • Mental status—normal • Urine output—normal |
Stage II | 15–30 % (750–1,500 ml) | • BP—systolic maintained, diastolic increased (narrow pulse pressure) • Heart rate >100 bpm (tachycardia) • Respiratory rate—increased • Skin—pale, cold, clammy • Mental status—anxious, restless • Urine output—decreased (≤0.5 ml/kg/h) |
Stage III | 30–40 % (1,500–2,000 ml) | • BP—systolic BP falls to 100 mmHg or less • Heart rate >120 bpm (marked tachycardia) • Respiratory rate >30 bpm (marked tachypnea) • Skin—pale, cold, sweating • Mental status—confusion, anxiety, agitation • Urine output—decreased further (<0.5 ml/kg/h) |
Stage IV | >40 % (>2,000 ml) | • BP—significantly decreased systolic blood pressure of 70 mmHg or less • Heart rate >140 bpm (extreme tachycardia), weak, thready pulse • Respiratory rate—marked tachypnea • Skin—extremely pale, cool, sweaty • Mental status—decreased level of consciousness, lethargy, coma • Urine output—negligible |
Monitoring in Shock
The following monitoring modalities are used in patients with shock:
Blood tests: CBC, chemistry, and crossmatch
Standard monitors and arterial line
Central venous line and pressure
Arterial blood gas analysis
Urine output measurements (Foley catheter)
Pulmonary artery catheter, if cardiac dysfunction
Echocardiography
Central venous pressure (CVP), when measured, should be measured as a trend. The CVP measures right heart filling pressures, which are representative of systemic blood volume. It should be remembered that conditions such as complete heart block, atrial fibrillation, tricuspid stenosis, or regurgitation lead to an inaccurate CVP reading, which can be diagnosed by the CVP tracing itself. Hemodynamic parameters in various shock states are listed in Table 40.5.
Table 40.5
Hemodynamic parameters in various shock states
Type of shock | HR | SV and CO | CVP | PCWP | SVR |
---|---|---|---|---|---|
Cardiogenic | |||||
Pump failure | ↑ | ↓ | ↓ | ↓ | ↑ |
Volume overload | ↑ | ↓ | ↑ | ↑ | ↑ |
Inflow/outflow obstruction | ↑ | ↓ | ↑ | ↑ | ↑ |
Heart block | ↓ | ↑ (CO ↓) | ↑ | ↑ | |
Vasodilatory | |||||
Sepsis | ↑ | ↓ (CO ↑) | ↓ | ↓ | ↓ |
Anaphylaxis and spinal shock | ↑ | N (CO ↑) | ↓ | ↓ | ↓ |
Hypovolemic | ↑ | ↓ | ↓ | ↓ | ↑ |
Pulmonary artery catheters (PAC) are nowadays not commonly used to guide fluid resuscitation. If there is a strong suspicion that right-sided filling pressures are unrepresentative of left-sided pressures (refractory cardiogenic shock, myocardial contusion, myocardial ischemia due to sepsis), then a PAC may be inserted. A PAC measures the relationship between the pulmonary circulation and the left heart. When using a PAC, the wedge pressure, end diastolic volume, stroke volume, CVP, and mixed venous oxygen saturation measurements are used to guide fluid resuscitation.
Management of Shock
The first step is to confirm that the patient is in shock. Blood pressure should be measured with an adequate size cuff or commonly via an arterial line. Often the patient is sedated and would not be able to communicate fully, making mental status changes difficult to assess. If the patient is urinating adequately, it can be usually assumed that the tissues are being perfused adequately. However, if the patient is on diuretics, then measuring urine output is not a reliable sign for tissue perfusion. Presence of lactic acidosis (negative base excess) is another sign of tissue hypoperfusion.
The end point of management of shock is achieved by increasing the blood pressure (Fig. 40.4). Usually this end point is an awake patient with an adequate urine output. Fluid and vasopressors (Table 40.6) should always be targeted to the pressure that is normal for that particular patient. If there is concern about a patient’s intravascular volume status, a central line is placed and the CVP is measured. Patients are then volume overloaded to a target CVP, which is usually a CVP of 8–10 mmHg in non-ventilated patients and 12–16 mmHg in patients on positive pressure ventilation.


Fig. 40.4
Management strategies in shock
Table 40.6
Choice of vasopressor in shock
Cardiogenic shock—dobutamine, norepinephrine |
Septic shock—norepinephrine |
Anesthesia-related or spinal shock (with absence of bradycardia)—phenylephrine |
Anaphylactic shock—epinephrine |
Hypotension with bradycardia—epinephrine |
Shock of unknown etiology—epinephrine or dopamine |
If a cardiac abnormality/dysfunction is suspected, then ECG and echocardiography (ejection fraction, wall motion abnormalities) are performed. If there is suspicion of significantly different muscle physiology/filling pressures between the right and left side of the heart, then a pulmonary artery catheter (PAC) may be placed. The PAC is used to measure stroke volume (cardiac output), left ventricular end-diastolic volume, wedge pressure, and mixed venous oxygen saturation.
The following interventions are carried out for a patient in shock:
Large bore intravenous access.
Oxygen supplementation and endotracheal intubation as necessary.
Surgical repair of hemorrhagic sites.
Fluid therapy: crystalloids, colloids, packed red blood cells, fresh frozen plasma, and platelets.
Supportive care.
Cardiogenic shock—goals are to increase cardiac output, decrease systemic vascular resistance, and decrease myocardial work and O2 consumption. Cardiogenic shock is treated with fluid therapy and vasopressors, as needed. Dobutamine is preferred for inotropy as it increases contractility and heart rate and mildly decreases afterload. To decrease afterload effectively, other vasodilators (nitroglycerine, nitroprusside, nicardipine) may be used. Additional interventions in cardiogenic shock include diuretics (used cautiously), antiarrhythmic agents, angioplasty for an ischemic event, intra-aortic balloon pump to improve coronary circulation, or ventricular assist device for severe cardiac dysfunction.
Distributive shock—fluids, vasopressors, anaphylaxis (epinephrine), and sepsis (see below).
Hypovolemic shock—aggressive fluid therapy ± vasopressor support (dopamine, norepinephrine).
Other Causes of Hypotension in the ICU
Excessive Sedation
Many patients in the intensive care are sedated. Commonly used sedative agents include propofol, midazolam, and opioids (morphine, fentanyl). These agents, especially when used in combination, may lead to hypotension. Their effects may be decreased by decreasing their dose and/or treating with a vasoconstrictor (phenylephrine).
Bradycardia
The normal compensatory response to hypotension is tachycardia. Therefore, hypotension with bradycardia is pathological. Bradycardia may be due to excessive beta-blockade, which reverses after stopping the beta-blockers, or rhythm disturbances, which may require pacing.
Tachycardia
Sinus tachycardia, as mentioned above, occurs as part of the compensatory mechanism for low blood pressure in a vasodilated state. Treating the cause (hypovolemia, sepsis, pain) results in the resolution of the tachycardia. Therefore, no intervention to treat the tachycardia is required per se. However, in patients with cardiac disease, the increase in myocardial work due to the tachycardia may cause ischemia. Therefore, heart rate control may be achieved in such patients by using beta-blockers. Additionally, patients with hypotension due to tachyarrhythmias (atrial fibrillation or flutter) may have to be cardioverted, with correction of electrolyte disturbances (magnesium, potassium) and administrations of drugs (diltiazem, beta-blockers, or amiodarone).
Sepsis
In the United States, sepsis affects approximately 3/1,000 people, and severe sepsis contributes to more than 200,000 deaths per year. Sepsis causes millions of deaths globally each year. Sepsis occurs in 1–2 % of all hospitalizations and accounts for as much as 25 % of ICU bed utilization. Sepsis is characterized by a whole-body inflammation, usually in response to an infection. It consists of a systemic inflammatory response syndrome and can be defined as:
1.
Infection: presence of infection leading to a systemic inflammatory response syndrome (SIRS) manifested by:
Temperature >38 °C or <36 °C
Heart rate >90 beats/min
Respiratory rate >20 breaths/min or PaCO2 <32 mmHg
WBC count >12,000/mm3, <4,000/mm3, or >10 % immature (band) forms
2.
Sepsis: infection confirmed or suspected, plus two of the above SIRS criteria
3.
Severe sepsis: sepsis, plus one organ dysfunction
4.
Septic shock: sepsis, plus hypotension despite fluid resuscitation
Pathophysiology
Sepsis is most commonly caused by bacteria, but can also be caused by fungi, viruses, and parasites in the blood, urinary tract, lungs, skin, or other tissues. No infective source is found in about one-third of the cases. Usually patients have an initial infection, called as the first hit. The inflammation may then persist, or the patient may have subsequent hits of infection. Persistent presence of inflammation then leads to the sepsis syndrome.
Unlike patients in cardiogenic shock, who are cold and hypotensive, septic patients are hot and hypotensive. This is because of the loss of sympathetic tone and vasodilation due to the accumulation of metabolites and increased amounts of nitric oxide. The vasodilation leads to a relative hypovolemia (normal amount of fluid but larger tubing). The stroke volume is reduced leading to myocardial ischemia and cardiac failure. Initially, the cardiac output may be increased due to an increase in heart rate. Eventually, due to persistent vasodilation of small blood vessels, there is widespread capillary leak and microcirculatory failure (Fig. 40.5). Additionally, the coagulation cascade is stimulated causing widespread deposition of fibrin and thrombi, which lead to stagnation of the microcirculation.


Fig. 40.5
Pathophysiology of sepsis
Common symptoms of sepsis include those related to a specific infection, accompanied by hypotension (decreased systemic vascular resistance); high fever or hypothermia; hot, flushed skin; tachycardia; hyperventilation; altered mental status; peripheral swelling; and disturbances in coagulation. Sepsis if not controlled leads to multiple organ dysfunction (MOD) and finally death (Table 40.7).
Table 40.7
End-organ dysfunction in sepsis
Lungs | Acute lung injury (PaO2/FiO2 < 300), ARDS (PaO2/FiO2 < 200) |
Brain | Encephalopathy, delirium, ischemia, microabscess, microthrombi |
Liver | Lactic acidosis, coagulopathy, disruption of metabolic processes, disruption of protein synthesis, elevation of bilirubin (jaundice) and liver enzymes, hypoglycemia |
Kidney | Acute kidney injury, oliguria/anuria, electrolyte abnormalities, volume overload |
Heart | Hypotension, heart failure, nonischemic troponin leak |
Hematologic | Thrombocytopenia, elevation of PT and INR, disseminated intravascular coagulation, leukocytosis/leukopenia |
GI tract | Stress ulcers |
Adrenal | Cortisol deficiency, however, steroid therapy in sepsis is controversial |
Management of Sepsis
Sepsis is usually managed as follows:
Intravenous fluids: target CVP of 8–12 mmHg, urine output >0.5 ml/kg/h, mixed venous oxygen saturation >70 %.
Antibiotics and antifungal medications.
Vasopressors to maintain blood pressure (epinephrine, norepinephrine, vasopressin), systolic blood pressure >90 mmHg, or mean arterial pressure >65 mmHg.
Mechanical ventilation.
Dialysis to support kidney function.
Central venous catheter, arterial catheter, or a pulmonary catheter may be placed to measure hemodynamic variables, such as cardiac output, mixed venous oxygen saturation, or stroke volume variation.
Preventive measures for deep vein thrombosis.
Prevention of stress ulcers and pressure ulcers.
Control of blood sugar levels with insulin (targeting stress hyperglycemia), although hypoglycemia is seen with severe liver dysfunction.
Mechanical Ventilation
Many patients in the ICU are mechanically ventilated. Therefore, it is of utmost important to be familiar with the various modalities of mechanical ventilation in order to provide optimal care to patients. In addition, weaning off patients early from mechanical ventilation decreases the incidence of nosocomial infections. Mechanical ventilation is provided for three main reasons:
Impaired oxygenation (PaO2 < 50 mmHg)—reduced diffusion capacity of O2 and V/Q mismatch (dead space ventilation or shunt). Examples—acute respiratory distress syndrome (ARDS), sepsis, pneumonia, pulmonary edema, pulmonary fibrosis, and cyanide or carbon monoxide poisoning
Impaired CO2 elimination (PaCO2 > 50 mmHg)—neuromuscular disorders (myasthenia gravis), loss of ventilator drive (brain injury, stroke), spinal cord injury, bronchospasm, pneumothorax, airway foreign body, and COPD
Airway protection—airway edema, loss of gag reflex, mental status changes (GCS <8), and inhalational burn injury
Noninvasive Positive Pressure Ventilation
Noninvasive positive pressure ventilation (NIPPV) is delivered without the use of an endotracheal tube. Positive pressure is delivered by a face mask covering the mouth and/or the nose. The face mask is held firmly in place by a tight fitting strap. Commonly used methods include continuous positive pressure airway (CPAP) and bi-level positive airway pressure (BiPAP).
CPAP
Continuous positive airway pressure is applied during both inspiration and expiration, in a spontaneously breathing patient. Oxygenation is improved due to recruitment of collapsed alveoli. In addition, CPAP helps to maintain the patency of the airway.
BiPAP
Continuous positive airway pressure is applied during both inspiration and expiration, but 2 airway pressure settings are used, higher pressure during inspiration and lower pressure during expiration.
Advantages and Uses
NIPPV allows ventilation for short periods, such as during sleep or immediately after discontinuation of endotracheal mechanical ventilation. Using NIPPV reduces complications with endotracheal ventilation; the patients remain awake, with reduced mortality and shorter ICU stays.
Disadvantages
These include lack of patient compliance, poorly fitting mask, and claustrophobia. Also, NIPPV offers no airway protection, and gastric distension can occur due to positive pressure and difficulty with oral feeding with the mask on.
Invasive Positive Pressure Ventilation
Invasive positive pressure ventilation is provided via an endotracheal tube (ETT) or tracheostomy. Once a patient is intubated for 2–3 weeks, it is prudent to change the ETT to a tracheostomy to prevent subglottic stenosis. The patient should be continuously monitored throughout (ECG, pulse oximetry, arterial blood pressure). A suction cannula should be readily available and put under the pillow before induction, plus a free flowing intravenous line should be available.
In unconscious patients, the ETT may be placed without sedation or muscle paralysis. After adequate preoxygenation, intubation may be facilitated with injection of drugs, such as midazolam, propofol, or etomidate, and succinylcholine. Ephedrine and phenylephrine should be available for hemodynamic support.
Using a small ETT may lead to high airway pressures; therefore, larger size ETTs should be used. Placement of the ETT is confirmed by auscultating the chest for breath sounds, using an ETCO2 detector and later by a chest radiograph. All intubated patients should receive pulmonary toilet, that is, suctioning of secretions, aerosol mists to administer bronchodilators, mucolytic agents, chest percussion, vibration therapy, and postural drainage. The aim in all mechanically ventilated patients is to oxygenate adequately, while preventing O2 toxicity.
Types of Ventilators
Ventilators are designed to give breaths which can be mandatory (controlled)—which is determined by the respiratory rate, assisted (as in assist control, synchronized intermittent mandatory ventilation (SIMV), pressure support), or spontaneous (no additional assistance in inspiration, as in CPAP). Ventilators can be generally classified as follows:
Volume controlled—or volume limited/targeted/cycled and pressure variable
Pressure controlled—or pressure limited/targeted/time cycled and volume variable
Dual controlled—volume targeted (guaranteed) and pressure limited
Flow cycled—such as in pressure support
Modes of Ventilation
Continuous Mandatory Ventilation
In continuous mandatory ventilation (CMV), the ventilator is programmed to a set tidal volume, respiratory rate, and I:E ratio (1:2) (Fig. 40.6). If the patient makes spontaneous ventilatory efforts between breaths, these are unsupported. For example, if the ventilator is set to deliver 10 bpm and the patient takes four spontaneous breaths/respiratory efforts per minute, the ventilator delivers the 10 set bpm, while the four patient breaths are unsupported.


Fig. 40.6
Airway pressure wave forms in different modes of ventilation (M mechanical breath, P patient effort, S spontaneous breath, ME mechanical exhalation)
Continuous mandatory ventilation (CMV) is most commonly used in the ICU in patients who are not breathing spontaneously. Usual ventilator settings are 10–12 breaths/min, tidal volume 6–10 ml/kg, desirable FiO2, and positive end-expiratory pressure (PEEP) of 5 cm H2O. With CMV, the patient receives the predicted minute ventilation, regardless of the patient’s effort. Disadvantage of CMV is that the airway pressure is variable and can be undesirably high, which can cause barotrauma.
Assist Control Ventilation
In assist-control ventilation (AC), in addition to the set mandatory breaths, the patient’s spontaneous efforts are fully supported by the ventilator, that is, each spontaneous effort by the patient leads to the delivery of a full ventilator-supported breath. For example, if the ventilator is set to deliver 10 bpm and the patient takes four spontaneous breaths/respiratory efforts per minute, the ventilator delivers a total of 14 bpm. The advantage of this mode is that the patient can breathe spontaneously without increasing the work of breathing.
Synchronized Intermittent Mandatory Ventilation
IMV is utilized in patients who are spontaneously breathing. The ventilator is set to deliver the desired tidal volume and respiratory rate, while the patient is allowed to breathe spontaneously in between the ventilator-delivered mandatory breaths. However, IMV can cause stacking of breaths, where the patient’s spontaneous breath may be stacked upon the ventilator’s mandatory breath (superimposing). To prevent the delivery of a large tidal volume breath and possibly barotrauma, SIMV is preferably used.
In SIMV, the ventilator synchronizes the delivery of mandatory breaths with the beginning of the patients’ own spontaneous breaths. If the patient does not initiate a breath, then the ventilator delivers the set tidal volume as in CMV mode. For example, if the ventilator is set to deliver 10 bpm and the patient takes four spontaneous breaths/respiratory efforts per minute, then six breaths are delivered as CMV, while four breaths are delivered as AC. Now, if the ventilator is set at 10 bpm and the patient is breathing spontaneously at 14 bpm, then ten breaths are delivered as AC, while four breaths are unsupported. These four breaths may increase the work of breathing. Therefore, pressure support may be added to assist the spontaneous breaths (SIMV with pressure support ventilation).
Pressure Control Ventilation
In pressure control ventilation (PCV), breaths are given to the patient at a set inspiratory pressure and respiratory rate. PCV may be used as assist control where both set and spontaneous patient breaths are delivered to the set pressure. Since PCV delivers breaths to a set and limited pressure, the risk of barotrauma is reduced. Disadvantage of PCV is that the delivered tidal volume is not guaranteed and requires more monitoring by the operator.
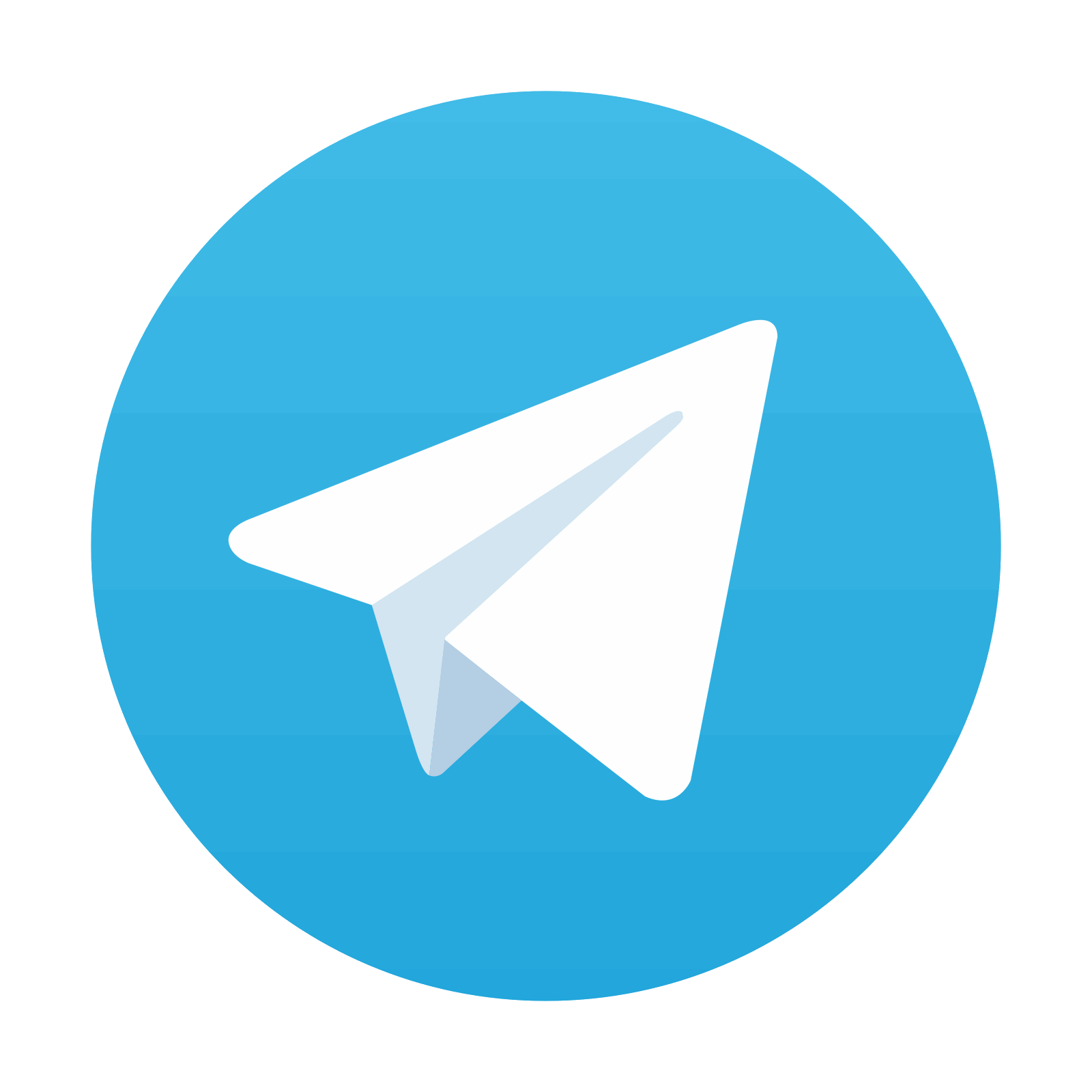
Stay updated, free articles. Join our Telegram channel

Full access? Get Clinical Tree
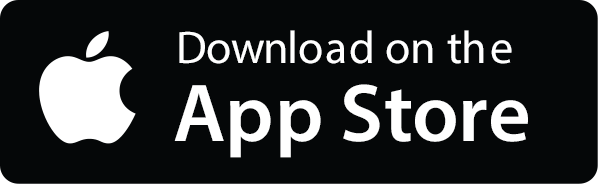
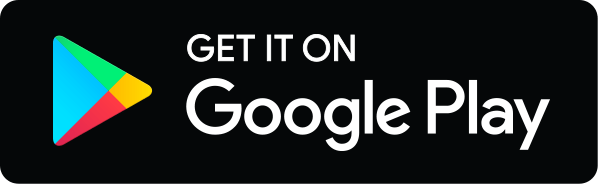
