The endothelium is a specialized organ that plays a central role in the regulation of clot formation (i.e., hemostasis) by presenting a nonthrombogenic surface to flowing blood and by enhancing clot formation when the endothelium is disrupted by trauma or injured by infection or inflammation (Fig. 143.4) (5,6). The normal endothelium produces inhibitors of blood coagulation and platelet activation and modulates vascular tone and permeability. Endothelial cells also synthesize and secrete the components of the subendothelial extracellular matrix including adhesive glycoproteins, collagen, fibronectin, and vWf. When disrupted, bleeding occurs. However, when injured, the endothelium often becomes a prothrombotic rather than an antithrombotic organ and unwanted clot formation may occur. The final phase in hemostasis, fibrinolysis, is also dependent on both plasma and endothelial cell factors (Fig. 143.5). None of these are measured in the traditional tests of coagulation (i.e., PT, aPTT) and, consequently, these tests will be normal unless the pace of fibrinolysis is such that clotting factor consumption is greater that replacement (i.e., a consumptive coagulopathy, DIC) (7). Fibrinolysis is initiated once thrombin is generated and will result in an increase in fibrin and fibrinogen fragments (D-dimer or FSPs/FDPs, respectively). Thrombin binding to thrombomodulin on the endothelial surface is a critical step in the thrombin-mediated activation of protein C (to produce activated protein C; aPC) and for the activation of thrombin-activatable fibrinolysis inhibitor (TAFI). However, activation or injury to endothelial cells frequently results in a shedding of surface expressed thrombomodulin (and an increase in plasma soluble thrombomodulin) and, therefore, a downregulation in intrinsic anticoagulation (i.e., decrease in aPC) and fibrinolysis inhibition (i.e., decreased TAFI), both of which will increase the risk of microvascular thrombosis and development of multiorgan dysfunction (8,9).
INTERACTION OF COAGULATION AND INFLAMMATION
There are multiple points of intersection between the biochemical events of inflammation and those of coagulation and fibrinolysis (10–12). While a full discussion of these points is beyond the scope of this chapter, the “cross-talk” between inflammation and coagulation likely takes place at the level of the endothelium, and is bidirectional wherein activation of either pathway affects the functioning of the other (10). While many different inflammatory cytokines have been identified as promoters of a procoagulant milieu, the interconnection of TF and tissue necrosis factor-α (TNF-α) may potentially be the most important of these (13). During sepsis, tissue factor expression is upregulated in activated monocytes and endothelial cells as a response to endotoxin, with the consequence being both the secretion of pro-inflammatory cytokines (e.g., IL-6, TNF) from activated mononuclear cells, and the activation of coagulation. This results in increased thrombin production which plays a central role in coagulation and inflammation through the induction of procoagulant, anticoagulant, inflammatory, and mitogenic responses (12). Thrombin results in the activation, aggregation and lysis of leukocytes and platelets, activation of endothelial cells with resultant increase in pro-inflammatory cytokines IL-6 and TNF expression. The net result of thrombin generation is to produce a pro-inflammatory and procoagulant state leading to the formation of fibrin and microvascular thrombosis. However, these pro-inflammatory effects of thrombin are counterbalanced by the anti-inflammatory effects of activated protein C (aPC) (see Fig. 143.4) (12).

FIGURE 143.1 Classical coagulation pathway. Coagulation is initiated either through the intrinsic pathway by activation of factor XII by the generation of high–molecular-weight kininogen and kallekrein, or through activation of the extrinsic pathway by tissue factor. Roman numerals indicate zymogen clotting factors; “a” indicates activated forms of the clotting factors.

FIGURE 143.2 Modified clotting cascade indicating cross-talk between the intrinsic and extrinsic pathways by the action of VIIa/tissue factor (TF) enhancing the conversion of factor XI to activated factor XI (XIa) (dotted lines).

FIGURE 143.3 The role of platelets in mediating primary hemostasis at sites of vascular injury. Platelets are initially activated and express specific adhesion receptors on their surface followed by adhesion to activated endothelial cells and exposed subendothelial components (e.g., collagen, von Willebrand factor). Subsequent platelet aggregation occurs with the development of a primary platelet plug. Coagulation occurs on the developing platelet plug with the creation of a fibrin clot.

FIGURE 143.4 The interaction of the protein C system with the endothelium. Thrombin bound to thrombomodulin (TM) modifies protein C bound to the endothelial protein C receptor (EPCR) on the cell surface to generate activated protein C (aPC). aPC acts as a natural anticoagulant by inactivating activated factors V (fVa) and VIII (fVIIIa), modulates inflammation by down regulating the synthesis of pro-inflammatory cytokines, leukocyte adherence and apoptosis, and enhances fibrinolysis by inhibiting thrombin activatable fibrinolysis inhibitor (TAFI) and plasminogen activator inhibitor type-1 (PAI-1).
The second important point of connection of coagulation and inflammation is through the protein C system (14–16). While the anticoagulant effects of aPC and its cofactor protein S are well known, only recently have the anti-inflammatory roles of these proteins been appreciated. In experimental models, aPC has been shown to increase the secretion of anti-inflammatory cytokines, reduce leukocyte migration and adhesion, and protect endothelial cells from injury. Additionally, the balance between the anticoagulant and anti-inflammatory roles of aPC may be mediated by the relative distribution of free and C4BP bound protein S (15,16). In vitro, aPC inhibits TNF-α elaboration from monocytes and blocks leukocyte adhesion to selectins, as well as influences apoptosis (12). The protein C pathway is engaged when thrombin binds to thrombomodulin on the surface of the endothelium. Binding of PC to the endothelial cell protein C receptor (EPCR) augments protein C activation by the thrombin–TM complex more than 10-fold in vivo. EPCR is shed from the endothelium through the action of inflammatory mediators and thrombin thereby down regulating aPC generation in sepsis and inflammation.
The third important link between inflammation and coagulation occurs at the level of fibrinolysis and also involves the protein C system. aPC is capable of neutralizing the fibrinolysis inhibitors plasminogen activator inhibitor type-1 (PAI-1) and thrombin activatable fibrinolysis inhibitor (TAFI). Consequently, depressed levels of aPC not only promote clot formation by reducing the inactivation of the procoagulant molecule–activated factors V and VIII (F.Va, F.VIIIa) leading to increased generation of thrombin and fibrin clots, but also by limiting the fibrinolytic response needed to degrade clots. TAFI (also known as carboxypeptidase R) has also been shown to inactivate inflammatory peptides such as complement factors C3a and C5a which can play a role in the contact activation of coagulation. In addition, polymorphisms of the promoter region of the PAI-1 gene which lead to differences in PAI-1 production have been demonstrated to affect the prognosis in meningococcal sepsis in and multiple trauma pointing out the important role of this regulatory system (17). This finding points out the importance of our developing knowledge of how common polymorphisms of genes encoding important molecules affect our response to infection and injury. The importance of these interactions between coagulation and inflammation, the central role of protein C, and the importance gene polymorphisms play in host responses and clinical outcomes is further reinforced by a recent report demonstrating increased mortality and organ dysfunction and increased inflammation in patients who exhibited a specific polymorphism (1641AA) of the protein C gene (18).

FIGURE 143.5 The generation of thrombin results in activation of endothelial cells with production of tissue-type plasminogen activator (tPA) and urothelial-type plasminogen activator (uPA). Plasminogen is converted to plasmin by tPA or uPA with resultant degradation of fibrin producing D-dimer fragments. Thrombin bound to thrombomodulin on the surface of endothelial cells activates protein C to activated protein C (aPC) and thrombin activatable fibrinolysis inhibitor (TAFI) to activated TAFI. TAFIa inhibits the action ofplasmin; additional inhibitors of plasmin are α2-antiplasmin (α2-AP) and α2-macroglobulin (α2M). Activated PC acts as an anticoagulant by degrading F.Va and F.VIIIa, and inhibiting the conversion of prothrombin to thrombin by the prothrombinase complex. Both tPA and uPA are inhibited by plasminogen activator inhibitor-type 1 (PAI-1).
AN APPROACH TO THE PATIENT WITH AN ACTUAL OR SUSPECTED COAGULATION DISORDER
Clinical History
Diagnostic assessment begins at the bedside. The medical history, both past and present, may lend some insight into the risk for significant bleeding (19,20). A prior history of prolonged or excessive bleeding or of recurrent thrombosis is important to elicit. Specific questions regarding bleeding should investigate the occurrence of any of the following: spontaneous, easy, or disproportionately severe bruising; intramuscular hematoma formation (either spontaneous or related to trauma); spontaneous or trauma-induced hemarthrosis; spontaneous mucous membrane bleeding; prior problems with bleeding related to surgery (including dental extractions, tonsillectomy, and circumcision); the need for transfusions in the past; menstrual history; and, finally, current medications. There are innumerable aspirin-containing medications available to the consumer, all of which can potentially interfere with platelet-mediated primary hemostasis. Many other drugs used in the ICU also are associated with bleeding abnormalities and are discussed later in this chapter. In situations involving trauma (either surgical or accidental), it is important to determine the severity of injury relative to the magnitude of bleeding that followed. A prior history of significant thrombosis (e.g., deep venous thrombosis, pulmonary embolus, stroke) also suggests the possibility that a hypercoagulable condition may be present. As thrombotic events are generally uncommon in younger adults, the occurrence of thrombotic events, particularly early cardiovascular events such as myocardial infarction, in young adult relatives should cause the clinician to consider the presence of a congenital thrombophilic abnormality in his patient. These include deficiencies of antithrombin III, protein C or protein S, the presence of the factor V Leiden R506Q mutation, and the prothrombin G20210A polymorphism/mutation. While the C677T mutation/polymorphism of the MTHFR (methylenetetrahydrofolate reductase) gene has previously been identified as a thrombotic risk factor, more recent evaluation of the available data suggests that the thrombophilic risk of this genetic may principally derive from the increase in serum homocysteine that can result from this mutation. Consequently, it may be that only in the presence of an elevated serum homocysteine is the C677T mutation of the MTHFR gene clinically relevant (21–23). In addition, vasculitis associated with an “autoimmune” disorder such as systemic lupus erythematosus (SLE) must always be considered in the evaluation of an individual with an unexplained pathologic clot. In all cases, the family history is important in trying to separate congenital from acquired disorders.
In a general sense, one can segregate defects into those involving primary or secondary hemostasis according to the nature of the bleeding. Patients with primary hemostatic defects tend to manifest “platelet- or capillary-type bleeding”—oozing from cuts or incisions, mucous membrane bleeding, or excessive bruising. This type of bleeding is seen in patients with quantitative or qualitative platelet defects or von Willebrand disease. In contrast, patients with deficits in secondary hemostasis tend to display “large-vessel bleeding” characterized by hemarthroses, intramuscular hematomas, and the like. This type of bleeding is most often associated with specific coagulation factor deficiencies or inhibitors.
Physical Examination
Development of generalized bleeding in critically ill ICU patients presents a special problem. Such bleeding is often associated with severe underlying multiple organ system dysfunction. Thus, correction of the coagulopathy usually requires improvement in the patient’s overall clinical status. Supportive evidence or physical findings of other concurrent organ system dysfunction (e.g., oliguria or anuria, respiratory failure, hypotension) often are readily apparent. With the exception of massive transfusion syndrome (discussed later), generalized bleeding in critically ill patients is often caused by sepsis-related DIC (24,25). However, the clinician must also consider the coagulopathy of severe liver dysfunction, undiagnosed hemophilia, or, in the elderly or debilitated, vitamin K deficiency in the differential diagnosis (24–26).
The physical examination of the patient with a bleeding disorder should answer several basic questions. Is the process localized or diffuse? Is it related to an anatomic or surgical lesion? Is there mucosal bleeding? And finally, when appropriate, are there signs of thrombosis (either arterial or venous)? These answers may give clues to the cause of the problem (primary vs. secondary hemostatic dysfunction; anatomic bleeding vs. generalized coagulopathy).
During the course of the examination, particular attention should be paid to the presence of several specific physical findings that may be helpful in determining the etiology of a suspected hemostatic abnormality. For example, the presence of an enlarged spleen coupled with thrombocytopenia suggests that splenic sequestration may be a contributor to the observed thrombocytopenia. Further, evidence of liver disease (e.g., portal hypertension, ascites) points to decreased factor synthesis as a possible etiology of a prolonged PT or aPTT. When lymphadenopathy, splenomegaly, or other findings suggestive of disseminated malignancy are detected, acute or chronic DIC should be suspected as the cause of prolonged coagulation times, hypofibrinogenemia, and/or thrombocytopenia. Purpura that are palpable suggest capillary leak from vasculitis, whereas purpura associated with thrombocytopenia or qualitative platelet defects are generally not elevated and cannot be distinguished by touch. Finally, venous and arterial telangiectasia may be seen in von Willebrand disease and liver disease, respectively. When selective pressure is centrally applied to an arterial telangiectasia, the whole lesion fades, whereas a venous telangiectasia requires confluent pressure across the entire lesion (as with a glass slide) for blanching to occur.
Diagnostic Laboratory Evaluation
This section focuses on selecting appropriate tests to enable the clinician to sort out information from the history, physical examination, or previously obtained (and often confusing) laboratory data. Before we proceed, however, the importance of correct specimen collection for hemostatic evaluation must be emphasized. In the ICU, it is common for laboratory samples to be drawn through an indwelling arterial or central venous cannula, often because other access is no longer available. Heparin is, therefore, commonly present, either in solutions used to flush the cannula, to transduce a waveform, or as a component of the intravenous infusion. Depending on the concentration of heparin in the infusing fluid and the volume of blood withdrawn, several tests can be influenced. Fibrin degradation products (FDPs) can be falsely elevated and fibrinogen can be falsely low. Likewise, the PT, aPTT, and thrombin time (TT) can be spuriously prolonged. A minimum of 20 mL of blood in adolescents and adults (10 mL of blood in younger children) should therefore be withdrawn through the cannula and either discarded or used for other purposes before obtaining a specimen for laboratory hemostasis analysis (27). This practice should minimize any influence of heparin on the results. In some clinical situations, it may not be reasonable to withdraw this volume of blood and a peripheral venipuncture may be necessary. Because the aPTT is sensitive to the presence of small amounts of heparin, the presence of an unexpected prolonged aPTT obtained through a heparinized catheter should raise the suspicion of sample contamination. In this setting, the TT also will be prolonged but will normalize if the contaminating heparin is neutralized (e.g., with toluidine blue or protamine sulfate).
The presence of most suspected bleeding disorders can be confirmed using routinely available tests. These include evaluation of the peripheral blood smear (including an estimate of the platelet count and platelet and red blood cell morphologic features); measurement of the PT, aPTT, and the TT; and, finally, assays for fibrinogen or the presence of FDPs or the D-dimer fragment of polymerized fibrin. This latter test is more specific for the fibrinolytic fragment produced when polymerized fibrin monomer, produced through the action of thrombin on fibrinogen, is cleaved by the proteolytic enzyme plasmin (8). In contrast to the older assays for fibrin degradation or fibrin-split products (FDPs and FSPs) which will be positive even if fibrin is not produced and the fragments are the result of proteolytic degradation of native fibrinogen, the D-dimer assay is positive only if fibrinogen has been cleaved to fibrin by the action of thrombin. Discretion should be used in determining which of these tests are most appropriate for assessment; they need not be ordered as a blanket panel on all patients with known or suspected bleeding disorders. Table 143.2 summarizes several major categories of hemorrhagic disorders and the tests that are characteristically abnormal in each. In most instances, measurement of the platelet count, fibrinogen level, PT, aPTT, and TT should provide sufficient information for determining the correct diagnosis—or at least making an educated guess. By using these five screening tests and assessing other more specific tests only when an absolute diagnosis is necessary, inappropriate use of laboratory resources may be avoided. The use of thromboelastography as tool to both assess hemostasis and guide transfusion of blood products in patients is frequently employed in Europe but less often in North America. While this methodology (thromboelastography [TEG] or rotational thromboelastometry [ROTEM]) offers potential benefits over plasma-based tests (e.g., aPTT, PT, TT), by simultaneously assessing the multiple elements of hemostasis in whole blood, adoption of this testing methodology has been sporadic as it has not yet been shown to be superior to the more traditional plasma-based tests of coagulation (28–30).
TABLE 143.2 Hemorrhagic Syndromes and Associated Laboratory Findings | ||
![]() |
Evaluation of Thrombosis
Patients who present with a thrombotic event will generally not display abnormalities of usual “clotting” studies, that is, their PT, aPTT, TT, and fibrinogen will usually be within normal ranges. While hyperfibrinogenemia and persistent elevations of F.VIII have been associated with an increased risk of thrombosis, both may be elevated by acute inflammation and consequently the finding of elevations of these clotting factors is of limited usefulness in the evaluation of a thrombotic event in an acutely ill individual. Other studies such as TEG, ROTEM, measurement of endogenous thrombin potential (ETP), indirect markers of thrombin generation (e.g., prothrombin fragment F1+2, thrombin–antithrombin complexes [TAT], and markers of fibrinolysis activation and inhibition (e.g., plasmin–antiplasmin complexes [PAP], PAI-1 activity) may be more useful in identifying those patients most at risk for thrombotic events (31). However, most of these studies do not fall into the category of “routine” tests readily available with a rapid turnaround time that would facilitate acute care decision-making. Several inherited or acquired abnormalities that place an individual at increased risk for thrombosis have been identified and determination of these factors should be undertaken when a thrombotic event is suspected or documented. Prior to initiation of anticoagulation, plasma levels of protein C (antigen and activity), protein S (antigen and activity; total and free), and antithrombin III (antigen and activity) should be obtained. In addition, PCR analysis for mutations in the factor V (factor V Leiden; [Arg]R506Q[Gln]), and prothrombin ([Gly]G20210A[Ala]) genes should be performed; determination of the methylenetetrahydrofolate reductase (MTHFR; [Cys]C677T[Thr]) mutation can be deferred unless serum homocysteine is found to be elevated. Acquired thrombotic risk factors include the presence of “Lupus” anticoagulants, antiphospholipid and anticardiolipin antibodies which may be associated with underlying autoimmune disorders or with acute inflammation. In adult populations, approximately 40% of patients with thrombosis will not display one of the known thrombophilic risk factors. The intensivist must look for confounding clinical conditions such as severe dehydration with marked hemoconcentration (in the case of central venous sinus thrombosis), indwelling catheters, vascular compression (e.g., cervical ribs), type-II heparin-induced thrombocytopenia (see below), etc. in their evaluation of a patient with thrombosis.
CONDITIONS ASSOCIATED WITH SERIOUS BLEEDING OR A HIGH PROBABILITY OF BLEEDING
Disseminated Intravascular Coagulation
Pathogenesis
Because it often occurs in conjunction with more serious, life-threatening disorders, DIC is one of the most serious hemostatic abnormalities seen in the ICU. The clinical syndrome itself results from the activation of blood coagulation, which then leads to excessive thrombin generation. The final result of this process is the widespread formation of fibrin thrombi in the microcirculation, with resultant consumption of certain clotting factors and platelets. Ultimately, this consumption generally results in the development of significant bleeding as the rate of consumption outpaces the rate at which the clotting factors and platelets are produced (32). Table 143.3 reviews several specific conditions associated with the development of DIC. In general, the conditions associated with DIC are the same for either adult or pediatric populations. These include a wide variety of disorders that share as their common feature the ability to initiate coagulation to varying degrees. The mechanisms involved generally can be considered in two categories: (1) those intrinsic processes that enzymatically activate procoagulant proteins; and (2) those that cause the release of tissue factor, which then triggers coagulation. These are complex events that can lead to significant bleeding and often complicate the management of an already critically ill patient.
TABLE 143.3 Underlying Diseases Associated With Disseminated Intravascular Coagulation |
![]() |
Fibrinolysis invariably accompanies thrombin formation in DIC (32). Thrombin generation or release of tissue plasminogen activator usually initiates this process. Plasmin is generated which then digests fibrinogen and fibrin clots as they form. Plasmin also inactivates several activated coagulation factors and also impairs platelet aggregation. DIC represents an imbalance between the activity of thrombin, which leads to microvascular thrombi with coagulation factor and platelet consumption, and plasmin, which degrades these fibrin-based clots as they form. Therefore, thrombin-induced coagulation factor consumption, thrombocytopenia, and plasmin generation all contribute to the presence of bleeding.
In addition to bleeding complications, the presence of fibrin thrombi in the microcirculation also can lead to ischemic tissue injury. Pathologic data indicate that renal failure, acrocyanosis, multifocal pulmonary emboli, and transient cerebral ischemia may be related clinically to the presence of such thrombi. The fibrinopeptides A and B (resulting from enzymatic cleavage of fibrinogen) lead to pulmonary and systemic vasoconstriction, which can potentiate an existing ischemic injury. In a given patient with DIC, either bleeding or thrombotic tendencies may predominate; in most patients, bleeding is usually the predominant problem. In up to 10% of patients with DIC, however, the presentation is exclusively thrombotic (e.g., pulmonary emboli with pulmonary hypertension, renal insufficiency, altered mental status, acrocyanosis) without hemorrhage. Whether the presentation of DIC is thrombotic, hemorrhagic or “compensated” (i.e., laboratory results consistent with DIC without overt bleeding), microthrombosis probably contributes to the development and progression of multiorgan failure.
Clinical Presentation and Diagnosis
The suspicion that DIC is present usually stems from one of two situations: (1) unexplained, generalized oozing or bleeding; or (2) unexplained abnormal laboratory parameters of hemostasis. This usually occurs in the context of a suggestive clinical scenario or associated disease (see Table 143.3). While infection and multiple trauma are the most common underlying conditions associated with the development of DIC, certain other organ system dysfunctions predispose to DIC, including hepatic insufficiency and splenectomy (24,25). Both of these conditions are associated with impaired reticuloendothelial system function and consequent impaired clearance of activated coagulation proteins and fibrin/fibrinogen degradation fragments which may inhibit fibrin polymerization and clot formation.
The clinical severity of DIC frequently has been assessed by the severity of bleeding and coagulation abnormalities. Recently, scoring tools employing a panel of laboratory tests along with severity of illness scores to assess the likelihood and severity of DIC have been proposed in an attempt to determine prognosis and direct initial therapy at the time of diagnosis. A listing of the tests most commonly employed in many of these scoring systems for the diagnosis of DIC are found in Table 143.4. The use of these scoring systems for the early diagnosis and treatment of DIC does appear to have prognostic value, particularly in patients with sepsis (33–35). The systems suggested by Leclerc (36) (for children) and those developed by the International Society on Thrombosis and Hemostasis (ISTH) (37) and Japanese Association for Acute Medicine (JAAM) (38) are three of the more commonly employed scoring systems and may serve as a template for the diagnosis of DIC: a qualitative score (three out of tests positive; Leclerc) or a quantitative score (five points ISTH, four points JAAM) are strongly suggestive of a diagnosis of DIC. The combination of a prolonged PT, hypofibrinogenemia, and thrombocytopenia in the appropriate clinical setting is sufficient to suspect the diagnosis of DIC in most instances. Severe hepatic insufficiency (with splenomegaly and splenic sequestration of platelets) also can yield a similar laboratory profile and must be ruled out.
TABLE 143.4 Laboratory Tests for the Diagnosis of DIC | |
![]() |
In addition to liver disease, several other conditions have presentations similar to DIC and must be considered in the differential diagnosis:
Liver disease
Massive transfusion
Primary fibrinolysis
Thrombotic thrombocytopenic purpura (TTP)/hemolytic uremic syndrome (HUS)
Heparin therapy
Dysfibrinogenemia
With the exception of massive transfusion syndrome, these disorders generally have only two of the three characteristic laboratory findings of DIC; a comparison of the laboratory findings in these disorders is noted in Table 143.2. In order to confirm a diagnosis of suspected DIC, confirmatory tests indicating increased fibrinogen turnover (i.e., elevated FDPs or D-dimer assay) may be necessary. The D-dimer assay for the D–D fragment of polymerized fibrin has been shown to be both highly sensitive and specific for proteolytic degradation of polymerized fibrin (fibrin clot that has been produced in the presence of thrombin). Consequently, this test is being employed with increasing frequency in patients with suspected DIC. However, remembering that thrombin is produced whenever coagulation is activated in the presence of bleeding, the clinician must interpret a modest elevation of D-dimer in a postoperative or trauma patient with some degree of caution. The presence of a marked elevation of D-dimer in a nonbleeding patient essentially excludes primary fibrinogenolysis as the sole cause of measurable FDPs in the serum. The TT is a less sensitive test for DIC, but may be useful in cases of suspected heparin overdose because it corrects in the test tube with the addition of protamine sulfate or toluidine blue. Similarly, the euglobulin clot lysis time may not be sensitive to fibrinolysis associated with DIC but is significantly shortened in most cases of primary fibrinolysis. Other tests of purported value, such as soluble fibrin monomer or thrombin–antithrombin complex formation, either have problems with sensitivity or are impractical for widespread use outside of research settings.
TTP and HUS
Specific mention of TTP and HUS should be made. While neither generally produces a coagulopathic state, both are characterized by marked microangiopathy and microvascular thrombosis. While their similar clinical presentation suggests that these two diseases may represent different ends of the spectrum of end-organ dysfunction possible in microangiopathic states, our improved understanding of the pathophysiology in these two entities confirms that they are not merely differing clinical manifestations of a single-disease entity (39). TTP is characterized by the pentad of microangiopathic hemolytic anemia (MAHA), thrombocytopenia, neurologic symptoms, fever, and renal dysfunction. While only 40% of patients will display the full pentad, up to 75% will manifest a triad of MAHA, neurologic symptoms, and thrombocytopenia. This disorder is felt to be due to the congenital or acquired (often antibody-mediated) absence of a vWf cleaving protease (ADAMTS13) resulting in the circulation of unusually large vWf multimers which can induce or enhance the pathologic adhesion of platelets to the endothelium. The therapy of choice for TTP is plasma exchange by apheresis. While the literature is not consistent regarding routine (prophylactic) platelet transfusions in TTP or HUS, except in the case of major bleeding they are considered contraindicated by many as platelet transfusions have been associated with an increased risk of arterial occlusion including stroke (40–43).
HUS is more commonly seen in children and is characterized by a prodrome of fever and diffuse diarrhea (often bloody). Endemic cases of HUS are generally caused by verotoxin expressing enteropathic strains of Escherichia coli (O157:H7) or shigatoxin expressing strains of Shigella. Sporadic cases are generally not associated with diarrhea and have been associated with congenital or acquired defects in complement factor H (39). Therapy is supportive including renal replacement measures. In contrast to the experience with TTP, plasma infusion and plasma exchange do not generally produce a dramatic improvement in clinical manifestations in typical endemic HUS. While limited data suggests that plasmapheresis/plasma exchange may play a role in the treatment of aHUS, there is no clear consensus regarding how it should be employed (44). Additionally, in limited use, the anticomplement monoclonal antibody eculizumab has also been shown to be beneficial in atypical HUS (39,44).
Management of DIC
The primary treatment for DIC is correction of the underlying problem that led to its development. Specific therapy for DIC should not be undertaken unless the patient has significant bleeding or organ dysfunction secondary to DIC, significant thrombosis has occurred, or treatment of the underlying disorder (i.e., acute promyelocytic leukemia) is likely to increase the severity of DIC.
Supportive therapy for DIC includes the use of several component blood products (45). Packed red blood cells are given according to accepted guidelines in the face of active bleeding. Fresh whole blood (i.e., less than 24 to 48 hours old) also may be given to replete both volume and oxygen-carrying capacity, with the additional potential benefit of providing coagulation proteins, including fibrinogen, and platelets. Fresh frozen plasma (FFP) is of limited value for the treatment of significant hypofibrinogenemia because of the inordinate volumes required to produce any meaningful increase in plasma fibrinogen concentration. Infusion of fibrinogen concentrate, or if concentrate is not available, cryoprecipitate is the preferred product for the correction of significant hypofibrinogenemia (46). FFP infusions, however, may effectively replete other coagulation factors consumed with DIC such as protein C although the increase in these proteins may be quite small unless large volumes of FFP are infused. The use of cryoprecipitate, FFP, or other blood products in the treatment of DIC has, in the past, been open to debate because of concern that these products merely provide further substrate for ongoing DIC and thus increase the amount of fibrin thrombi formed. However, clinical (autopsy) studies have failed to confirm this concern.
The goal of blood component therapy is not to produce normal “numbers” but rather to produce clinical stability. If the serum fibrinogen level is less than 75 to 50 mg/dL, repletion with fibrinogen concentrate or cryoprecipitate to raise plasma levels to 100 mg/dL or higher is the goal. Fibrinogen concentrate dose is calculated based on a formula provided by the manufacturer in the package insert. For cryoprecipitate, a reasonable starting dose is one bag of cryoprecipitate for every 10 kg body weight every 8 to 12 hours. As cryoprecipitate is not a standardized component (i.e., its content varies from bag to bag), one should recheck the fibrinogen level after an infusion to document the increase in fibrinogen level. The amount and timing of the next infusion is then adjusted according to the results. Platelet transfusions also may be used when thrombocytopenia is thought to contribute to ongoing bleeding. Many of the fibrin/fibrinogen fragments produced in DIC have the potential to impair platelet function by inhibiting fibrinogen binding to platelets. This may be clinically significant at the concentration of FDPs achieved with DIC. Platelet transfusions in patients with DIC should be considered to maintain platelet counts up to 40,000 to 80,000/μL depending on the clinical specifics of the patient.
Pharmacologic therapy for DIC has two primary aims: to “turn off” ongoing coagulation so that repletion of coagulation factors may begin, and to impede thrombus formation and ensuing ischemic injury. Several recombinant blood products have been developed which have some potential usefulness in the treatment of DIC. The first of these was recombinant aPC, which was ultimately removed from the market due to lack of significant efficacy. The second new agent for the treatment of severe bleeding, including DIC, is recombinant activated factor VII (rhF.VIIa). While there are limited controlled trials of its use, and none in the pediatric age range with the exception of those patients with acquired inhibitors to F.VIII, it has been shown to be a potent agent for the control of bleeding in a number of medical and surgical settings, including DIC and other consumptive coagulopathies (47–49). This agent has also been shown to correct the hemostatic defect caused by the antiplatelet agents aspirin and clopidogrel (50). However, while control of bleeding can be effected with this agent, no studies have demonstrated a benefit in mortality (51). There have been reports that the use of rhF.VIIa may result in an increase in thrombosis and thromboembolic events, although the incidence appears to be small and the severity of most events mild (52). An additional recombinant agent under investigation is recombinant thrombomodulin. In limited use, it has been shown to decrease the severity of DIC and development of multiorgan failure (53). In addition to aPC, other anticoagulant molecules such as heparin and antithrombin III, and thrombolytic agents continue to be studied as therapy for DIC and sepsis (54,55).
Liver Disease and Hepatic Insufficiency
Abnormal Hemostasis in Liver Disease
Liver disease is a common cause of abnormal hemostasis in ICU patients, with abnormal coagulation studies or overt bleeding occurring in approximately 15% of patients with either clinical or laboratory evidence of hepatic dysfunction. It is a common cause of a prolonged PT or aPTT, often without any clinical sequelae. The hemostatic defect associated with liver disease is multifactorial with multiple aspects of hemostasis affected (56,57).
In liver disease, synthesis of several plasma coagulation proteins is impaired. These include factors II, V, VII, IX, and X. Fibrinogen synthesis by the liver usually can be maintained at levels that prevent bleeding until terminal liver failure supervenes. The physiologic action of fibrinogen synthesized by a diseased liver may not be normal owing to an increased carbohydrate content in its structure (sialic acid) which may result in a diminished hemostatic activity (i.e., a dysfibrinogen). Factor XIII activity also is often decreased in the setting of hepatocellular disease. However, the clinical significance of this decrease in factor XIII is uncertain because levels as low as 3% provide for normal fibrin clot stabilization. Although factor VIII (i.e., factor VIII:C, AHF) is synthesized in the liver, recent data shows it to be in hepatic sinusoidal cells rather than hepatocytes (58). Consequently, synthesis seems to be independent of the state of hepatic function. Indeed, factor VIII levels may be increased in some types of liver disease. Plasma protein C and antithrombin III levels are low in many conditions of hepatic insufficiency, with variable effects.
In addition to these deficiencies in plasma coagulation protein synthesis, many patients with liver disease, particularly cirrhosis, have increased fibrinolytic activity. The mechanism for this heightened fibrinolytic state is not clear but may be related to the increased amounts of plasminogen activator often noted in these patients. It may be difficult to discern whether fibrinolysis occurs solely because of underlying severe liver disease or as a result of concurrent DIC as patients with cirrhosis are at increased risk for the development of DIC. The clinical distinction between primary DIC and a secondary hemostatic defect resulting from liver disease can be virtually impossible to make if active bleeding is present. In liver disease, levels of FDPs can be increased by both increased fibrinolysis and by decreased hepatic clearance. Finally, clinically significant fibrinolysis is a frequent occurrence in patients who undergo portacaval shunt procedures.
Thrombocytopenia may be present to a variable degree in patients with hepatic dysfunction. This is usually ascribed to splenic sequestration. It is rarely profound and generally does not produce clinically significant bleeding as a solitary defect. In vitro platelet aggregation may also be affected, however. Increased plasma concentrations of FDPs are a possible cause of these qualitative platelet abnormalities. The thrombocytopenia of liver disease in conjunction with other coagulation or hemostatic defects secondary to liver disease may result in bleeding that is difficult to manage clinically, particularly if all aspects of the problem are not addressed.
Patients with hepatocellular liver disease may also exhibit decreased synthesis of the vitamin K–dependent anticoagulant proteins, protein C and protein S, as well as antithrombin III (56). Decreased levels of these natural anticoagulants may increase the risk of thrombosis. Neither the PT, aPTT, nor TT will be affected by the levels of any of these naturally occurring anticoagulants.
Presentation
The hemostatic defect in liver disease is multifactorial, and each patient should be approached accordingly. The most common scenario is a patient with liver disease and a prolonged PT without overt bleeding in whom the potential for bleeding is a concern. In patients with liver disease and impaired synthetic capabilities, particularly those who are critically ill, factor VII activity levels are usually the first to decrease due to its short half-life of 4 to 6 hours and increased turnover. This results in a prolonged PT, and can be noted even when usual markers of hepatocellular injury or hepatic insufficiency remain relatively normal (56,57). A prolonged TT in the setting of liver disease may indicate the presence of dysfibrinogenemia as a result of altered hepatic fibrinogen synthesis, or may indicate an acquired defect in fibrin polymerization (e.g., increased FDPs). As the severity of liver disease increases, the aPTT also may be affected, reflecting more severely impaired synthetic function. In this setting, plasma concentrations of the vitamin K–dependent coagulation proteins decrease, as do those of factor V (which is not vitamin K–dependent). Although fibrinogen synthesis occurs in the liver, plasma levels of fibrinogen are generally maintained until the disease approaches end-stage. When fibrinogen levels are severely depressed, liver failure has typically reached the terminal phase. In contrast to the hypofibrinogenemia noted with consumptive coagulopathies, hypofibrinogenemia noted in liver disease is the result of decreased synthesis and is not accompanied by a marked increase in either FDPs or D-dimers.
In more severe forms of liver disease, fibrinolysis may complicate clinical management. The differentiation between concomitant DIC and fibrinolysis attributable to liver disease alone may be difficult (57). The D-dimer assay result should be negative in the patient who has liver disease and elevated FDPs but no active bleeding. Further clinical distinction usually is not possible.
Management
If the patient is not actively bleeding, no specific therapy is required, with certain provisos. In patients with a markedly prolonged PT who are in a postoperative state or are scheduled for an invasive procedure, correction of the PT should be attempted. FFP or a prothrombin complex concentrate (PCC) provides the most immediate source of specific coagulation factors (i.e., factors II, VII, IX, and X). While FFP usually corrects an isolated mild PT prolongation, it is less effective in correcting a more profound prolongation of the PT. Consequently, consensus is moving toward the use of a PCC, preferably a 4-factor PCC (4PCC) as the initial choice to correct a markedly prolonged PT due to the greater amount of F.VII contained in 4PCCs. For this reason, 4PCCs are also preferred over the use of rhFVIIa to correct a prolonged PT (59). Fibrinogen support in the form of fibrinogen concentrate or cryoprecipitate is required if fibrinogen levels are <50 to 100 mg/dL, or if there is documentation of a significant dysfibrinogenemia.. The routine correction of prolonged PT/INR in patients who are to undergo minor invasive procedures has not been shown to affect procedural blood loss (60).
Vitamin K deficiency also is relatively common in this patient population, and replacement may be needed. In contrast to patients with dietary vitamin K deficiency and normal liver function, correction of the PT in vitamin K–responsive critically ill patients typically requires longer than 12 to 24 hours. Patients with significant hepatic impairment may manifest a partial response or may not respond at all. Individuals with severe liver disease, or marked prolonged vitamin K deficiency, will demonstrate a prolongation of the aPTT in addition to a prolonged PT. This prolongation of the aPTT is the result of other clotting factors besides F.VII being low. In this setting, the use of rhFVII a will not provide adequate hemostatic support. If the prolongation of the aPTT is due solely to decreases in the vitamin K–dependent factors (II, VII, IX, X), infusion of a 4PCC is the treatment of choice. However, if other clotting factor deficiency is suspected, plasma (either FFP or commercial solvent/detergent-purified commercial product) is preferred. While recombinant human activated factor VII (rhF.VIIa) infusions have been shown to control the bleeding in severe liver disease, reduced reduction in mortality has not been demonstrated (51,59). The use of rhF.VIIa should be reserved for patients with poorly controlled bleeding that is unresponsive to other more established therapeutic modalities such as infusion of plasma or 4PCCs.
A comprehensive therapeutic approach is needed in the patient with active bleeding as a result of liver disease. Initially, a 4PCC at a dose calculated to increase F.VII to ≥50% of normal (i.e., 0.5 units/mL) should be administered. If a 4PCC is not available, plasma, 10 to 15 cc/kg body weight, may be given. Doses of 4PCC or plasma should be repeated every 6 to 8 hours until bleeding slows significantly, and should then be continued at maintenance levels as dictated by clinical status and coagulation studies. Recombinant human activated factor VII may be used in those patients unresponsive to FFP infusions (60). Cryoprecipitate should be infused for fibrinogen levels <50 to 100 mg/dL. Platelet transfusions also may be required if the platelet count is <40 to 80,000/μL, depending on the clinical situation. Vitamin K should be empirically administered on the presumption that part of the synthetic defect may result from a lack of this cofactor. However, one must anticipate a poor response to vitamin K in the presence of severe liver disease. Transfusions of packed cells are given as deemed appropriate by the clinician.
Vitamin K Deficiency
The most common cause of a prolonged PT in the ICU is vitamin K deficiency. Vitamin K is necessary for the gamma-carboxylation of factors II, VII, IX, and X, without which these factors cannot bind calcium and are not efficiently converted into their activated forms. Factor VII has the shortest half-life of these coagulation proteins; accordingly, the PT is the most sensitive early indicator of vitamin K deficiency.
Vitamin K deficiency is relatively common in critically ill patients for several reasons including the use of broad-spectrum antibiotics, poor nutrition preceding or subsequent to ICU admission, and the use of parenteral nutrition without vitamin K supplementation. Many of the second- and third-generation cephalosporins directly interfere with vitamin K absorption from the gut lumen. The metabolites of some of these antibiotics may even act as competitive inhibitors of vitamin K. In addition, these and other antibiotics may kill or inhibit the growth of gut bacteria and thus limit the amounts of vitamin K they normally produce and excrete into the gut lumen. While malnutrition also may contribute to the development of vitamin K deficiency, this usually requires 1 to 2 weeks to develop in the complete absence of vitamin K intake. However, the use of parenteral alimentation without vitamin K supplementation coupled with antibiotic use may result in rapid vitamin K depletion and prolongation of the PT can occur within only 2 to 3 days. Finally, fat malabsorption states, including cystic fibrosis, may be associated with vitamin K deficiency. Vitamin K is fat soluble and is not absorbed well in some conditions of biliary tract and intrinsic small bowel disease. In the ICU, vitamin K deficiency usually results from the interaction of several of these factors and is rarely limited to one of the conditions mentioned. It is the responsibility of the clinician to maintain an awareness of the potential for vitamin K deficiency and to treat accordingly.
The differential diagnosis of an isolated prolongation of the PT, with or without bleeding, includes both vitamin K deficiency and liver disease. The clinical presentation of these patients is often quite similar. In fact, the distinction sometimes can be made only on the basis of the response (or lack thereof) to empirical vitamin K therapy. Warfarin administration (either overt or covert) also should be excluded as a cause of a prolonged PT. Newer, long-acting vitamin K antagonist (VKA) rodenticides (so-called “super-warfarin”), which, when ingested, produce a profound, prolonged, vitamin K–resistant reduction in vitamin K–dependent clotting factors may produce an isolated prolongation of the PT initially. Treatment of poisoning with these agents requires aggressive prolonged use of vitamin K, and, in the bleeding patient, infusions of FFP or rhF.VIIa. Confirmation of warfarin exposure as the cause of a prolonged PT is possible by toxicologic methods to detect the drug and/or its metabolites, or one can identify the presence of noncarboxylated forms of vitamin K–dependent clotting factors in plasma (proteins induced by vitamin K antagonist; PIVKAs). In addition, the presence of a specific inhibitor or congenital deficiency of factor VII will also result in an isolated prolongation of the PT. Acquired inhibitors of factor VII are rare, and homozygous deficiency of factor VII has not been described. Individuals heterozygous for factor VII deficiency and those with certain polymorphisms of the promoter region of the factor VII gene tend to have factor VII levels in the 25% to 35% range and do not appear to be at significant increased risk for bleeding. Lupus-like anticoagulants resulting from inflammation may also result in an isolated prolongation of the PT; these are generally of no clinical significance and are not associated with an increased risk of bleeding. These patients are not at increased risk for bleeding.
The laboratory findings of an isolated vitamin K deficiency, in addition to a prolonged PT, include a normal fibrinogen level, platelet count, and factor V level. Factor V is not a vitamin K–dependent protein and should therefore be normal except in cases of DIC (consumption) or severe liver disease (decreased production). Prolongation of the aPTT from vitamin K deficiency, warfarin therapy, or from liver disease is a relatively late event and occurs initially as a result of factor IX depletion.
Management
The management of vitamin K deficiency consists primarily of its repletion, usually by intravenous or subcutaneous routes in critically ill patients. Therapy should not await the development of bleeding or oozing but should be administered when the PT abnormality is detected and vitamin K deficiency is thought to be responsible. As with other drugs administered subcutaneously (e.g., insulin), adequate blood pressure and subcutaneous perfusion are needed to ensure reliable absorption from the soft tissues. Concern about the possibility of anaphylactoid reactions with the intravenous use of vitamin K exists. This risk is almost completely negated when the drug is given as a piggyback infusion over 30 to 45 minutes in a small volume of fluid rather than as a bolus or “slow-push” dose. This is the preferred method of drug administration in hemodynamically unstable patients. The usual dose of vitamin K in adults is 10 to 15 mg intravenously or subcutaneously (1 to 5 mg in young children, up to 10 mg in larger children). In an otherwise healthy person, the PT should correct within 12 to 24 hours after this dose. Serial dosing of critically ill patients is often utilized, however, and the PT may require up to 72 hours to normalize. If the PT does not correct within 72 hours after three daily doses of vitamin K, intrinsic liver disease should be suspected. Further administration of vitamin K is of no additional benefit in this setting.
When the patient is actively bleeding, it is not sufficient to give vitamin K alone. A more immediate restoration of coagulation is required. FFP has traditionally been employed in this setting. To restore hemostasis to an acceptable level (30% to 50%) of normal enzyme activity, 10 to 15 cc/kg body weight of FFP is typically required. A similar approach is used in patients previously given warfarin. Recombinant human activated factor VII (rhF.VIIa) has been used with success to reverse the bleeding noted in vitamin K deficiency and in warfarin overdose (60,61).
Massive Transfusion Syndrome
Transfusion of large quantities of blood can result in a multifactorial hemostatic defect. The genesis of this problem is related to the “washout” of plasma coagulation proteins and platelets, and it may be exacerbated by the development of DIC with consequent factor consumption, hypothermia, acidosis or, rarely, by citrate toxicity or hypocalcemia. These variables often act in combination to cause a coagulopathic state (62).
A washout syndrome can result from the transfusion of large amounts of stored blood products devoid of clotting factors and platelets. This develops exclusively in patients who receive large volumes of packed RBCs (e.g., trauma victims, patients with massive gastrointestinal hemorrhage or hepatectomy, or those undergoing cardiopulmonary bypass) without also receiving FFP and platelets. Factors V and VII have short shelf half-lives and are often deficient in blood that has been banked longer than 48 hours. In addition, a qualitative platelet defect can be demonstrated in whole blood within hours of its storage, especially if an acid–citrate–dextrose solution is used. Consequently, transfusion of large quantities of stored whole blood may produce limited benefit in controlling the bleeding resulting from decreased clotting factors and platelets.
Massive transfusion had generally been defined, in adults, as the transfusion of >10 units of RBCs within 24 hours, >4 units of RBs in 1 hour, or >50% blood volume replacement within 3 hours. The development of a washout coagulopathy is directly dependent on the volume of blood transfused relative to the blood volume of the patient. As a general rule, residual plasma clotting activity after one-blood volume exchange falls to 18% to 37% of normal; whereas after a two-blood volume exchange, residual activity is only 3% to 14%; and after a three-blood volume exchange, less than 5% of normal clotting function remains.
As previously discussed, DIC may develop in many clinical settings, including some associated with major hemorrhage or massive transfusion. In the presence of hypotension associated with hypovolemia or hemorrhagic shock, DIC is a common sequela. Major trauma itself, especially with the release of tissue factors into the plasma, also can result in the development of DIC. Exsanguinating hemorrhage sometimes requires blood replacement faster than a type and crossmatch of each unit can be performed, and unmatched blood is given as a lifesaving measure. Donor–recipient incompatibility—even when the mismatch is only of the minor blood group systems—can lead to DIC. Human error resulting in major incompatibility can produce severe hemolysis and be lethal. Finally, microaggregates of blood cells that form within stored blood products also can cause DIC. The advent of smaller pore, more effective filtering systems for blood product administration, however, has essentially eliminated this as a source of problems.
The patient who is bleeding as a consequence of massive transfusion or washout presents with diffuse oozing and bleeding from all surgical wounds and puncture sites. Laboratory abnormalities include prolonged PT, aPTT, and TT. Fibrinogen levels and platelet counts are typically decreased; FDPs are not usually increased unless concurrent DIC is present (see Table 143.2). The likelihood that the clinicolaboratory picture is a direct result of the massive transfusion can be estimated from the amount of bleeding that has occurred and the blood volume administered relative to the patient’s blood volume (i.e., the number of blood volume exchanges that have been given). The more stored blood (e.g., packed RBCs) transfused relative to the patient’s blood volume, the greater the chance of the development of coagulopathy due to massive transfusion.
Management
The therapeutic approach to patients who develop a coagulopathy from massive transfusion is supportive. Platelets and FFP are given to replete the components of coagulation that are typically lacking (40). Platelet administration may help stem bleeding from anatomic wounds. Severe bleeding associated with thrombocytopenia alone is uncommon unless counts fall below 20,000 to 30,000/µL of blood. Because of the complex nature of bleeding seen with massive transfusion, patients may benefit from platelet transfusion at counts even as high as 80,000 to 100,000/µL. FFP is preferred over cryoprecipitate because it has a more complete coagulation protein composition. However, cryoprecipitate may be specifically given when fibrinogen depletion is thought to be a major contributor to the observed bleeding. Over the past 2 to 3 decades, the approach to transfusion support to individuals who require multiple blood transfusions acutely for the treatment of trauma or massive surgical hemorrhage has evolved from a policy of 1 unit of FFP and 1 unit of platelets for every 4 to 5 units of pRBCs transfused to a current 1:1:1 ratio of pRBC:FFP:platelets (63,64). However, the increased patient exposure to plasma with these new transfusion algorithms has also increased the risk for transfusion-related acute lung injury (TRALI) (65). Use of a commercially prepared solvent/detergent virally purified plasma produced form pooled fresh plasma has been shown to result in a decreased risk of TRALI in comparison to that with traditional single-donor plasma, and has also been shown to have a more consistent clotting factor profile than does the various forms of “fresh frozen” plasma available at hospitals (66,67).
Prospective identification of those at risk to develop a coagulopathy from massive transfusion is important. When the magnitude of the insult and the anticipated need for blood are large, both platelets and FFP should be given before a coagulopathy develops. Many institutions have developed infusion algorithms that include activating a “massive transfusion” protocol with their Transfusion Medicine service. These programs have generally resulted in more efficient utilization of blood products in the setting of trauma care (68,69). Use of a higher pRBC:plasma:platelet ratio transfusion regimen should prevent washout and its attendant bleeding. If the patient continues to bleed despite what should be adequate therapy for massive transfusion syndrome, other causes should be considered. Specifically, anatomic bleeding and the possibility of DIC should be investigated. Therapy in this setting may include rhF.VIIa infusion (49).
Anticoagulant Overdose
Anticoagulant therapy is not unusual in the ICU, and the possibility of errors in administration exists. Methods of prophylactic anticoagulant use, systemic anticoagulation, and thrombolytic therapy are sometimes poorly standardized and can lead to overdose.
Heparin
Heparin is a repeating polymer of two disaccharide glycosaminoglycans and is commercially prepared from either porcine intestinal mucosa or bovine lung. Heparin is currently found in two forms, unfractionated heparin (UH) and low–molecular-weight heparin (LMWH). It is important to understand the differences between these two forms of the drug as they have different mechanisms of action and associated precautions. Unfractionated heparin has an immediate effect on coagulation that is mediated primarily through its interaction with antithrombin III. The resulting heparin–antithrombin III complex possesses a much greater affinity for thrombin than does AT III alone and inactivates thrombin, thereby damping-down clot formation. In addition, heparin also has a direct effect inhibiting activated factor X (F.Xa). This anticoagulant effect of UH is relatively minor. Consequently, achieving a therapeutic aPTT with UH is very difficult in the face of low levels of AT III. The degree of anticoagulation produced by heparin has traditionally been monitored by the prolongation of the aPTT. In contrast, LMWH, produced by controlled enzymatic cleavage of heparin polymers, produces anticoagulation almost exclusively through inhibition of F.Xa. This produces a more stable degree of anticoagulation, and due to its longer half-life (approximately 3 to 5 hours) and biologic activity (approximately 24 hours), allows for intermittent bolus therapy (i.e., every 12 or 24 hours) while still maintaining steady-state effect. However, LMWH does not produce consistent prolongation of the aPTT and requires assay of anti-Xa activity for monitoring (if desired). Recently, a therapeutic range for the anti-Xa level achieved with UH infusions has been developed. As most hospital labs now routinely measure anti-Xa levels, some institutions/clinicians monitor UH therapy and adjust doses accordingly.
Heparin is metabolized in the liver by the “heparinase” enzyme in a dose-dependent fashion with excess heparin then being excreted through the kidneys. As the rate of heparin administration is increased, the half-life of the drug is prolonged due the increase in the percentage of the drug being excreted by the kidney. For example, when a 100 U/kg bolus of heparin is infused intravenously, the average half-life of the drug is 1 hour. If the bolus is increased to 400 or 800 U/kg, however, the half-life is prolonged to 2.5 and 5 hours, respectively. The nonlinear response results in greater drug effects on coagulation with smaller dosage increments. When one “re-boluses” or increases a heparin infusion rate in response to insufficient anticoagulation (i.e., inadequate prolongation of the aPTT or anti-Xa level), a point will be reached when further small increments in the heparin infusion rate may result in a substantially greater prolongation of the aPTT. The risk of pathologic bleeding associated with heparin increases when the prolongation of the aPTT is beyond the therapeutic window (generally considered to be 1.5 to 2.5 times the patient’s baseline aPTT, corresponding to a plasma heparin concentration of 0.2 to 0.4 units/mL, or 0.3 to 0.7 anti-Xa units/mL). As a corollary, administration of heparin as a continuous infusion rather than in an intermittent bolus dose regimen is less likely to be associated with pathologic bleeding.
Management
Serious bleeding associated with heparin overdose can be rapidly reversed by protamine sulfate. Protamine binds ionically with heparin to form a complex that lacks any anticoagulant activity. As a general rule, 1 mg of protamine neutralizes approximately 100 U of heparin (specifically, 90 USP units of bovine heparin or 115 USP units of porcine heparin). The dose of protamine needed is calculated from the number of units of active heparin remaining in the patient’s system. This, in turn, is estimated from the original heparin dose and the typical half-life for that infusion rate. The aPTT is used to gauge the residual effects of heparin. Protamine itself potentially has anticoagulant effects, and precautions are necessary during its administration. The drug should be given by slow intravenous push over 8 to 10 minutes. A single dose should not exceed 1 mg/kg (50 mg maximum dose). This dose may be repeated, but no more than 2 mg/kg (100 mg maximum dose) should be given as a cumulative dose without rechecking coagulation parameters. The dose of protamine should always be monitored by coagulation studies. Significant side effects are most commonly seen in situations of overly rapid drug administration and include hypotension and anaphylactoid-like reactions. LMWH is not consistently neutralized by protamine, so invasive procedures should not be performed within 24 hours of administration. Bleeding following LMWH therapy has been treated effectively with rhF.VIIa.
Warfarin
Warfarin and vitamin K are structurally similar in their respective 4-hydroxycoumarin nucleus and naphthoquinone ring. The mechanism of action of warfarin is through inhibition of vitamin K epoxide reductase (VKOR; specifically the C1 subunit VKORC1) which is necessary to maintain a reduced form of vitamin K (vitamin K hydroquinone). Vitamin K hydroquinone is a necessary substrate of the γ-carboxylase which creates the calcium-binding site on the vitamin K–dependent clotting factors (factors II, VII, IX, X). This postsynthetic modification is necessary to produce a calcium-binding site on the molecule which, when occupied, allows for the efficient activation of the zymogen clotting factor into its enzymatically active form. When warfarin is present in sufficient plasma concentrations, there is depletion of reduced vitamin K (vitamin K hydroquinone) and consequent depletion of the active forms of vitamin K–dependent factors.
The PT, or more precisely the international normalized ratio (INR) calculated from the PT, is an accurate indicator of the intensity of anticoagulation with warfarin when its use has continued beyond 2 or 3 days. Factor VII has a half-life of only 4 to 6 hours and (the active form) is rapidly depleted after one or two doses of warfarin. The remainder of the vitamin K–dependent factors may take up to a week to become depleted. The PT becomes prolonged, and INR elevated, with factor VII depletion alone but does not reflect an overall state of anticoagulation until an equilibrium period of several days has passed. Over this time, the other vitamin K–dependent factors are depleted and PT prolongation (INR elevation) can then be used to assess the anticoagulant effects of warfarin. In severe cases of warfarin overdose, the aPTT also becomes prolonged as a result of depletion of the active forms of factors II, IX, and X. While the INR is calculated from the PT and is frequently used as a surrogate for the PT and in this manner used to assess bleeding risks, the INR has only been validated as an indicator of anticoagulant intensity with VKA therapy. While some retrospective studies have identified an INR 1.5 as being associated with an increased risk of bleeding, INR alone has not been shown to be a consistent indicator of bleeding risk with invasive procedures, in liver disease or other medical conditions (70–72).
Several drugs and pathophysiologic conditions are associated with potentiation of warfarin’s effects on coagulation. Table 143.5 lists many of the drugs known to prolong the effects of warfarin. These drugs have a variety of mechanisms, which generally include either inhibition of function or competitive binding of the enzymes responsible for active warfarin metabolism. Aspirin does not seem to have any direct influence on warfarin metabolism but can so profoundly influence qualitative platelet function that it must be considered as a potentiator of warfarin’s anticoagulant effects. The same is true for clofibrate. Ingestion of large quantities of aspirin may also impair prothrombin (factor II) synthesis, further increasing the effects of warfarin administration. Warfarin is metabolized by the liver. Conditions of acute and chronic hepatic dysfunction can alter warfarin metabolism and vitamin K–mediated γ-carboxylation of the vitamin K–dependent coagulation proteins. Broad-spectrum antibiotics also may limit vitamin K availability through their alteration of the gut flora (in addition to any direct effect on vitamin K metabolism). All of these factors may ultimately influence a patient’s response to warfarin.
A clinical syndrome referred to as “warfarin (coumadin) necrosis” has been noted during the initial stages of anticoagulation with a VKA. It is characterized clinically by the development of skin and subcutaneous necrosis, particularly in areas of subcutaneous fat, and pathologically by the thrombosis of small blood vessels in the fat and subcutaneous tissues. This syndrome is caused by the rapid depletion of the vitamin K–dependent anticoagulant protein C prior to achieving depletion of procoagulant proteins and occurs predominantly in individuals heterozygous for protein C deficiency. While anticoagulation generally requires a decrease in procoagulant protein levels to approximately 20% to 25%, a prothrombotic milieu is created with protein C levels of 40% or less. Consequently, individuals who are heterozygous for protein C deficiency and have baseline protein C levels of 50% to 60% may develop a prothrombotic environment during the first few days of warfarin therapy. The risk of developing warfarin necrosis appears to be greater when an initial dose of warfarin greater than 10 to 15 mg is administered. The development of this syndrome generally can be avoided if heparin and warfarin therapy are overlapped until “coumadinization” is complete, and if large loading doses of warfarin are avoided.
TABLE 143.5 Drugs That Potentiate the Anticoagulant Effects of Warfarin |
![]() |
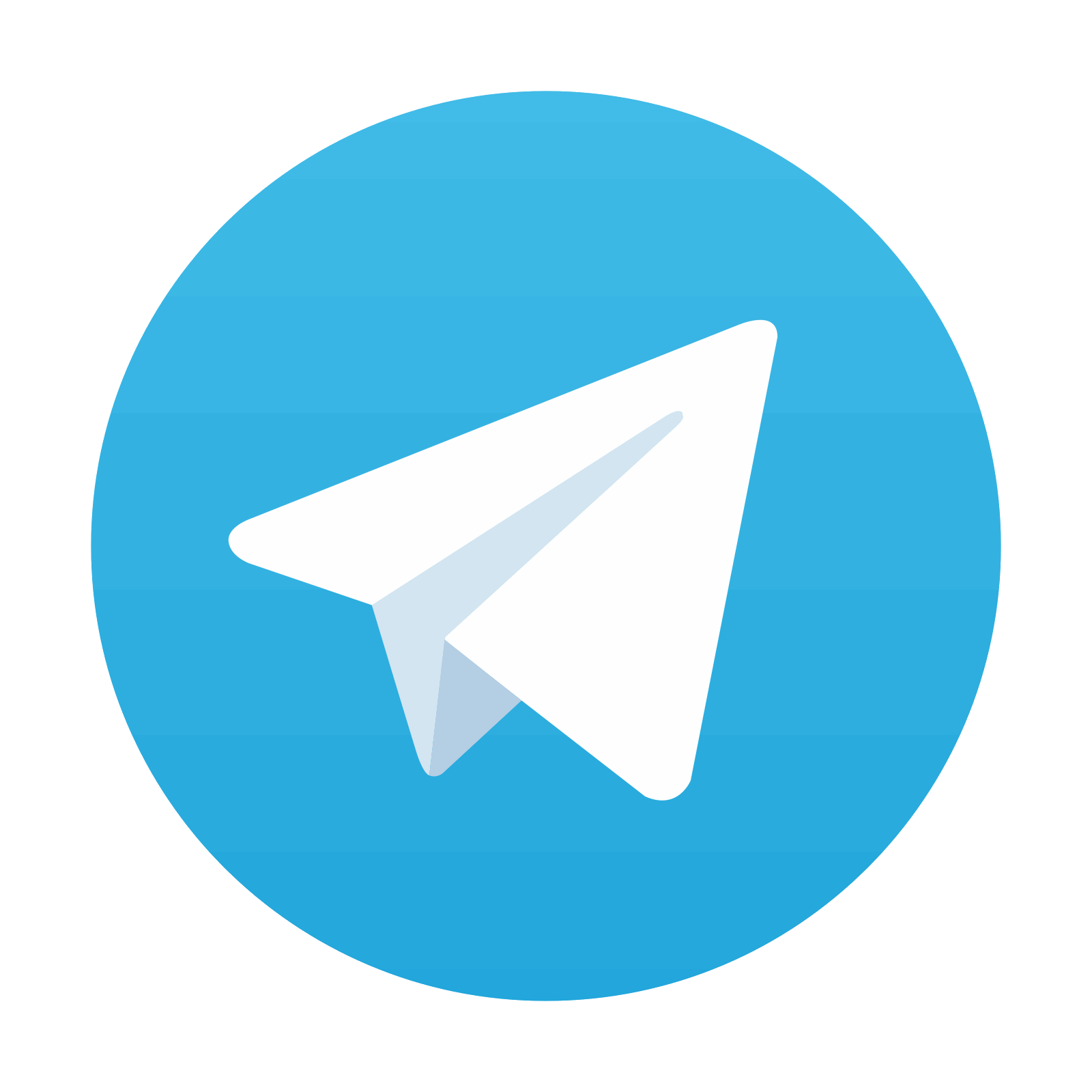
Stay updated, free articles. Join our Telegram channel

Full access? Get Clinical Tree
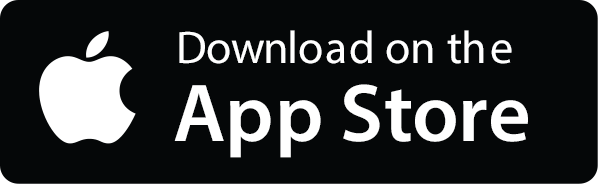
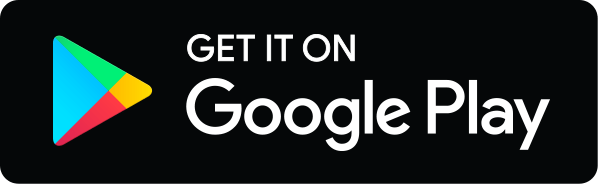