Clinical Pharmacology of Local Anesthetics
John F. Butterworth IV
A review of the clinical pharmacology of local anesthetics must consider those factors that influence the usefulness and toxicity of the drugs as a group, as well as those factors specific to each compound. The various local anesthetics available commercially differ markedly in their clinical applications and potential for toxicity. In clinical practice, not every local anesthetic is suitable for every regional block procedure. Thus, an astute clinician will select among a restricted set of compounds for that one with the onset and duration of action most consistent with surgical needs. The chemical structures of the various agents and the basic pharmacological differences between the ester and amide compounds have been presented in the preceding two chapters and will not be reported herein. This information will not be repeated here. The clinical profiles of the commonly available agents are summarized in Table 4-1.
Local anesthetic toxicity may take the form of central nervous system (CNS) activation (or depression), cardiac arrhythmias or depressed cardiac inotropy, local irritant actions including neurotoxicity and myotoxicity, allergy, or miscellaneous agent-specific reactions such as methemoglobinemia (more common with benzocaine and prilocaine) and addiction (cocaine). Cardiovascular and CNS toxicity is considered in Chapter 5. The remaining forms of toxicity (save for addiction) will be considered in this chapter.
Factors that Influence Local Anesthetic Activity
In humans, the potency, onset, and duration of anesthesia have strong statistical associations with the physicochemical properties and inherent vasodilator activites of the various agents. With most agents, onset, duration, and adequacy of anesthesia may be altered by drug dosage and/or addition of vasoconstrictors. The site of injection has a profound influence on the potency, onset, duration and adequacy of anesthesia of a specific local anesthetic dose. Attempts have been made to alter the onset and duration of anesthesia by using mixtures of local anesthetics, carbonation (adding carbon dioxide), or addition of bicarbonate (NaHCO3) or any of a long list of other additives to local anesthetic solutions.
Physicochemical Properties
Important physicochemical properties that associate with measures of local anesthetic activity include lipid solubility, protein binding, and pKa. Measurements of these properties for the various local anesthetic compounds are provided in Table 3-1.
Lipid solubility has a strong association with the potency of local anesthetic compounds (Fig. 4-1), particularly when chemically similar compounds are compared. Procaine and chloroprocaine, agents of low lipid solubility, have octanol-to-buffer partition coefficients for their free-base neutral form of 100 and 810, respectively, at 36°C. These drugs are typically administered at concentrations of 2% to 3% to attain effective conduction anesthesia in humans. On the other hand, tetracaine, bupivacaine, and etidocaine, compounds of increased lipid solubility, have octanol-to-water partition coefficients for their free-base forms of 5,822; 3,420; and 7,317, respectively, at 37°C (1). Reflecting greater potency, these agents produce effective anesthesia at concentrations between 0.25% to 1.5%. Note, however, that the agent with the greatest free base octanol-to-buffer concentration ratio (etidocaine) is typically applied in greater concentrations than either tetracaine or bupivacaine. Lidocaine and mepivacaine are intermediate both in terms of lipid solubility (partition coefficients of about 366 and 130, respectively) and of their anesthetic potency in vivo (typical anesthetic concentration of 1%–2%).
A consistent correlation exists between lipid solubility and anesthetic potency when similar compounds are applied to an isolated nerve in vitro. Thus, there is good correlation among potency and solubility when mepivacaine, ropivacaine, and bupivacaine are compared or when procaine and tetracaine are compared. However, the correlation between lipid solubility and anesthetic potency is less consistent in humans owing to other biologic considerations that exist in vivo but not in an in vitro preparation (Fig. 4-1) (2).
Lipid solubility also associates with duration of action. For unknown reasons, many books and review articles overlook this association. Among certain pairs of related anesthetics—etidocaine and lidocaine, mepivacaine and bupivacaine, and procaine and tetracaine—in every case, the agent with greater lipid solubility will have the more prolonged duration of action. We will consider this association when we discuss protein binding. Increased lipid solubility also associates with increased delay of onset for every drug comparison just cited, save that of etidocaine versus lidocaine, in which etidocaine has at least as fast an onset as lidocaine. Etidocaine’s anomalously rapid onset remains poorly understood.
All clinically useful compounds must have at least some minimal lipid solubility. The protonated (charged) forms of local anesthetics have much lower octanol-to-buffer partition coefficients than the neutral (uncharged) forms (1). At 36°C, for bupivacaine, the charged local anesthetic has a coefficient of 2, whereas the uncharged base local anesthetic has a coefficient of
3,420. Compounds that do not readily permeate membranes (e.g., QX314, an obligatorily charged quaternary analog of lidocaine) will produce nerve block only after a prolonged delay when applied on the extracellular side of a nerve, as would take place during clinical regional anesthesia. Obligatorily charged local anesthetics will potently block sodium (Na) currents when applied within cytoplasm (3).
3,420. Compounds that do not readily permeate membranes (e.g., QX314, an obligatorily charged quaternary analog of lidocaine) will produce nerve block only after a prolonged delay when applied on the extracellular side of a nerve, as would take place during clinical regional anesthesia. Obligatorily charged local anesthetics will potently block sodium (Na) currents when applied within cytoplasm (3).
Table 4-1 Commonly used local anesthetics | ||||||||||||||||||||||||||||||||||||||||||||||||||||||||||||||||||||||||||||||||||||||||||||||||||||||||||||||||||||||||||||||||||||||||||||||||||||||||||||||||||||||||||||||||||||||||||||||||||||||||||||||||||||||||||||||||||||||||||||||||||||||||||||||||||||||||||||||||||||||||||||||||||||||||||||||||||||||||||||||||||||||||||||||||
---|---|---|---|---|---|---|---|---|---|---|---|---|---|---|---|---|---|---|---|---|---|---|---|---|---|---|---|---|---|---|---|---|---|---|---|---|---|---|---|---|---|---|---|---|---|---|---|---|---|---|---|---|---|---|---|---|---|---|---|---|---|---|---|---|---|---|---|---|---|---|---|---|---|---|---|---|---|---|---|---|---|---|---|---|---|---|---|---|---|---|---|---|---|---|---|---|---|---|---|---|---|---|---|---|---|---|---|---|---|---|---|---|---|---|---|---|---|---|---|---|---|---|---|---|---|---|---|---|---|---|---|---|---|---|---|---|---|---|---|---|---|---|---|---|---|---|---|---|---|---|---|---|---|---|---|---|---|---|---|---|---|---|---|---|---|---|---|---|---|---|---|---|---|---|---|---|---|---|---|---|---|---|---|---|---|---|---|---|---|---|---|---|---|---|---|---|---|---|---|---|---|---|---|---|---|---|---|---|---|---|---|---|---|---|---|---|---|---|---|---|---|---|---|---|---|---|---|---|---|---|---|---|---|---|---|---|---|---|---|---|---|---|---|---|---|---|---|---|---|---|---|---|---|---|---|---|---|---|---|---|---|---|---|---|---|---|---|---|---|---|---|---|---|---|---|---|---|---|---|---|---|---|---|---|---|---|---|---|---|---|---|---|---|---|---|---|---|---|---|---|---|---|---|---|---|---|---|---|---|---|---|---|---|---|---|---|---|---|---|---|---|---|---|---|---|---|---|---|---|---|---|---|---|---|---|---|
|
The pKa of a chemical compound identifies the pH at which the ionized and nonionized forms are present in equal concentrations. In the case of local anesthetics, pKa has a much-discussed, putative association with the speed of onset of anesthesia (4,5). For any local anesthetic that must permeate membrane barriers, rapid onset of action is favored by increasing amounts of drug in the base (uncharged or neutral) form. The percentage of a specific local anesthetic that is present in the base form when injected into tissue is inversely proportional to the pKa of that agent. As a consequence, many reviewers assert that one can predict the relative speed of onset among differing local anesthetics by comparing their pKas (5). This rule is not valid, despite the large number of examination questions that have been written on this topic! Mepivacaine, lidocaine, and etidocaine, for example, possess pKas of 7.7, 7.8, and 7.9, respectively, at 36°C (1). When these agents are injected into tissue at a pH of 7.4, roughly 65% of these drugs exist in the ionized form and 35% in the nonionized base form. Yet, the onset of block with etidocaine is at least as fast as with the other two agents. In a similar way, tetracaine possesses a pKa of 8.4 at 36°C. Thus, roughly 5% is present in the nonionized form and 95% exists in the charged cationic form at a tissue pH of 7.4. At the same temperature, chloroprocaine has a pKa of 9.1, meaning that only 2% will be in the free-base form and 98% will be protonated. Nevertheless, the onset of block with chloroprocaine for all forms of regional anesthesia is considerably faster than with tetracaine (and this holds true even when adjustments are made for their relative potencies). The pKas of the various local anesthetic agents are provided in Table 3-1. Those who wish to preserve the pKa “rule” often argue that chloroprocaine is used at greater concentrations and dosages than other local anesthetics, and that the more rapid onset of action could be related simply to the larger number of molecules of this agent that can be safely administered compared to other agents. This explanation collapses when 1% chloroprocaine and 1% lidocaine are compared for spinal anesthesia (Fig. 4-2) (6). Chloroprocaine has a faster
onset time than lidocaine. Thus, pKa is not nearly as predictive of rate of onset as generations of anesthesiologists have been led to believe.
onset time than lidocaine. Thus, pKa is not nearly as predictive of rate of onset as generations of anesthesiologists have been led to believe.
Many authors have opined that the relative protein binding of various local anesthetics has an influence on their duration of anesthesia. Various unwieldy, logically inconsistent, and almost certainly incorrect explanations have been offered for this association. Nevertheless, despite the corellation, no direct relationship exists between local anesthetic protein binding and local anesthetic binding to Na channels. Would any credible pharmacologist argue that a relationship exists between the greater (nonspecific) protein binding of sufentanil versus fentanyl and (specific) binding of these agents to μ-receptors in brain and spinal cord? Certainly not! For all classes of drugs, the less water-soluble the compound the greater fraction of the drug will be protein bound in blood. The only conceivable connection between protein binding and duration of local anesthetic action lies in the fact that local anesthetics of increased lipid solubility (by definition) are protein bound to greater extent when they reach the bloodstream. For thermodynamic reasons, more lipid-soluble agents will have a greater tendency than less lipid-soluble agents to remain in a lipid-rich environment (e.g., the plasma membrane) than diffuse into the blood. The longer that the local anesthetic molecule remains within the membrane (rather than diffusing away from the nerve toward the bloodstream), the longer that the molecule has the potential to bind Na channels and produce nerve block. Once the local anesthetic enters the bloodstream, it is highly unlikely to reenter the nerve membrane and contribute to conduction block.
Bupivacaine is about 95% protein bound (and has an octanol-to-buffer partition coefficient for the free-base form of 3,420) and is a very potent anesthetic with a long duration of action (1). On the other hand, procaine, which is only 6% protein bound (and has an octanol-to-buffer partition coefficient for the free-base form of 100), is less potent and has a relatively short duration of action. Mepivacaine and lidocaine, which are intermediate in terms of protein binding (55%–75%), are also intermediate in terms of lipid solubility (partition coefficients for the free-base forms of 130 and 366, respectively), potency, and anesthetic duration. As should be obvious, it is erroneous to consider the nonspecific binding of a drug to α1-acid glycoprotein and albumin as having any direct relationship to the binding of that drug to its specific binding site in membrane-spanning region S6 of domains III and IV on the Na channel (7).
Vasodilator Properties
The clinical activity of local anesthetics is also modified by other factors. Faster vascular absorption reduces the number of local anesthetic molecules available for binding to Na channels. Faster absorption into the bloodstream may also reduce the apparent local anesthetic in vivo potency and duration of action. All local anesthetics except cocaine exhibit a biphasic effect on vascular smooth muscle (8,9). At extremely low concentrations, they inhibit nitric oxide release in vascular smooth muscle, leading to vasoconstriction. This action is of unknown clinical importance because at the much greater concentrations generally used for regional anesthesia local anesthetics tend to be vasodilators (10). Differences in the relative degree of vasodilation produced by local anesthetics may influence their anesthetic profile. For example, some in vitro studies have shown that lidocaine is significantly more potent than mepivacaine on an isolated nerve (consistent with their octanol-to-water partition coefficients) (4). Human studies, however, show little difference in the relative anesthetic potency of these two agents and sometimes find that the duration of action of mepivacaine is longer than that of lidocaine (11). Perhaps these differences relate to greater vasodilation by lidocaine: Addition of epinephrine to lidocaine and mepivacaine reduces both the difference in vasodilator activity and the duration of action (11).
In summary, the clinically important properties of local anesthetics include potency, delay of onset, and duration of action. Associations exist between the clinical profiles of the various agents and their physicochemical properties. In vivo, the clinical activity of these drugs may be altered by other actions, such as their relative tendency to produce vasodilation. On the basis of their anesthetic profile in humans, the local anesthetics may be classified as follows:
Agents of low potency and short duration of action, including procaine and chloroprocaine
Agents of intermediate potency and duration of action, including lidocaine, mepivacaine, and prilocaine
Agents of high potency and prolonged duration of action, including tetracaine, bupivacaine, ropivacaine, and etidocaine
In terms of onset, chloroprocaine, lidocaine, mepivacaine, prilocaine, and etidocaine possess a relatively rapid onset of action. Tetracaine, bupivacaine, and ropivacaine have longer
latencies of onset (except during spinal anesthesia, during which all anesthetics produce a relatively fast onset of block). In general, increasing potency associates with increasing lipid solubility, protein binding, delay of onset, and duration of action.
latencies of onset (except during spinal anesthesia, during which all anesthetics produce a relatively fast onset of block). In general, increasing potency associates with increasing lipid solubility, protein binding, delay of onset, and duration of action.
In addition to these properties, one other important clinical consideration is the ability of local anesthetic agents to differentially inhibit sensory versus motor fibers. Physicians have long known that nerve fibers of differing sizes have differing susceptibility to local anesthetics (as well as to pressure, lack of oxygen, and lack of glucose). In general, among fibers of similar types, the larger the fiber, the more resistant it is to local anesthetic block (12). Smaller myelinated fibers (e.g., Aδ-fibers) are more susceptible to local anesthetics than are larger myelinated fibers (e.g., Aα- or Aβ-fibers). And, larger unmyelinated fibers are less susceptible to block than are smaller unmyelinated fibers. The “size principle” fails when unmyelinated fibers are compared with myelinated fibers. A “pure” differential block of smaller pain fibers cannot be achieved, however, because the unmyelinated fibers (e.g., C-fibers) as a group are less susceptible to local anesthetics than are the myelinated fibers (13). Thus, using conventional local anesthetic techniques, one cannot completely block all pain-transmitting Aδ- and C-fibers without also producing some inhibition of motor fibers. Although differential spinal blockade has been produced with reduced intrathecal doses of procaine and used to identify sympathetically mediated pain states of the lower extremities, local anesthetics will not produce surgical analgesia for incision without motor block. Physicians can also use mixtures of local anesthetic and other agents to produce postoperative analgesia via epidural and peripheral neural infusions. Again, these techniques cannot produce analgesia sufficient for surgical incision without motor block.
Bupivacaine and etidocaine, two potent, long-acting local anesthetics introduced into clinical practice at about the same time, provide an interesting contrast in terms of their differential sensory/motor blocking activity. Bupivacaine is widely used in epidural solutions for surgical anesthesia, for obstetric analgesia, and for relief of postoperative pain owing to its ability to provide adequate sensory analgesia while preserving motor function, particularly at concentrations ≤0.25%. Thus, a laboring parturient can be rendered pain-free yet still be able to move her legs (or even walk) (4). Etidocaine, on the other hand, shows little separation between sensory and motor blockade. To achieve adequate epidural sensory anesthesia, 1.5% concentrations of etidocaine are usually required. At these concentrations, etidocaine has an extremely rapid onset of action and a prolonged duration of anesthesia; however, sensory anesthesia is associated with a profound degree of motor blockade, and the motor block can sometimes outlast the sensory block during offset of anesthesia. Thus, etidocaine was useful for surgical epidural anesthesia in which optimum muscle relaxation is desirable, whereas its marked inhibition of motor function rendered it useless for obstetric or postoperative epidural analgesia.
As previously noted, bupivacaine was the first agent that showed some relative specificity for sensory fibers, such that sensory analgesia without profound inhibition of motor fibers often could be observed (14). Unfortunately, the success was incomplete. When the sensory block was adequate for skin incision, there was almost always a significant degree of motor block. Differences among local anesthetics are sometimes apparent during the onset or offest of peripheral nerve block. For example, during the onset of median nerve block with mepivacaine, there is almost no difference in the relative inhibition of sensory nerve function as assessed by the amplitude of sensory nerve action potentials (SNAPs) recorded from volunteer subjects versus inhibition of motor nerve function as assessed by compound motor action potential (CMAP) amplitude (15). With bupivacaine, the inhibition of SNAP amplitude occurred earlier and was more profound than CMAP. At steady state, both agents inhibited SNAPs and CMAPs comparably and profoundly (Fig. 4-3) (15).
Other Factors Influencing Anesthetic Activity
Although the inherent pharmacologic properties of the various local anesthetic agents largely determine their anesthetic profile, other factors may influence the quality of regional anesthesia, including (a) dosage of local anesthetic administered, (b) addition of a vasoconstrictors and other additives to the local anesthetic solution, (c) site of administration, (d) the temperature of the local anesthetic solution, (e) pregnancy, and (f) mixtures of local anesthetic solutions.
Dosage of Local Anesthetic Solutions
The mass of drug (the total number of local anesthetic molecules) administered will influence the onset, quality, and duration of anesthesia (Table 4-2) (4). For any agent, as the dose is increased, the likelihood of satisfactory anesthesia and the duration of anesthesia will increase, and the latency of onset of anesthesia will decrease. In general, the dosage of local anesthetic administered can be increased by administering a larger volume of a less concentrated solution or a smaller volume of a more concentrated solution. For example, a dose-response study of bupivacaine for epidural analgesia in laboring women showed that increasing the concentration from 0.125% to 0.5% while maintaining the same injectate volume (10 mL) decreased latency, improved the incidence of satisfactory analgesia, and increased the duration of sensory analgesia (16). A study of bupivacaine in surgical anesthesia showed that increasing the concentration from
0.5% to 0.75% (increasing the dose from about 100 mg to 150 mg) produced a faster onset and longer duration of sensory anesthesia (17). In addition, the likelihood of satisfactory sensory anesthesia and the degree of motor blockade was increased. When prilocaine (600 mg) was administered in the epidural space either as 30 mL of a 2% solution or 20 mL of 3% solution, there was no difference in onset, adequacy, or duration of anesthesia and onset, depth, and duration of motor blockade (18). In some blocks, the volume of anesthetic solution administered may influence the “spread” of anesthesia; for example, 30 mL of 1% lidocaine administered in the epidural space anesthetized four more dermatomes than did 10 mL of 3% lidocaine (19). On the other hand, some animal studies suggest that a smaller volume of a more concentrated local anesthetic solution produces a denser, more persistant block than a larger volume of a less conventional solution (20). Nevertheless, the consensus from clinical studies suggests that, except for the possible effect on the dermatomal spread of epidural anesthesia, the primary qualities of regional anesthesia, namely, onset, depth, and duration of blockade, are related to the mass of drug injected; that is, the product of volume times concentration, and the proximity of the local anesthetic molecules to the intended target.
0.5% to 0.75% (increasing the dose from about 100 mg to 150 mg) produced a faster onset and longer duration of sensory anesthesia (17). In addition, the likelihood of satisfactory sensory anesthesia and the degree of motor blockade was increased. When prilocaine (600 mg) was administered in the epidural space either as 30 mL of a 2% solution or 20 mL of 3% solution, there was no difference in onset, adequacy, or duration of anesthesia and onset, depth, and duration of motor blockade (18). In some blocks, the volume of anesthetic solution administered may influence the “spread” of anesthesia; for example, 30 mL of 1% lidocaine administered in the epidural space anesthetized four more dermatomes than did 10 mL of 3% lidocaine (19). On the other hand, some animal studies suggest that a smaller volume of a more concentrated local anesthetic solution produces a denser, more persistant block than a larger volume of a less conventional solution (20). Nevertheless, the consensus from clinical studies suggests that, except for the possible effect on the dermatomal spread of epidural anesthesia, the primary qualities of regional anesthesia, namely, onset, depth, and duration of blockade, are related to the mass of drug injected; that is, the product of volume times concentration, and the proximity of the local anesthetic molecules to the intended target.
Table 4-2 Effects of dose and epinephrine on local Anesthetic procedures | |||||||||||||||||||||
---|---|---|---|---|---|---|---|---|---|---|---|---|---|---|---|---|---|---|---|---|---|
|
Use of Additives with Local Anesthetic Solutions
Vasoconstrictors, typically epinephrine, are frequently added to local anesthetic solutions. Vasoconstrictors decrease the rate of vascular absorption, allowing more anesthetic molecules to reach the nerve membrane, improving depth and prolonging duration of anesthesia (Table 4-2). In clinical anesthesia, local anesthetic solutions usually contain a 1:200,000 (5 μg/mL) concentration of epinephrine, based on studies of lidocaine in epidural and intercostal blocks (4,21). Only limited information is available regarding the optimum concentration of epinephrine with other local anesthetic agents or other block procedures. Other α-agonists, such as clonidine and phenylephrine, have been used as additives to solutions of local anesthetics.
In some studies, phenylephrine produced greater prolongation of spinal anesthesia when combined with tetracaine; however, more recent studies conducted under double-blind conditions found no difference between epinephrine and phenylephrine in prolonging tetracaine spinal anesthesia (Fig. 4-4) (22). Epinephrine has differing effects on specific local anesthetics. Procaine, lidocaine, and mepivacaine are significantly prolonged by epinephrine during infiltration anesthesia, peripheral nerve blocks, or epidural anesthesia (11,23,24,25,26). Bupivacaine and etidocaine local infiltration blocks are prolonged by epinephrine (27). The durations of epidural blocks with these agents are not, however, markedly prolonged by epinephrine (24,27,28). It is generally assumed that with bupivacaine and etidocaine the increased lipid solubility and delayed uptake of these agents by the bloodstream may underlie the diminished effect of epinephrine.
The interaction of epinephrine with the long-acting agents, such as bupivacaine, is dependent on the setting, block technique, and concentration of drug used. In epidural analgesia for labor, for example, the frequency and the duration of adequate analgesia were improved when epinephrine 1:200,000 was added to 0.125% and 0.25% bupivacaine (16); however, the addition of epinephrine to 0.5% and 0.75% bupivacaine did not significantly improve epidural blocks in either obstetric or surgical patients (16,29). Epinephrine appears to improve the quality of analgesia provided by dilute intrathecal solutions of bupivacaine plus opioid (30). The degree of motor blockade is increased following the epidural administration of epinephrine-containing solutions of bupivacaine and etidocaine (29). The differing effects of epinephrine in prolonging the duration of differing local anesthetics is most apparent during spinal anesthesia (see Chapter 10). Epinephrine greatly increases the duration of tetracaine spinal anesthesia, but prolongs lidocaine and bupivacaine spinal anesthesia to a lesser extent (22,31,32,33,34).
Attempts have been made to modify local anesthetic solutions to shorten their delay of onset and/or to prolong their duration of anesthesia. Carbonation of local anesthetic solutions was thought to speed the onset of action of various local anesthetics (14,35). In isolated nerves, carbon dioxide enhances diffusion of local anesthetics through nerve sheaths
and hastens inhibition of action potentials (36,37). Double-blind studies, however, failed to demonstrate a significantly more rapid onset of action when lidocaine carbonate was compared with lidocaine hydrochloride for epidural blockade (38). In fact, addition of NaHCO3 to lidocaine (which would be expected to reduce the fraction of the protonated local anesthetic form) reduced the onset delay relative to the carbonated prepration (39). Other double-blind studies failed to show benefit from carbonation of bupivacaine (40,41). Thus, at present, there appears to be no benefit to carbonation of local anesthetic solutions in terms of onset of block under clinical conditions.
and hastens inhibition of action potentials (36,37). Double-blind studies, however, failed to demonstrate a significantly more rapid onset of action when lidocaine carbonate was compared with lidocaine hydrochloride for epidural blockade (38). In fact, addition of NaHCO3 to lidocaine (which would be expected to reduce the fraction of the protonated local anesthetic form) reduced the onset delay relative to the carbonated prepration (39). Other double-blind studies failed to show benefit from carbonation of bupivacaine (40,41). Thus, at present, there appears to be no benefit to carbonation of local anesthetic solutions in terms of onset of block under clinical conditions.
As mentioned, attempts have been made to speed the onset of conduction blockade by adding NaCHO3 to local anesthetic solutions immediately before injection (39,42,43,44). Sodium bicarbonate will increase the pH of the local anesthetic solution, which in turn will increase the amount of drug in the uncharged base form. Thus, the rate of diffusion across the nerve sheath and nerve membrane could be increased, speeding the onset of anesthesia. In vitro studies of pH adjustment suggest that the apparent potency of local anesthetics increases at more basic pH (Fig. 4-5) (45). Addition of NaCHO3 to lidocaine prior to median nerve block decreased the latency of onset of block of compound motor action potentials, but had no effect on the latency of block of sensory nerve action potentials or on inhibition of pin-prick sensibility (46). Numerous clinical studies have been performed in which the addition of NaCHO3 to local anesthetic solutions has had a variable effect on the latency, duration, or effectiveness of blockade (Fig. 4-6) (5,44). Overall, it appears that NaCHO3 has its greatest benefit when added to local anesthetic solutions mixed with epinephrine by the manufacturer. These solutions tend to have a reduced pH (relative to “plain” solutions of local anesthetics) that increases the shelf life of the epinephrine. Bicarbonate would be expected to have a lesser effect on solutions that are less acidic to begin with, and most studies of this topic bear this out (44). Addition of NaCHO3 consistently reduces the pain of injection of local anethetics (47).
Potassium occasionally has been added to local anesthetic solutions in unsuccessful attempts to improve local anesthesia (48,49). Various attempts have been made to prolong the duration of anesthesia by incorporating dextran into local anesthetic solutions (50,51). In one clinical study, prolonged durations of anesthesia were observed in occasional patients, but the mean duration of intercostal nerve blockade was not significantly altered when solutions of bupivacaine with and without dextran were compared (52). Rosenblatt and Fung have suggested that the difference in results obtained by various investigators may be related to the pH of the dextran solution used with more alkaline dextran solutions producing prolongation and less alkaline dextrans being ineffective (53). Perhaps alkalinization of the anesthetic solution may be responsible for prolonged conduction blockade rather than the dextran itself.
There has been much recent interest in addition of the α2-agonist clonidine to local anesthetic solutions. Clonidine has local anesthetic properties in vitro: Clonidine and other similar agents will block both Aα- and C-fibers (Fig. 4-7) (54). Thus, it remains unclear whether prolongation of local anesthesia by clonidine is the result of pharmacodynamic prolongation of local anesthetic effects, a direct action of clonidine on nerves, a central action of clonidine, or some combination of effects (55). When added to intermediate-duration agents such as mepivcaine and lidocaine, clonidine markedly prolongs the duration of brachial plexus or femoral nerve blocks. Clonidine prolongs the duration of spinal anesthesia when it is included with mepivacaine. Clonidine even prolongs the duration of tetracaine when clonidine is taken as an oral agent (56,57). Clonidine, like epinephrine, appears to be less useful at prolonging the duration of such agents as bupivacaine or ropivacaine (58).
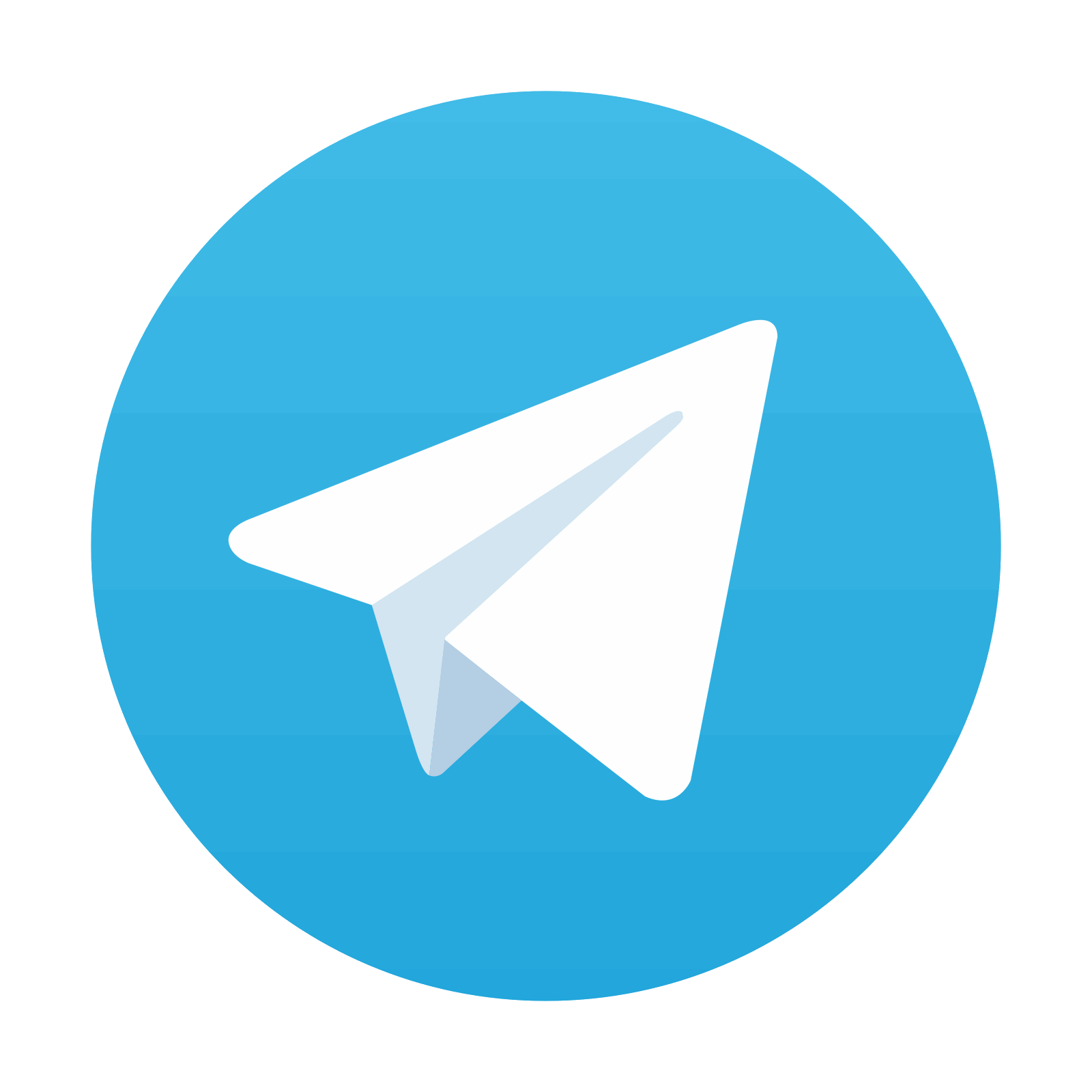
Stay updated, free articles. Join our Telegram channel

Full access? Get Clinical Tree
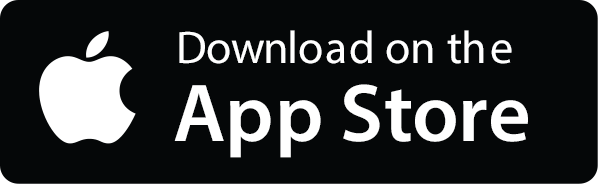
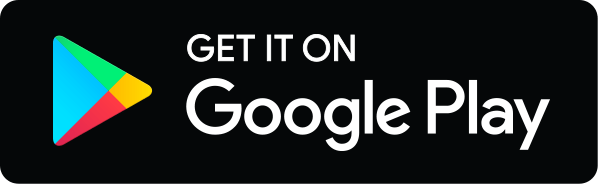