Clinical Measurement and Monitoring
Clinical measurement is limited by four major constraints:
Feasibility of measurement. The sensitivity and inherent variability of a clinical measurement depend on complex interactions and technical difficulties at the biological interface between the patient and the instrument.
Reliability of measurements is determined by the properties of the measurement system. This is influenced by the calibration and correct use of the instrument. Simple examples include the correct placement of ECG electrodes, or the appropriate size of cuff for non-invasive measurement of arterial pressure. Delicate equipment, e.g. a blood gas analyser, requires regular maintenance and calibration.
Interpretation depends on the critical faculties of the anaesthetist who interprets the significance of measurements in the context of complex physiological systems. Arterial pressure may be within the normal range despite severe hypovolaemia or derangement of cardiovascular function within the limits of physiological compensation. Global measurements of end-tidal carbon dioxide tension or oxygen saturation are influenced by many factors in a highly complex system. More information is required to deduce the cause of a change in the measurement.
Value of clinical measurements in patient care is defined by the role of a measurement in improving patient care. This includes the ease, convenience, continuity and usefulness of a clinical measurement, and evidence of improvement in patient safety and clinical outcome.
Monitoring is the process by which clinical measurements are assessed and used to direct therapy. In general, monitors consist of four components (Table 16.1): (1) a device which connects to the patient – this may either be a direct attachment or via a tube or lead; (2) a measuring device, often a transducer which converts the properties of the patient into an electrical signal; (3) a computer which may amplify the signal, filter it and integrate it with other variables to produce a variety of derived variables; (4) a display which may show the results as a wave, a number or a combination. It is important to appreciate that most monitors do not directly measure the displayed variable, and that the displayed variable may not reflect physiological function. For example, an electrocardiograph (ECG) does not measure cardiac function and therefore a normal ECG trace does not guarantee that the heart is pumping effectively. When interpreting measurements, the following questions should be asked:
TABLE 16.1
Connection to patient
Measuring device
Electronic filter/amplifier
Display
What is being measured? In the case of arterial pressure, there is an obvious answer. However, in some cases, for example ‘depth of anaesthesia’, it may not be clear what the monitor is measuring. In addition, many monitors use data from a variety of sources. For example, heart rate is usually derived from the ECG. However, if the ECG fails to provide the data required, the monitor often switches automatically to a rate from either a pulse oximeter or an arterial pressure waveform. Thus, the displayed value may change rapidly despite the patient remaining stable.
How is it measured? Arterial pressure is often measured by either a transducer attached to an arterial cannula or an automated oscillometer. Although a transducer is often regarded as the more accurate, the readings must be compared with the preoperative values recorded on the ward, usually with an oscillometer. Therefore, where accurate control of arterial pressure is essential, it is advisable to start invasive pressure monitoring before anaesthesia to avoid any confusion with non-invasive measures.
Is the environment appropriate? Many monitors have been designed for use in operating theatres and do not function correctly if exposed to the cold and vibration, for example in an ambulance or helicopter. Another example is the strong magnetic field produced by magnetic resonance imaging (MRI) scanners. The electrical currents induced may damage not only incompatible monitors but even produce burns to a patient’s skin.
Is the patient appropriate? Monitors designed for adult use often fail to produce reliable readings when used on small children. Particularly obese adults may require a large blood pressure cuff, and poor-quality ECG readings may be obtained.
Has the monitor been applied to the correct part of the patient? For example, in aortic coarctation, arterial pressure may be markedly different in each arm. Pulse oximeters also fail to work reliably if placed on a limb distal to a blood pressure cuff.
Is the variable within the range of the monitor? Most monitors are validated on healthy patients in laboratories. Whether such monitors continue to provide accurate results during the extreme physiological changes of, for example, anaphylaxis is uncertain. This does imply that the usefulness of monitors declines with the health of the patient: that is, they are least reliable when needed most. In most cases of acute perioperative patient deterioration, additional monitoring is needed.
Has the monitor been checked, serviced and calibrated at the correct intervals? To reduce costs, departments may re-use single-use equipment and fail to ensure that service checks are carried out. All equipment should be tagged with a service sticker. This should identify the date serviced, when the next service is due and who to contact in case of malfunction. Equipment which has not been serviced or is past its service date should not be used.
Table 16.2 shows the checks which the anaesthetist should follow before using a patient monitor.
TABLE 16.2
What is being measured?
What method is being used?
Has the monitor been serviced and calibrated?
Is the environment appropriate?
Is the patient appropriate?
Is it attached to the appropriate part of the patient?
Is the range appropriate?
Can the display be read?
Are the alarms on and have the limits been set?
PROCESS OF CLINICAL MEASUREMENT
Stages of Clinical Measurement
There are four stages of clinical measurement:
detection of the biological signal, by a sensing device which responds to a characteristic signal in the form of electrical, mechanical, electromagnetic, chemical or thermal energy
transduction, in which the output from the sensor is converted into another form of energy, usually to a continuous electrical signal
amplification and signal processing to extract and magnify the relevant features of the signal and reduce unwanted noise
display and storage – the output from the instrument is presented to the operator. Storage for future use may be achieved using mechanical markers, printed copy or computer memory.
The Microprocessor Revolution
Advantages of digital signal processing include:
continuous real-time detection, processing and recording of measurements
increased range of measurements possible
miniaturization of complicated and powerful instruments
sophisticated artefact rejection and noise reduction algorithms
complex on-line mathematical and statistical signal processing in upgradeable software, e.g. Fourier analysis of the EEG
automated control of the apparatus and the timing and process of measurement, and integration of alarms
storage in memory, permitting trend analysis, future display and further analysis
user-friendly audio-visual display of recordings, integrating many simultaneous clinical measurements, and able to be customized by the user
There are a few important disadvantages:
dependence on electrically powered equipment
degradation of clinical skills and alternative manual measurements through disuse
impoverished understanding of the principles of complex measuring equipment and the requirements for correct use
illusion of the unquestionable accuracy of measurements produced by expensive computer-controlled equipment and presented on an impressive display or typed copy.
Essential Requirements for Clinical Measurement
The Importance of Repeated Measurements
Differences in repeated clinical measurements arise from three causes:
change in the clinical condition of the patient
variability inherent in the biological signal or measuring instrument
confounding errors – the recorded measurement does not reflect the signal.
Measurement of Continuous Signals Over Time
Analogue and Digital Processing
Data Display
Useful instruments communicate measurements in an appropriate and user-friendly manner.
Microprocessor-Controlled Displays
Digital signal processing has revolutionized clinical measurement. Modern monitors comprise a single system integrating various measurements of physiology, and display information as discrete measurements as well as continuous analogue waveforms (Fig. 16.1). This paradox, the conversion of analogue information into digital and then back to analogue, illustrates the real power of digital signal processing to manipulate and present information in a relevant and user-friendly manner. Data patterns (waveforms, trends, graphs) can be recreated or processed in other ways from the original digital signal to assist the anaesthetist, with the original digital signal being stored in a computer record without degradation of the quality of the signal.
BIOLOGICAL ELECTRICAL SIGNALS
Amplification
Noise and Interference
Noise Originating from the Patient: Millivolt ECG potentials on the body surface are hundreds of times larger than microvolt EEG signals on the scalp. EMG signals may be even larger, and muscular activity, especially shivering, causes severe interference. Two features of electronic amplifier design substantially improve the EEG signal-to-noise ratio. ECG potentials are essentially the same across the scalp and are ignored by amplifiers with a high common mode rejection. EMG activity has a higher frequency content than the EEG signal, and may be minimized by a low-pass filter which attenuates the higher-frequency response of the amplifier to a level which attenuates the EMG signals and does not interfere with the characteristics of the EEG.
Noise Originating from the Patient–Electrode Interface: Recording electrodes do not behave as passive conductors. All skin–metal electrode systems employ a metal surface in contact with an electrolyte solution. Polarization describes the interaction between metal and electrolyte which generates a small electrical gradient. Electrodes comprising metal plated with one of its own salts, e.g. silver—silver chloride, avoid this problem because current in each direction does not significantly change the electrolyte composition. Mechanical movement of recording electrodes may also cause significant potential gradients – alteration in the physical dimensions of the electrode changes the cell potential and skin–electrode impedance. Differences in potential between two electrodes connected to a differential amplifier are amplified and asymmetry of electrode impedance seriously impairs the common mode rejection ratio of the recording amplifier.
Noise Originating Outside the Patient: Electrical interference. Mains frequency interference with the recording of biological potentials may be troublesome, particularly in electromagnetically noisy clinical environments. Patients function physically as large unscreened conductors and interact with nearby electrical sources through the processes of capacitive coupling and electromagnetic induction.
THE CARDIOVASCULAR SYSTEM
Electrocardiography
Different lead positions detect electrical activity from different parts of the myocardium. The commonest position of the electrodes used in the operating theatre is the CM5 arrangement, as this is the best position to detect ischaemia of the left ventricle (Fig. 16.2).
Arterial Pressure
Oscillometric Measurement of Arterial Pressure
The oscillometric measurement of arterial pressure estimates arterial pressure by analysis of the pressure oscillations which are produced in an occluding cuff by pulsatile blood flow in the underlying artery during deflation of the cuff (Fig. 16.3). The original automatic oscillometers used two cuffs. The upper cuff was inflated to occlude the arterial flow and then gradually deflated. As the blood flow began to pass under the upper cuff, the small changes in volume were detected by the lower cuff with an electromechanical pressure transducer. Modern machines use a single cuff with two tubes for inflation/measurement. During slow deflation, each pulse wave produces a pressure transient in the cuff which may be distinguished from the slowly decreasing ambient pressure in the cuff. Above systolic pressure, the transients are small, but suddenly increase in magnitude when the cuff pressure reaches the systolic point. As the cuff pressure decreases further, the amplitude reaches a peak and then starts to diminish. The mean arterial pressure correlates closely with the lowest cuff pressure at which the maximum amplitude is maintained. As the cuff pressure reaches diastolic pressure, the transients abruptly diminish in amplitude. To avoid high cuff pressures and long deflation times, monitors inflate the cuff to just above a normal systolic pressure and then slowly decrease the pressure until a pulse is detected. Consequently, estimates of diastolic pressure can be unreliable. If a pulse is not detected, the cuff is then inflated to a higher pressure. This process may be repeated several times before a measurement is made.
FIGURE 16.3 Diagram showing: (A) Relationship between cuff pressure and intra-arterial pressure as cuff pressure decreases during oscillometry; (B) the signal created by the relative pressure changes in A. The sharp spikes of pressure in B are created by the walls of the artery opening and closing. These spikes are detected by a transducer first when the cuff pressure is just below systolic arterial pressure; their amplitude reaches a peak at mean arterial pressure and they cease when the cuff pressure is below diastolic pressure.
Clinical studies comparing automatic oscillometric instruments with direct arterial pressure have demonstrated good correlation for systolic pressure with a tendency to overestimate at low pressures and underestimate at high pressures. Mean and diastolic pressures were less reliable. The 95% confidence interval for all three indices exceeded 15 mmHg. The disadvantages of automated oscillometry are shown in Table 16.3.
TABLE 16.3
Disadvantages of Automated Oscillometry
Delayed measurement with arrhythmias or patient movement
Inaccuracy with systolic pressure < 60 mmHg
Inaccurate if the wrong size cuff used
May be inaccurate in obese patients
Discomfort in awake patients
Skin and nerve damage in prolonged use
Delay in injected drugs reaching the circulation
Backflow of blood into i.v. cannulae
Pulse oximeter malfunction as cuff is inflated
Other Techniques: Measuring devices depend on the detection of movement of the arterial wall using changes in pressure or sound below audible frequencies and detection of blood flow using the Doppler shift of an ultrasound signal, or plethysmography. Several other techniques have been used to measure arterial pressure, but have failed to find widespread usage. These include the Penaz technique, which measures the effect of external pressure on the blood flow through a finger, and other devices relying on pressure measurements over an artery, Doppler probes or detection of Korotkoff sounds with a microphone.
Direct Measurement
The advantage of direct measurements is a real-time measure of arterial pressure, which is essential when administering drugs such as vasopressors to critically ill patients (Table 16.4). Such measurement systems also provide a means for obtaining samples for arterial blood gas analysis and other blood tests. The use of arterial cannulae has therefore become standard practice for severely ill patients, both in the operating theatre and in the intensive care unit.
TABLE 16.4
Advantages of Direct Arterial Pressure Measurement
Accuracy of pressure measurement
Beat-by-beat observation of changes when blood pressure is variable or when vasoactive drugs are used
Accuracy at low pressures
Ability to obtain frequent blood samples
However, errors are common as a result of malpositioning of the transducers and failure to zero the transducer before use. For example, if the operating table is moved upwards while the transducer remains static, the difference in height artificially increases the pressure reading. Further, while modern disposable sets are usually reliable and accurate, they may occasionally malfunction. Unusual readings should therefore be checked against a reading from a non-invasive monitor. Complications relating to arterial cannulae are shown in Table 16.5.
TABLE 16.5
Complications Relating to Arterial Cannulae
Requires skill to insert
Bleeding
Pain on insertion
Arterial damage and thrombosis
Embolization of thrombus or air
Ischaemia to tissues distal to puncture site
Sepsis
Inadvertent injection of drugs
Late development of fistula or aneurysm
Resonant Frequency and Damping: Fourier showed that all complex waveforms may be described as a mixture of simple sine waves of varying amplitude, frequency and phase. These consist of a fundamental wave, in this case at the pulse frequency, and a series of harmonics. The lower harmonics tend to have the greatest amplitude and a reasonable approximation to the arterial pressure waveform may be obtained by accurate reproduction of the fundamental and first 10 harmonics. In other words, to reproduce an arterial waveform at 120 beats min–1 accurately would require transduction with a linear frequency response up to a frequency of at least (120 × 10)/60 = 20 Hz. Accurate reproduction of a waveform requires that both the amplitude and phase difference of each harmonic are faithfully reproduced. This requires a transduction system with a natural frequency higher than the significant frequency components of the system, and the correct amount of damping.
The fluid and diaphragm of the transducer constitute a mechanical system which oscillates in simple harmonic motion at the natural resonant frequency. This determines the frequency response of the measurement system (Fig. 16.4). The resonant frequency of a catheter-transducer measuring system is highest, and the frictional resistance to fluid flow which dampens the frequency response is lowest, when the velocity of movement of fluid in the catheter is minimized. This is achieved with a stiff, low-volume displacement diaphragm and a short, wide, rigid catheter.
Determination of the Resonant Frequency and Damping: The resonant frequency and the effects of damping may be estimated by applying a step change in pressure to the catheter-transducer system and recording the response (Fig. 16.5). The underdamped system responds rapidly but overshoots and oscillates close to the natural resonant frequency of the system; frequency components of the pressure wave close to the resonant frequency are exaggerated. By contrast, the overdamped system responds slowly and the recorded signal decreases slowly to reach the baseline, with no overshoot. High-frequency oscillations are damped, underestimating the true pressure changes. These extremes are undesirable.
Optimal Damping: Optimal damping maximizes the frequency response of the system, minimizes resonance and represents the best compromise between speed of response and accuracy of transduction. A small overshoot represents approximately 7% of the step change in pressure, with the pressure then following the arterial waveform (Fig. 16.5).
Central Venous Pressure
There are four common routes for central venous catheterization.
Long catheters inserted via the antecubital fossa are relatively easy and safe to insert but are of small diameter. Catheters inserted via the basilic or cephalic vein are sometimes difficult to advance past the shoulder. It is also difficult to determine if the tip of the catheter is within a central vein without X-ray imaging. Thrombosis of the veins is common if the catheter is left in situ for more than 24 h.
Femoral venous catheters are inserted just below the inguinal ligament. They are also relatively easy to insert and may be of large gauge to allow rapid transfusion of fluids. This route is often chosen in children. However, the site of insertion is often within a skin fold, making skin sepsis more likely.
Internal jugular catheters are used most commonly because the vein is superficial, of larger diameter, and easily managed. This is the route which is often most appropriate for use in an emergency. However, the insertion point is adjacent to several vital structures, including the carotid artery, lung, brachial plexus and cervical spine, with the result that direct needle trauma to these structures can occur. Current guidelines recommend the use of an ultrasound probe for insertion of a catheter via the internal jugular route to improve the accuracy of insertion and to minimize complications.
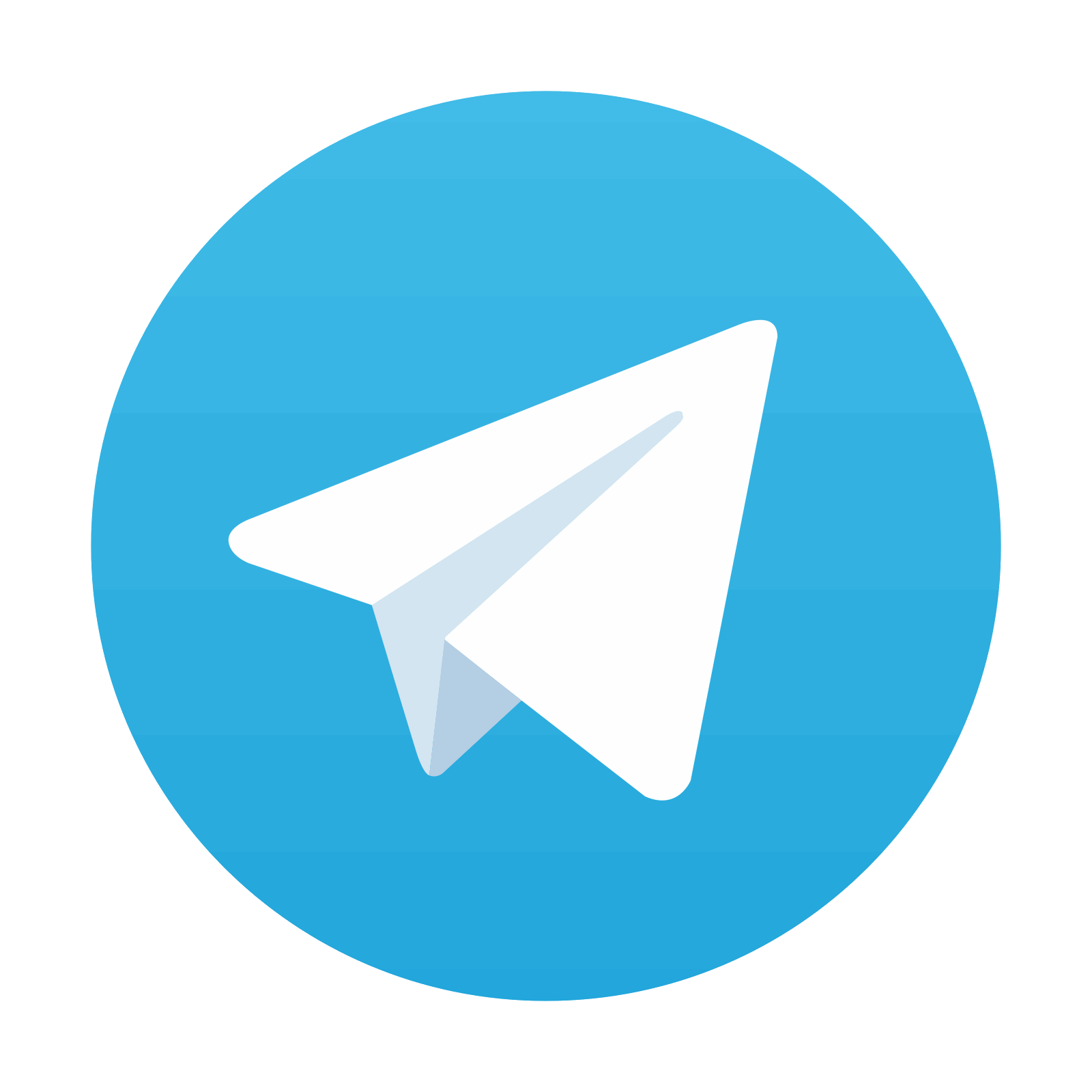
Stay updated, free articles. Join our Telegram channel

Full access? Get Clinical Tree
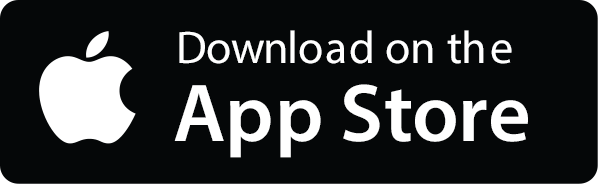
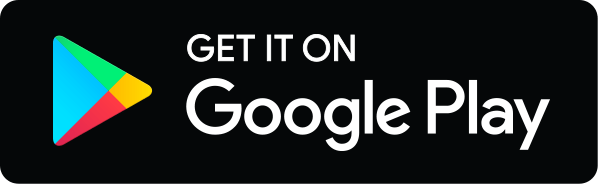