Introduction
Any chemical agent with general anesthetic properties and the ability to be delivered via inhalation is labeled as an “inhalational anesthetic agent.”
Characteristics of an ideal inhalation anesthetic used for induction and maintenance of general anesthesia in the operating room are as follows:
Ability to be obtained in pure form with low cost of production.
Safe when used for long durations.
No acute cardiovascular or respiratory side effects.
Low blood: Gas solubility ensuring rapid induction and elimination.
The use of inhalational anesthetic agents has been extended to the intensive care units (ICUs) where they have been administered for sedation, for the management of refractory bronchospasm, and for the control of status epilepticus, which are unresponsive to conventional anticonvulsant medications.
History
The concept of inhalational anesthesia dates back to the 11th century when the Arab physicians used a sponge soaked in a narcotic drug on a patient’s face.
Table 8.1 summarizes the milestones in the history of inhalational anesthesia.
Classification
The inhalational anesthetics can be either volatile anesthetic agents or nonvolatile anesthetic gases.
Volatile Anesthetic Agents
These agents are liquid at room temperature and require a specialized device called a vaporizer for inhalational administration.
A vaporizer accurately varies the amount of anesthetic vapor added to the total gas mixture and in turn the concentration of vapor in the alveoli.
Volatility is the tendency of a liquid to vaporize, and the pressure developed by the evaporation of the liquid volatile agent is called the vapor pressure, which is dependent on the surrounding temperature and the physical properties of the liquid.
The most commonly used volatile anesthetic agents are summarized below:
Mechanism of Action
It is still largely unknown, and the action of the agents on multiple targets contributes to the anesthetic effect.
The site of action for the immobilizing effect is in the spinal cord. In contrast, the supraspinal sites, namely, the amygdala, hippocampus, and cortex, are the targets for sedation, hypnosis, and amnesia. The hypothesis behind the action of inhaled anesthetics is still an area of ongoing research. However, the common hypotheses are as follows:
Lipid solubility theory: It states that the movement of gas into the lipid membrane disrupts the ion channels and the propagation of the action potential. Lipid solubility forms the basis of the Meyer–Overton hypothesis.
It is suggested that when a sufficient number of inhalational anesthetic molecules bind to target sites in the brain cells and dissolve in the lipid cell membrane, they produce an anesthetized state.
It postulated that the number of molecules dissolved in the lipid cell membrane and not the type of inhalational agent is important to cause anesthesia. It thus supports the additive nature of anesthetic agents.
This concept was supported by the fact that there existed a direct correlation between lipid solubility of inhaled anesthetics and the potency expressed as minimum alveolar concentration (MAC). Potency increased as oil:gas solubility increased.
Lipid bilayer expansion theory: Binding of anesthetic agents can modify the lipid bilayer by changing its curvature/elasticity, by causing changes in phase separation, and by creating changes in bilayer thickness. Lipid bilayer expansion is the basis of the critical volume hypothesis by Mullins.
Critical Volume Hypothesis by Mullins: It stated that the absorption of bulky and hydrophobic anesthetic molecules into the hydrophobic region within the cell membrane causes its distortion due to volume displacement. Expansion beyond a “critical volume” distorts channels necessary for sodium-ion flux and the development of action potentials essential for synaptic transmission.
These theories were introduced to overcome the weakness of the lipid-based theories (stereoisomers of an anesthetic agent have variable potency but same oil:gas partition coefficient).
It states that the anesthetics bind to the hydrophobic portion of the ion channels/proteins and can either induce/prevent or alter the kinetics of the conformational change or compete with various ligands. Some examples are:
Action at the molecular level: Two-pore domain potassium channels contribute to potassium membrane conductance and are widely distributed in the central nervous system (CNS) both pre- and postsynaptically. Halothane, isoflurane, sevoflurane, and desflurane enhance the activity of these channels, leading to hyperpolarization of the plasma membrane and potentially explaining their anesthetic effects.
Neuromodulator theory states that the anesthetics bind to cell surface receptors and increase the flux of chloride ions.
Pharmacokinetics of Inhalational Agents
Pharmacokinetics involves the uptake and elimination of inhalational agents. Several partial pressure gradients exist between the anesthetic machine and the final target organ, that is, the brain. Thus, we can alter the brain concentration indirectly by in-depth knowledge of pharmacokinetics. The factors which account for the difference in gradients are further classified as inflow and outflow factors:
Inflow factors: The inflow factors determine the concentration of inhaled anesthetics in the alveoli. These are as follows:
Vaporizer setting/inspired concentration of agent (Fi): The greater the inspired concentration, the faster the induction of anesthesia. The inspired concentration can be achieved by either increasing the higher dial setting of the vaporizer or by increasing the amount of fresh gas flow.
Breathing system: Lower volume of breathing system with lower circuit absorption leads to higher inspired concentration.
Effective alveolar ventilation: Increased and effective alveolar ventilation results in a faster increase in alveolar partial pressure.
The factors which govern it are as follows:
Functional residual capacity (FRC): A larger FRC leads to a decrease in alveolar partial pressure because of dilution of inspired gases and, consequently, slower onset of anesthesia.
The time constant: It is an important concept to be understood. The time constant for the circuit is the total volume of circuit divided by the fresh gas flow rate, while that for lungs, it is the volume of FRC divided by minute ventilation.
It takes three time constants for 95% of a concentration change to be achieved.
Second gas effect: The coadministration of an anesthetic agent with nitrous oxide results in the increased partial pressure of inhaled anesthetic agents. This occurs due to the rapid uptake of nitrous oxide by the lungs. This phenomenon is termed as the second gas effect.
Outflow factors: These factors are the determinant of uptake of inhaled anesthetics from the lungs. The uptake of the inhaled anesthetic agent can be calculated using the formula:
Uptake = λB/G × Q × (PA–PV) divided by barometric pressure, where
λB/G = blood-gas partition coefficient;
(PA–PV) = difference between alveolar partial pressure and mixed venous pressure.
The factors governing the uptake are:
Solubility: It is defined as the ratio of its concentration in the blood to alveolar gas when there is no difference in their partial pressures. The blood solubility of an inhalational agent is expressed as a blood/gas partition coefficient (λB/G).
The agents with a higher blood–gas partition coefficient (chloroform, ether) have a greater uptake in the pulmonary circulation, a slower increase in alveolar partial (FA/Fi ratio), and therefore prolonged induction.
Cardiac output (Q): Increase in cardiac output means a greater uptake, that is, amount of agent removed from the alveolus increases leading to a fall in alveolar concentration.
Alveolar venous partial pressure gradient (PA–PV): The value of this gradient depends on the tissue uptake. The tissue uptake, in turn, depends on:
Out of all, blood flow is the most important factor, and based on it, the tissues have been classified into:
High absorption and slow release of anesthetic agents from fat tissues result in more delayed induction and emergence in obese.
Lower albumin and cholesterol levels also account for rapid onset of anesthesia.
Minimum Alveolar Concentration
MAC is the minimum end-tidal concentration of inhaled anesthetic at 1 atm pressure in 100% oxygen at equilibrium, which produces immobility in 50% of subjects in response to a standard surgical midline incision. It reflects the actions of an inhalation agent on spinal cord-mediated reflexes. It is equivalent to the ED50 expressed for intravenous (IV) agents. The MAC value of commonly used anesthetic agents is listed in Table 8.2.
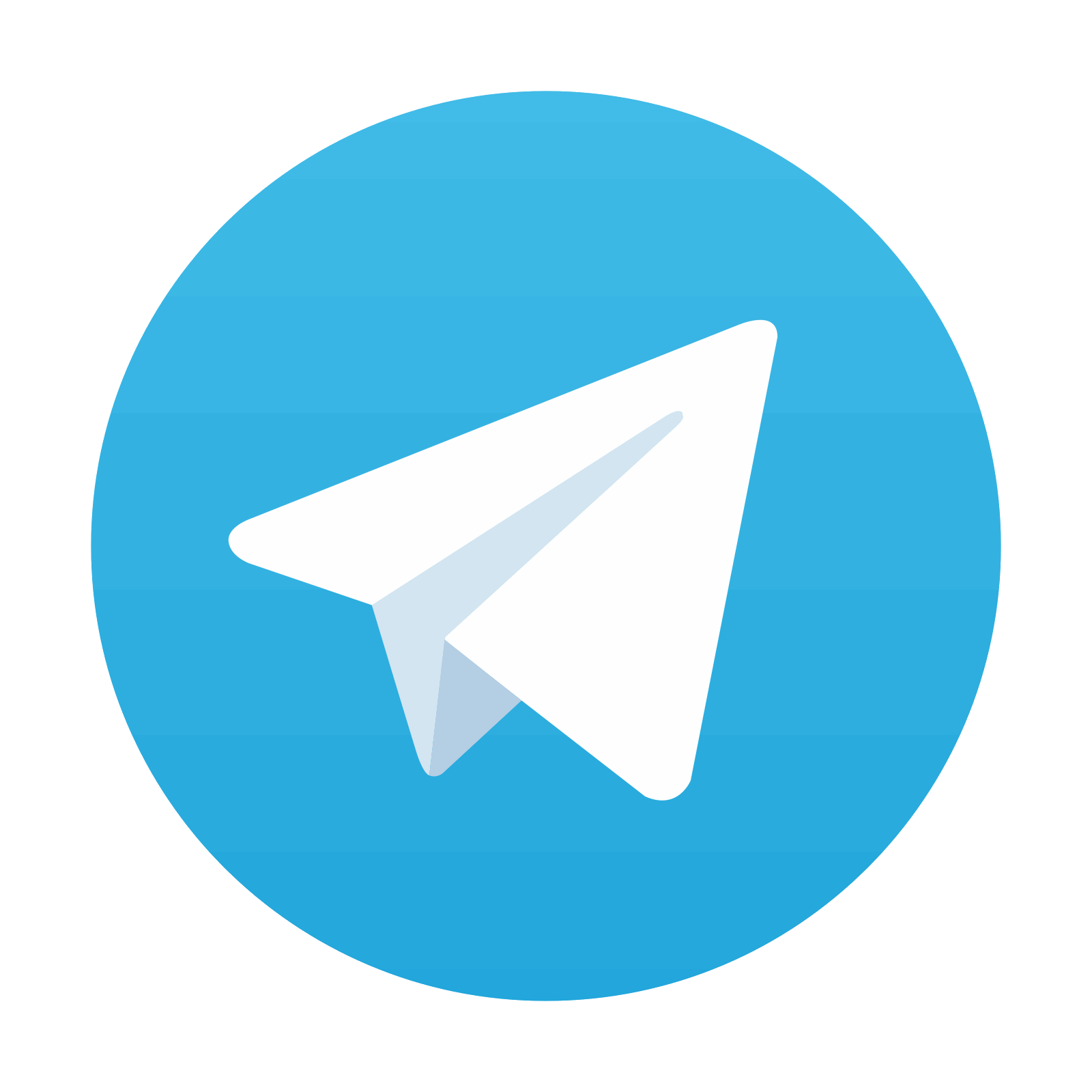
Stay updated, free articles. Join our Telegram channel

Full access? Get Clinical Tree
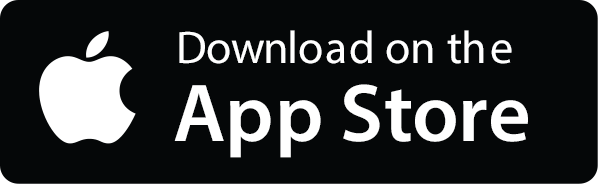
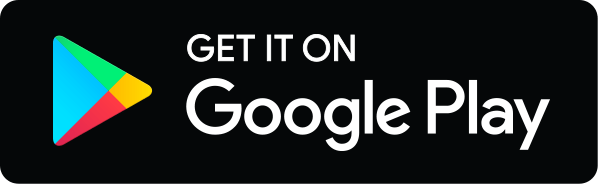
