The editors and publisher would like to thank Drs. James E. Baker and Isobel A. Russell for contributing to this chapter in the previous edition of this work. It has provided the framework for much of this chapter.
Categorization of congenital heart disease (CHD) may be based on distinctive anatomic or physiologic features of the defects ( Box 26.1 ). Sometimes complete comprehension of the anatomic complexities in a patient with CHD may be difficult because of a wide range of anatomic lesions. Fortunately, many lesions share similar pathophysiologic conditions despite their anatomic variations. Understanding these physiologic conditions will lead to successful management of a patient with complex CHD.
Acyanotic versus cyanotic—VSD versus TOF
Simple versus complex—ASD versus HLHS
Left-to-right shunt versus right-to-left shunt versus mixing lesions—ASD versus TOF versus HLHS
ASD, Atrial septal defect; HLHS, hypoplastic left heart syndrome; TOF, tetralogy of Fallot; VSD, ventricular septal defect.
Fundamental Pathophysiology in Congenital Heart Disease
Normally, pulmonary blood flow (Qp) and systemic blood flow (Qs) do not mix, and the entire cardiac output flows sequentially from one circulation to the other. All of the systemic venous return is directed to the pulmonary circulation and, likewise, all of the pulmonary venous return is directed to the systemic arterial circulation. Shunting occurs when a portion of the venous return of one circulation is redirected back to the arterial outflow of the same circulation. This redirected flow occurs when there is an abnormal communication or a defect between two otherwise separate structures. The relative downstream blood pressures of the communicating structures dictate the direction of the shunt flow, whereas the size of the defect determines the amount of shunting. Small defects tend to be restrictive with limited flow, and large defects tend to be nonrestrictive with unimpaired flow.
Left-to-Right Shunts
A left-to-right (L → R) shunt occurs when part of the pulmonary venous return is redirected toward the pulmonary arterial system. This defect may occur at numerous locations, including the pulmonary veins (anomalous pulmonary venous return), the atrial septum (atrial septal defect), the ventricular septum (ventricular septal defect [VSD]), and at the great vessels (patent ductus arteriosus [PDA]).
The portion of the pulmonary blood flow (Qp) that is redirected toward the pulmonary artery is recirculated pulmonary blood flow. The portion of the pulmonary blood flow that is appropriately directed toward the systemic circulation (Qs) is effective pulmonary blood flow. Their sum is the total pulmonary blood flow (Qp) ( Fig. 26.1 ).

Typically, CHD lesions with left-to-right (L → R) shunts remain acyanotic lesions. However, pulmonary overflow can result in pulmonary edema and hypotension. Prolonged hypotension can lead to circulatory shock with multiple organ system failure and lactic acidosis; the long-term effects of pulmonary overflow may be an increase of pulmonary vascular resistance (PVR) and abnormal cardiac chamber dilation. Over time an unrepaired large left-to-right (L → R) shunt can reverse its direction and become a cyanotic lesion. This is known as Eisenmenger physiology.
Right-to-Left Shunts
A right-to-left (R → L) shunt occurs when a portion of the systemic venous return is redirected to the systemic arterial outflow without first circulating through the lungs. The hallmark of lesions producing a right-to-left shunt is arterial oxygen desaturation. CHD lesions with right-to-left (R → L) shunts are cyanotic lesions. The physiologic effect of a right-to-left (R → L) shunt is arterial oxygen desaturation, because the recirculated oxygen-poor systemic venous blood mixes with the oxygen-rich pulmonary venous blood. The degree of desaturation depends upon the magnitude of right-to-left shunt as well as the degree of desaturation of the systemic venous return.
Mixing Lesions
Whereas a shunt connotes a communication between pulmonary and systemic venous circulations with partial mixing, many forms of CHD result in a complete blending of the two. Mixing lesions therefore are conditions in which oxygen content is equilibrated between the two circulations, yielding identical or nearly identical oxygen saturation at both the pulmonary and systemic arterial level.
As with right-to-left shunts, one of the chief characteristics of mixing lesions is systemic arterial oxygen desaturation. The degree of desaturation depends on the flow volume of the two contributing circulations as well as the difference in the individual oxygen saturation. A decrease in pulmonary venous saturation from apnea or atelectasis will decrease the saturation of the final mixed circulation. A decrease in the systemic venous saturation will also cause the final systemic arterial saturation to decrease. Factors that cause a decrease in systemic venous oxygen saturation include fever (increase of systemic oxygen consumption), low cardiac output states (which cause increased oxygen extraction in the microvasculature), and anemia (decrease in systemic oxygen delivery).
Significance of Qp:Qs Ratio in Mixing Lesions
In CHD with mixing physiology, a Qp:Qs ratio close to 1 will maximize the effective component of each circulation and minimize the wasteful recirculated component. For Qp:Qs ratio more than 1, preferential flow toward the pulmonary artery increases pulmonary blood flow, resulting in increased oxygen saturation of the mixed blood, but decreases systemic cardiac output and yields less oxygen delivery. For a Qp:Qs ratio less than 1, preferential flow toward the aorta increases systemic blood flow leading to higher systemic perfusion pressure, but the increased output contains blood with lower oxygen saturation and also leads to a decrease in oxygen delivery.
Relative resistance to flow of the two circulations, PVR and systemic vascular resistance (SVR), determines the Qp:Qs ratio. If PVR exceeds SVR, Qs will exceed Qp. Likewise, if SVR exceeds PVR, Qp will exceed Qs. SVR and PVR are both affected by many factors, which are listed in Box 26.2 .
Events That Increase Systemic Vascular Resistance
Light anesthesia
Sympathetic nervous system activation
Administration of α-agonists
Physical manipulations (e.g., compression of the femoral arteries by flexing the hips of infants and small children)
Events That Decrease Systemic Vascular Resistance
Deep anesthesia
Administration of vasodilating drugs—nitrates, intravenous and inhaled anesthetics
Events That Increase Pulmonary Vascular Resistance
Alveolar hypoxemia (e.g., from low inspired oxygen concentrations)
Hypercapnia
Acidosis
High lung volumes or pressures—tend to collapse pulmonary capillaries
Low lung volumes with atelectasis—tend to collapse larger pulmonary blood vessels
Light anesthesia
Sympathetic nervous system stimulation
Hypothermia
Events That Decrease Pulmonary Vascular Resistance
Hyperventilation
Hypocarbia
Alkalosis
Oxygenation
Inhaled nitric oxide
Warmth
Bronchodilators (e.g., albuterol)
Significance of the Patent Ductus Arteriosus
The ductus arteriosus connects the pulmonary artery to the descending aorta and is functionally closed within 4 days after birth in a healthy neonate as a result of an increase in arterial oxygen tension and decrease in placental prostaglandins (also see Chapter 34 ). However, mixing lesions with one functional ventricle often require a PDA to supply blood flow to the underdeveloped side. Shunting through the PDA in systole is either left to right (e.g., pulmonary atresia with intact ventricular septum) or right to left (e.g., hypoplastic left heart syndrome [HLHS]), depending on which side of the heart is hypoplastic. In other words, systemic blood flow is ductal-dependent in certain lesions such as HLHS. For other lesions such as pulmonary atresia the ductus arteriosus is required for pulmonary blood flow.
Ductal shunting during diastole, however, is usually left to right through the PDA because the aorta has a higher resting tone than the pulmonary artery. This means that a large amount of blood can be diverted to the lungs and away from the coronary arteries during diastole. Consequently the myocardium may become ischemic and infarcted because of coronary ischemia. Maneuvers that decrease PVR will cause more pulmonary runoff and exacerbate coronary ischemia.
Eisenmenger Syndrome
CHD may subject the lungs to abnormal blood flow or pulmonary artery pressure ( Box 26.3 ). Over time, the pulmonary vasculature may undergo a process of remodeling with a gradual increase in PVR that results in pulmonary hypertension, even if the underlying hemodynamic problem is corrected. When pulmonary hypertension becomes irreversible and pulmonary pressure becomes supersystemic, blood is preferentially directed toward the systemic circulation and the direction of the shunt is right to left (R → L) even if the original shunting pattern was left to right (L → R). This condition is called Eisenmenger syndrome and often is a contraindication for surgical correction of the shunt.
Increased Pulmonary Blood Flow
Atrial septal defect
Anomalous pulmonary venous return
Increased Pulmonary Artery Pressure
Ventricular septal defect
Atrioventricular canal defect
Aortopulmonary window
Truncus arteriosus
Transposition of the great arteries
Double-inlet left ventricle
Patent ductus arteriosus
Obstructive Lesions
Obstructive lesions consist mainly of left ventricular outflow track (LVOT) obstructions and coarctation of the aorta.
Left Ventricular Outflow Track Obstruction
LVOT obstructive lesions account for about 3% to 6% of CHD and can occur at subvalvar, valvar, and supravalvar levels. Valvar aortic stenosis is the most common form of LVOT obstruction in children. Bicuspid aortic valve is the most prevalent abnormality in this disease and often leads to clinical manifestations in early adulthood. Subvalvar aortic stenosis encompasses a variety of lesions, which include a thin membrane, thick fibromuscular ridge, diffuse tunnel-like obstruction, and abnormal mitral valve attachments. Supravalvar aortic stenosis ( Fig. 26.2 ) often has an hourglass deformity consisting of a discrete constriction of a thickened ascending aorta at the superior aspect of the sinuses of Valsalva and is often a feature in patients with Williams syndrome.

In utero, critical aortic stenosis can lead to HLHS. Infants with severe aortic stenosis present with heart failure and failure to thrive. Older children with aortic stenosis are rarely symptomatic but will develop left ventricular hypertrophy, premature coronary atherosclerosis, and congestive heart failure over time. Various interventional and surgical approaches are available for aortic stenosis including balloon valvuloplasty, the Ross-Konno procedure, resection of the obstruction, and valve replacement. Timing and type of the surgery depend on the individual case.
Coarctation of the Aorta
Coarctation of the aorta is a discrete narrowing of the thoracic aorta just distal to the left subclavian artery ( Fig. 26.3 ). It can be an isolated lesion or may be found in conjunction with other lesions such as aortic stenosis and VSDs. Infants with critical coarctation are at risk of developing heart failure and death when the ductus arteriosus closes. Those infants whose circulation is ductal dependent need to continue intravenous prostaglandin E 1 infusion to maintain the patency of the ductus arteriosus until surgery. Long-term follow-up of patients with coarctation of the aorta is imperative as there are long-term sequelae in adulthood, including recoarctation, hypertension, aortic aneurysm, coronary artery disease, and stroke. In parturients, preeclampsia can occur in pregnancy, even after successful surgical correction (also see Chapter 33 ).

Perioperative Management
Surgery for CHD is planned with the cooperative input of a multidisciplinary team that includes surgeons, cardiologists, critical care specialists, and anesthesiologists. Patients require optimal medical care prior to surgery. For the anesthesia provider, understanding the physiology of the cardiac lesion and the subsequent effects of the planned surgical palliative or corrective procedure will lead to successful management of the patient (also see Chapter 13 ).
History and Physical Examination
A review of the patient’s history includes attention to details that are of importance to pediatric anesthetic care in general, such as pertinent pregnancy details, prematurity, and postnatal course (also see Chapter 34 ). Patients with CHD frequently have associated syndromes (trisomy 21, DiGeorge syndrome) or evidence of chronic illness (renal dysfunction, pulmonary edema, imbalances in electrolyte and glucose metabolism). Complete review of the patient’s preoperative medications and laboratory studies (i.e., complete blood count, electrolytes, coagulation studies, indices of renal and hepatic function) is mandatory.
The anesthesia provider should review the available diagnostic studies such as the echocardiograms ( Fig. 26.4 ) and cardiac catheterization studies (see Fig. 26.2 ). Magnetic resonance imaging (MRI) will also provide invaluable anatomic details (see Fig. 26.4 ). Electrocardiograms and chest radiographs are part of the routine preoperative evaluation. Medical and surgical interventions that have been instituted previously and any interim change or deterioration in the patient’s status are evaluated. Previous sternotomy is a risk factor for increased operative blood loss and cardiac trauma during dissection as a result of adhesions to the sternum and chest wall. Many hospitalized neonates require continuous infusions of inotropic drugs or other medications such as prostaglandin E 1 to maintain ductal patency and hemodynamic stability while awaiting surgery.

Physical examination includes an assessment of airway problems (such as might occur in patients with genetic syndromes, e.g., trisomy 21), signs of congestive heart failure (tachypnea, wheezing, dilated neck veins), cyanosis, nutritional status, and any other coexisting conditions. Outpatients who have been scheduled for elective surgery are evaluated the day of surgery for new onset of signs or symptoms of an intercurrent illness such as an upper respiratory tract infection. Inpatients are evaluated for their hospital course along with any developing problems such as an increased white blood cell count, which could indicate the presence of an infectious or inflammatory process.
Preparation for Surgery
All patients must follow the standard American Society of Anesthesiologists (ASA) guidelines for fasting. Outpatients taking cardiac medications are generally advised to continue therapy up to and including the day of surgery, although preference may vary among anesthesia providers regarding diuretics and angiotensin-converting enzyme inhibitors as well as angiotensin receptor blocking (ARB) drugs. Anticoagulants and antiplatelet medications are usually not given several days before surgery.
Operating Room Setup
Preparation of the operating room should include readiness of age-appropriate airway equipment, intravenous equipment, and invasive monitors. All intravenous administration sets should be meticulously de-aired to prevent paradoxical arterial embolization of intravenous air bubbles. A warming blanket or surface cooling equipment is made available, and adjustment of the operating room temperature precedes entry of the patient (27° C for small or premature infants and 24° C for older children). Hemodynamic medications are prepared in weight-appropriate dilutions before surgery. Preparation also includes readiness of specialized equipment such as transesophageal echocardiography (TEE) or nitric oxide (iNO) delivery systems.
Induction of Anesthesia
Induction of anesthesia for patients with CHD may involve either the use of inhaled or intravenous anesthetics ( Boxes 26.4 and 26.5 ). The goal is to execute a smooth anesthetic induction to avoid increased anxiety, crying, coughing, or breath-holding. These events may aggravate unfavorable physiologic effects such as increased right-to-left shunting and dynamic right- or left-sided ventricular outflow tract obstruction in susceptible patients.
Sevoflurane 1.5%-3.5% (ET)
Decrease SVR, contractility
Decrease dose if N 2 O used; may cause myocardial depression
Fentanyl 20-50 μg/kg
Nonsignificant effect on contractility, SVR
May cause loss of sympathetic tone, bradycardia
May cause chest wall rigidity with rapid administration or large doses
Sufentanil 5-10 μg/kg
Similar to fentanyl
Ketamine (IV) 1-2 mg/kg
Increases HR, increase or no change in SVR, PVR
Actually a myocardial depressant with sympathetic stimulant properties; may decrease contractility in patients with depleted SNS
May cause bronchorrhea (prevented with atropine, 20 μg/kg)
Ketamine (IM) 3-5 mg/kg
Etomidate (IV) 0.2-0.3 mg/kg
Preserves HR, SVR, PVR, contractility
May inhibit endogenous corticosteroid production; burning pain at the injection site
BP, Blood pressure; CHF, congestive heart failure; CO, cardiac output; ET, end-tidal; HR, heart rate; IM, intramuscular; IV, intravenous; PVR, pulmonary vascular resistance; SNS, sympathetic nervous system; SVR, systemic vascular resistance.
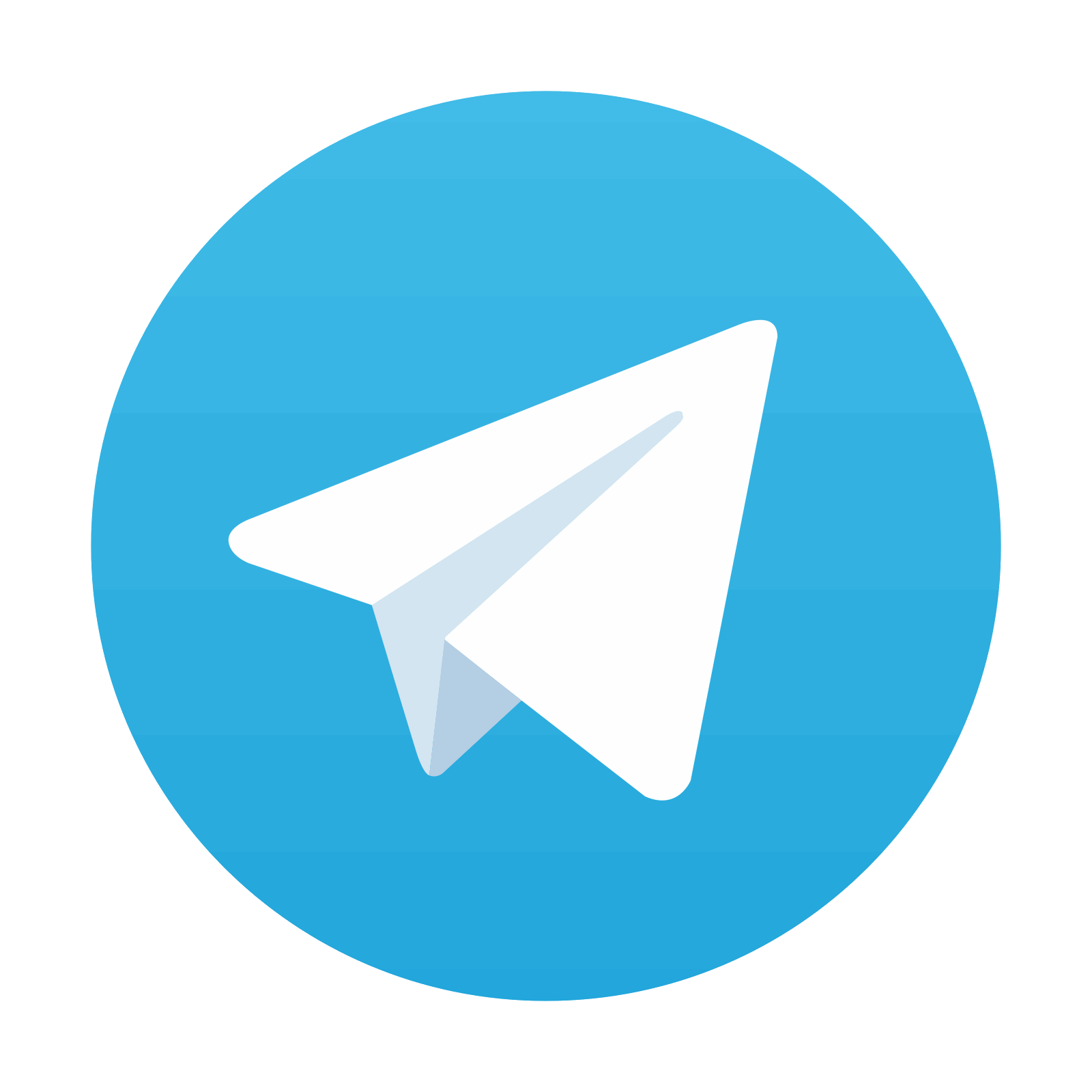
Stay updated, free articles. Join our Telegram channel

Full access? Get Clinical Tree
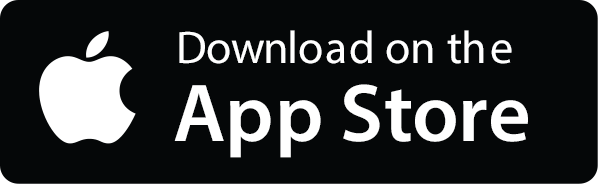
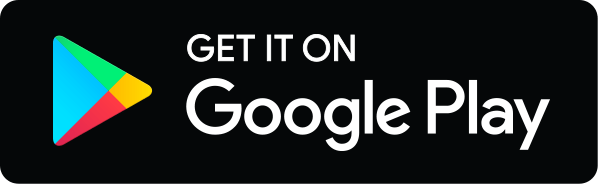
