Introduction
Throughout history, literally since ancient times when the physician to Roman emperor Claudius, Scribonius Largo, in 46 AD wrote of applying electric rays (Torpedo torpedo and Torpedo nobiliana) to the cranial surface as a treatment of headaches, electrical stimulation has been used in the treatment of various neurological disorders.1 Electroshock, introduced by Ugo Cerletti (1877–1963) in 1938 for the treatment of severe psychosis, was the first modern example of therapeutic application of brain stimulation.2
Functional neurosurgery has been a rapidly growing form of neurosurgical therapy. Traditionally, these have most commonly been performed for the treatment of medically refractory Parkinson’s disease (PD), but expanded to include essential tremor, dystonia, and some neuropsychiatric diseases.3 Surgical procedures such as pallidotomy and thalamotomy were performed to ameliorate the dystonic symptoms in patients with PD. However, over the past 30 years deep brain stimulation (DBS) has supplanted these irreversible surgical procedures. DBS evolved from observing the effects of electrical stimulation of deep brain loci when identifying the correct position of coagulant electrodes for these ablative procedures. Deep brain stimulators consist of the microelectrodes implanted in specific brain areas connected, with subcutaneously tunneled wires, to impulse generators located under the skin in the chest or abdomen. The impulse generators send electrical impulses to the microelectrodes, which alters the spontaneous neural discharges in these areas. The Food and Drug Administration (FDA) first approved thalamic DBS in 1997 for tremor, and globus pallidus internus (GPi) and subthalamic nucleus (STN) in 2003 for PD.4 DBS has emerged as the treatment of choice in adults for the treatment of PD refractory to medical therapy. Fasano and Lozano reported that currently more than 100,000 patients have undergone DBS surgery. As opposed to pallidotomy, DBS is less destructive of brain tissue, is associated with fewer cognitive and motor side effects, and is reversible and adjustable.6
More recently, DBS has also been found to be effective for the treatment of medically refractory dystonia, which frequently becomes symptomatic in childhood or early adolescence.7 Dystonia is a syndrome of sustained muscle contractions producing twisting and repetitive movements or abnormal postures often resulting in simultaneous contraction of both agonist and antagonist muscles. Voluntary movement is often spastic and painful. Dystonias are classified as either primary (idiopathic) or secondary. Primary dystonia occurs without other neurological signs and without magnetic resonance imaging (MRI) evidence of brain abnormalities. Secondary dystonia is associated with a definable lesion in the CNS caused by stroke, cerebral palsy, encephalopathies, or neurodegenerative diseases. These are usually associated with abnormal brain images as seen on MRI or with a known history of major central nervous system (CNS) injury.8 Primary dystonias can be generalized (primary generalized dystonia), segmental (affecting adjoining parts of the body), or focal (affecting one muscle or muscle group).9 Primary dystonias are often hereditary. The best-known gene locus is the DYT1 (also referred to as TOR1A), where a three-base pair deletion is responsible for approximately 30% of juvenile-onset primary generalized dystonias.10 Medical management includes levodopa, trihexyphenidyl, baclofen, botulinum toxin injections, and others. Treatment of secondary dystonia with trihexyphenidyl has had mixed results. Irritability, sedation, and constipation have all been dose-limiting factors of trihexyphenidyl therapy. Botulinum toxin is useful for focal dystonias but has time-limited effectiveness, requiring repeated injections.11
Intrathecal baclofen (ITB) is a treatment option for both dystonia and spasticity in the pediatric population.12 Baclofen is a gamma-aminobutyric acid (GABA) agonist that is thought to affect neurons in the spinal nerve roots and cortex. Oral baclofen does not adequately cross the blood-brain barrier and thus is often administered directly into cerebrospinal fluid (CSF), either as a bolus or as a continuous infusion, via a pump. Secondary dystonias tend to respond well to ITB therapy, while primary dystonias do not.9 The incomplete efficacy of ITB and other drug regimens when treating dystonia and the successful use of DBS in PD led investigators to try DBS for the treatment of dystonia in the pediatric population.
In 2003, the Activa DBS (Medtronic, Minneapolis, MN) device was granted limited FDA approval in the United States for primary generalized and segmental dystonia, in patients ages 7 years or greater, under the humanitarian device exemption (HDE).10 Both the GPi and STN targets were included in the HDE labeling.
The basal ganglia, specifically the globus pallidus, play an important role in dystonia. Electrophysiological recordings in the GPi of humans with primary dystonia show abnormal oscillatory discharge patterns.10 This finding coupled with the successful reductions of dystonic muscle spasms with pallidotomy in PD patients has led to the targeting of the GPi for DBS microelectrode implantation. The GPi is by far the most targeted site for the placement of DBS with few studies targeting the STN.3–14
Most major surgical centers require patients to meet several criteria to be considered for DBS: (1) unequivocal diagnosis of primary or secondary dystonia; (2) failure to manage dystonia with anticholinergic, antiepileptic, benzodiazepines, baclofen, or, in patients with focal or segmental dystonia, treatment failure after injection of botulinum toxin; (3) significant disability, despite optimal medical management. Standardized rating scales are used to accurately assess clinical outcomes. The most commonly used rating scale for generalized dystonia is the Burke-Fahn-Marsden Dystonia Rating Scale (BFMDRS)15, 16 and for cervical dystonia is the Toronto Western Spasmodic Torticollis Rating Scale (TWSTRS).17 Reviews and meta-analyses of several studies have examined the efficacy of DBS in the treatment of primary dystonia with and without the DYT1 mutation.18 Almost all studies have reported improvement with DBS, but the degree of improvement has varied widely across studies, ranging from 21% to 95% improvement in the BFMDRS movement score, with most studies showing 60% to 70% improvement.3, 5, 10, 11, 14 For example, Vidailhet et al. studied 22 patients with primary generalized dystonia. The patients were evaluated preoperatively, and at 3, 6, and 12 months postoperatively. A mean improvement of 54% in the BFMDRS movement score was seen at 12 months with chronic stimulation. At 3 months, patients underwent videotaped double-blind evaluations in the presence and absence of neurostimulation on alternate days. When stimulated, patients showed a statistically significant mean improvement of 29% in the BFMDRS movement score, compared with the unstimulated condition.19
Several authors have reported long-term improvement of patients with primary dystonia who were treated with DBS of the GPi. Vidailhet et al. reported that in a 3-year follow-up study, their previously reported 22 patients continued to show mean improvement in BFMDRS movement scores of 58%.20 Others have also reported long-term improvement for as long 10 years after surgery.9, 21–23 Goto et al. and Hebb et al. reported on cases where after several years of treatment the DBS was stopped without any deterioration in clinical benefit in cervical or cranial dystonia.24, 25
The benefits of GPi DBS in primary dystonia are well documented, while the benefits in patients with secondary dystonia are less certain. Meta-analysis performed by Holloway et al. identified three factors associated with outcomes of DBS for dystonia: etiology of dystonia, duration of dystonia, and nucleus stimulated.14 Several studies found significantly better outcomes in patients with primary dystonia than in those with secondary dystonia.4, 10, 14 Patients with long-standing dystonia with fixed skeletal deformities can have limited functional improvement with DBS, even when dystonia symptoms are ameliorated.26 Placement of DBS in the GPi showed overall better patient outcomes than those with DBS placed in the VLp of the thalamus.27 In a more recent review, Fasano and Lozano expanded the list of predictors of surgical outcome to include etiology, brain MRI, body site, genetic status, phenomenology, duration of dystonia, implanted site, type of surgery, target, and energy delivered with stimulation.5 Interestingly, Marks et al.11 and Air et al.7 noted that while patients with secondary dystonia (particularly cerebral palsy) showed less impressive responses to DBS, the caregivers reported meaningful benefits despite small absolute changes in rating scale scores. Traditional dystonia scales may not be able to distinguish small but meaningful improvements in levels of function that are important in this patient population.
The body of evidence has definitively demonstrated the value of DBS in the treatment of movement disorders as originally seen in adults with PD and more recently in patients of all ages with dystonia. As the duration of dystonia has been significantly correlated to outcomes, placing DBS in pediatric patients is becoming more commonplace. Surgical placement of DBS in the pediatric population presents substantial anesthetic challenges. The remainder of this chapter focuses on the techniques employed to overcome these challenges.
Anesthetic Considerations
There is currently no consistent agreement in the literature as to which anesthetic technique should be used for DBS surgery. The technique used varies according to institutional practices and has included monitored anesthesia care with nerve blocks or local anesthesia, conscious sedation, and general anesthesia. Currently, none of the anesthetic techniques for DBS insertion has produced significantly superior outcomes over the others.7, 10, 14 However, several studies report that general anesthesia diminishes the microelectrode recordings (MERs) used to fine-tune the localization of the optimal site for placement of the DBS electrodes, as targeted using coordinates obtained with MRI.28 Hutchinson found the firing rates in GPi were substantially decreased and long pauses were noted in patients with dystonia receiving general anesthesia, with propofol, compared to those mapped under local anesthesia.29 The effect of propofol on GPi firing rates may be explained by the high density of GABA receptors in this region. Propofol has been reported to have a high affinity for GABA receptors in the GPi.30–32 Several reports also recommend avoiding the use of benzodiazepines, due to their high affinity for GABA receptors and relatively long duration of action. They have been shown to significantly diminish MERs in the GPi.32, 33
Conversely, Pinsker et al.34 reported excellent results in a fairly large series where they implanted 86 DBS electrodes in 42 patients (reoperation in two patients) under general anesthesia with intravenous (IV) propofol and remifentanil. They did not report any diminution of MER due to general anesthesia. Further, they reported an average motor improvement from baseline of 64.72% (range = 20.39–98.52%) as assessed by either the BFMDRS or the TWSTRS at the final patient follow-up greater than 1 year after implantation. These results compare well with the results noted by Holloway et al. in their meta-analysis.14 Sanghera et al.35 studied the effects of general anesthesia with desflurane in 11 pediatric patients with dystonia. They found no differences between awake and anesthetized patients with respect to GPi nuclei firing rates.
The previous studies notwithstanding, a substantially greater number of reports on DBS surgery employ monitored anesthetic care with local anesthesia. Of the drugs studied, dexmedetomidine has emerged as the drug of choice for awake craniotomies during DBS placement in both adult and pediatric populations.3, 6, 11, 14, 28, 29, 31–33, 36–38 Dexmedetomidine is a potent, highly selective α-2-adrenoreceptor agonist, acting at the subcortical regions of the brain, specificallywithin the locus ceruleus. Efferent neurons within the locus ceruleus release norepinephrine (NE), which stimulates wakefulness and arousal. The α-2-adrenoreceptors are present in the presynaptic membranes and inhibit the release of NE. By inhibiting the release of NE from the locus ceruleus, dexmedetomidine produces sedation that closely simulates natural sleep. While patients are well sedated, they can be easily aroused intraoperatively during DBS testing. Dexmedetomidine does not interact with GABA receptors and therefore doesn’t alter MERs within the GPi. Koroglu et al.38 compared the sedative, hemodynamic, and respiratory effects of dexmedetomidine to those of propofol in children undergoing MRI. Onset of sedation was significantly faster with propofol, but dexmedetomidine produced less hemodynamic changes and had significantly less respiratory depression. Propofol frequently produced brief periods of apnea and oxygen desaturation. This was very rare with dexmedetomidine. Respiratory effects seen with dexmedetomidine were usually due to the bolus being given too rapidly. Dexmedetomidine is therefore an excellent medication for use in “awake” anesthesia for DBS placement. With this in mind, the remainder of this chapter is devoted to the technique of “awake anesthesia” as practiced at my hospital (Cook Children’s Medical Center [CCMC] in Fort Worth, TX).
Anesthesia for “Awake” DBS Placement: The CCMC Way
The overall surgical experience for the patient and their family can be divided into three basic time frames. These are (1) the preoperative evaluation and patient preparation; (2) day of surgery procedures including IV access, head frame placement and MRI scan, and DBS placement and testing; and (3) pulse generator implantation (typically occurs a few weeks after the electrode placement).
Patient Selection, Preoperative Evaluation, and Patient Preparation
Patient selection is critical to successful implantation. Risks and benefits should be weighed, and all alternatives, especially less invasive ones, must be considered. The use of DBS in primary genetic dystonias, especially DYT1, is now well accepted as a treatment option. There is now good experience with children in several institutions. Patients selected as candidates for DBS surgery undergo extensive neuropsychological assessment. The patients and their parents are provided with all the information necessary to making an informed decision about DBS placement.
The value of preoperative patient preparation and perioperative support is extremely important. Child life specialists or specially trained psychologists can teach the patients relaxation and stress management strategies. These include imagery, biofeedback, and distraction. Studies have found that child life preparation significantly reduced children’s anxiety throughout the perioperative period. Further, anxiety scores were reportedly reduced for up to 1 month postoperatively.39–41 At CCMC, a child life specialist meets with the patients and their families during the preoperative visit. The child life specialist then accompanies the patient throughout the surgery providing emotional support. This improves the likelihood that the patient can be successfully maintained under minimal anesthesia during the critical lead placement using MER, and allows for postplacement motor testing.
In addition to the preoperative preparation, the patient receives a thorough preanesthetic evaluation. Past medical and surgical histories are obtained and pertinent labs are drawn (typically complete blood count (CBC), basic metabolic panel, and coagulation studies). The anesthesiologist works closely with the child life specialist to help reduce the patient’s (and the patient’s family’s) anxiety over the upcoming surgery. In institutions without child life services, the perioperative nursing staff and the anesthesia team can perform many of the preparation techniques.39
Day of Surgery
Induction, Head Frame Placement, and MRI Scan
On the day of surgery, the patient is met by the child life specialist and brought to a preoperative preparation room, where all the standard surgical paperwork and identification procedures are completed and surgical consents are signed. Older, cooperative patients have their IV started at this time. For younger or nervous patients, the IV is not started until they are in the operating room (OR). Typically, no preoperative medication is given and, as noted earlier, midazolam in particular is avoided owing to its significant inhibition of MERs in the GPi. However, if a patient is significantly upset intranasal dexmedetomidine (1 mcg/kg) can be given as a preoperative medication. Yuen et al.42 compared the effects of intranasal dexmedetomidine and oral midazolam for premedication. They found no significant differences in parental separation acceptance, behavior scores at induction, and wake-up behavior scores. They noted that the dexmedetomidine treated patients were significantly more sedated at induction than the midazolam patients.
The patient is brought to the OR by the circulating nurse and the child life specialist. Here the IV is started (if needed) and head frame placed. All standard monitors are placed and the patient is initially sedated with inhalational N2O for the IV placement. Once the IV is secured, the N2O is discontinued and a bolus dose of dexmedetomidine (0.5–1 mcg/kg) is infused over 10 minutes, followed by a continuous infusion of 0.2–0.7mcg/kg/hr. We avoid the use of anticholinergic drugs due to concerns their use combined with dexmedetomidine may cause significant hypertension and tachycardia. In addition, the first dose of vancomycin (10 mg/kg) is infused over 30 minutes. In order to reduce the discomfort associated with the placement of the head frame, a modified scalp block is performed (see Chapter 6). A complete scalp block involves regional anesthesia to the nerves that innervate the scalp, including the greater and lesser occipital nerves, the supraorbital and supratrochlear nerves, the zygomaticotemporal nerves, the auriculotemporal nerves, and the greater auricular nerves. Figure 9.1 is an anatomic representation of the nerve distribution in the scalp. Pinosky et al.43 found that performing a scalp block significantly reduced the hemodynamic response to head pinning in anesthetized patients. The modified scalp block involves the supraorbital and supratrochlear nerves anteriorly and the greater and lesser occipital nerves posteriorly. The supraorbital and supratrochlear nerves are blocked with 2 mL of 0.2% ropivicaine, on each side, as they emerge from the supraorbital notch. A 23-gauge needle is inserted approximately 1 cm lateral to the foramen and advanced in a medial direction to avoid entering the foramen. The greater and lesser occipital nerves are blocked with a field block using 5 mL of 0.2% ropivicaine. A 22-gauge spinal needle is inserted approximately halfway between the occipital protuberance and the mastoid process and then advanced laterally along the nuchal line while injecting the ropivicaine. In our experience, this modified scalp block is very effective in reducing the discomfort associated with the head frame. In addition, our patients tend to report less postoperative headache associated with the head frame. As an added measure the scalp areas where the frame pins will be located are infiltrated locally with 2–3 mL of 0.5% bupivacaine. In fact, we use this as an indicator of the effectiveness of the scalp block. With an effective scalp block the patients do not respond to the needle stick or local injection. Because of its rapid onset and short duration of action, small boluses of propofol (0.5–1mg/kg) can be given to more heavily sedate the patient during the scalp block and head frame placement. At CCMC we have found that preforming the scalp block and placing the head frame are more easily accomplished if the patient is propped up in a seated position. In fact, we routinely perform this part of the surgery in a wheelchair (Figure 9.2).

Full access? Get Clinical Tree
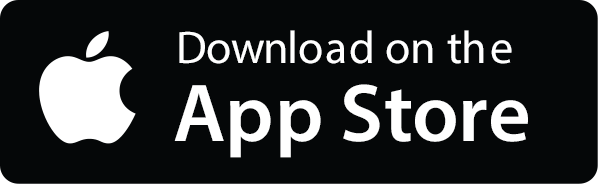
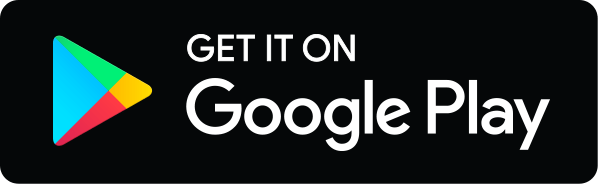