Key Points
A post-operative pulmonary complication is a pulmonary abnormality that produces significant disease or dysfunction that adversely affects the patient’s clinical course.
Pulmonary complications are more likely to predict long-term mortality after surgery, particularly among older patients.
Pulmonary conditions can be preoperatively investigated and optimised in order to decrease the risk of post-operative pulmonary complications.
Clinical investigations such as spirometry, flow volume curves, pulmonary function testing and cardio-pulmonary exercise testing can be useful in assessing the severity of pulmonary conditions and identifying patients at risk of perioperative morbidity.
By eliciting a careful history and physical examination, performing the right investigation at the right time and seeking specialist opinion early, we can reduce the likelihood of post-operative pulmonary complications.
Introduction
As a reflection of improvements in healthcare, an increasing number of patients living with multiple co-morbidities, such as chronic obstructive pulmonary disease (COPD) and obesity, are undergoing surgery. COPD is currently reported to affect 5 per cent of adults in the UK, with obesity affecting almost 25 per cent (Raluy-Collado et al., 2015). Consequently, post-operative complications due to pulmonary pathophysiology are becoming more commonplace.
The reported frequency of post-operative pulmonary complications in the literature varies from 2 per cent to 70 per cent. This wide range is due, in part, to patient selection and procedure-related risk factors. Differing definitions also, however, account for much of the variability and make comparison of reported incidences across different studies difficult.
One definition for a post-operative pulmonary complication is ‘a pulmonary abnormality that produces identifiable disease or dysfunction that is clinically significant and adversely affects the clinical course’ (O’Donohue, 1992). This would therefore include (Hall et al., 1991):
Bronchospasm
Atelectasis
Infection, including bronchitis and pneumonia
Prolonged mechanical ventilation and respiratory failure
Exacerbation of underlying chronic lung disease
Pulmonary thromboembolism.
Pulmonary complications may also be more likely than cardiac complications to predict long-term mortality after surgery, particularly among older patients (Manku, Bachetti and Leung, 2003). In this chapter we will be focussing not only on pulmonary conditions that can be preoperatively investigated and optimised in order to decrease the risk of post-operative pulmonary complications post cardiothoracic surgery, but also on clinical investigations to identify those patients at risk.
Spirometry
Spirometry is the most frequently used measure of lung function, measuring volume against time (Figure 6.1). It can be performed relatively quickly. Patients are usually sitting up in a chair. They are asked to take a maximal inspiration in, and are then asked to expel air for as long and as quickly as possible. Measurements that are made include:
forced expiratory volume in one second (FEV1)
forced vital capacity (FVC)
the ratio of the two volumes (FEV1/FVC).
Spirometry and the calculation of the FEV1/FVC allow the identification of obstructive or restrictive ventilator defects. A FEV1/FVC <70 per cent where the FEV1 is reduced more so than the FVC signifies an obstructive defect (Figure 6.2).
Figure 6.2 Obstructive spirometry. Obstructive spirometry demonstrated with the interrupted line.
Obstructive defects on spirometry are caused by lung diseases such as asthma and COPD.
An FEV1/FVC >80 per cent, where the FVC is reduced more so than the FEV1, is seen in restrictive defects such as interstitial lung diseases (ILD) and diseases leading to chest wall abnormalities (Figure 6.3).
Figure 6.3 Restrictive spirometry. Restrictive spirometry demonstrated with the interrupted line.
Flow Volume Curves
Spirometry focusses mainly on expiration. Sometimes, it is useful to look at both the inspiratory and expiratory phases of respiration. This is where a flow volume curve comes in. Flow volume curves are produced when a patient performs a maximal inspiratory manoeuvre, which is followed by a maximal expiratory manoeuvre. A graph is produced with a positive expiratory limb and a negative inspiratory limb (Figure 6.4). The maximal flow rate during expiration can also be measured; this is the peak expiratory flow rate (PEFR).
Patients with obstructive lung diseases have reduced expiratory flow in the peripheral airways. This leads to a concave appearance of the descending portion of the expiratory flow limb, rather than a straight line. (Fig 6.5) In patients with emphysema, the loss of elastic recoil and structural support results in collapse of the distal airways towards the end of expiration. This results in ‘scalloping’ of the expiratory limb. Even if the flow volume curve morphology is normal, a reduction in PEFR may be an indication of asthma with early airflow obstruction.
Figure 6.5 An obstructive flow volume curve. An obstructive pattern displayed by the interrupted curve.
In restrictive lung disease, the expiratory limb has a linear or a convex appearance because the flow rates are preserved, but the problem relates to a parenchymal disorder, e.g. lung fibrosis. (Fig 6.6)
Figure 6.6 A restrictive flow volume curve. A restrictive pattern displayed by the interrupted.
Flow volume curves come into their own in the detection of large airway abnormalities. Intrathoracic large airway obstruction, e.g. due to a tracheal tumour, results in the flattening of the expiratory limb. (Fig 6.7) The inspiratory limb is preserved. This is because in expiration there is a rise in intrathoracic pressure. The presence of an obstructive lesion causes a drop in the resultant airflow, which is seen on the curve.
Figure 6.7 A flow volume curve in intrathoracic obstruction. A flow volume curve in intrathoracic obstruction is displayed by the interrupted curve.
In fixed extrathoracic large airway obstruction (e.g. vocal cord paralysis) there is symmetrical flattening of both the inspiratory and expiratory limbs. (Fig 6.8) This is because airflow is limited in both directions, and not significantly affected by intrathoracic pressure changes.
Cardiopulmonary Exercise Testing
Cardiopulmonary exercise testing (CPET) may be used as a preoperative risk stratification tool to predict post-operative outcomes in patients awaiting elective surgery.
Unlike other investigation tools, CPET simultaneously measures cardiac, respiratory and musculoskeletal function to identify where limitations arise and reliably determines cardiorespiratory fitness. CPET involves patients exercising on a treadmill or cycle ergometer with ongoing measurements of variables such respiratory and heart rate, oxygen saturation, cardiac output and maximal oxygen consumption (VO2max) (Agnew, 2010). CPET objectively quantifies the work rate at which the end-organ oxygen demand exceeds oxygen delivery, which is a marker of the participants’ functional reserve capacity. The point at which CO2 production exceeds the participants’ VO2max is known as the anaerobic threshold (AT).
Patients are initially assessed in a baseline resting state for 3 minutes. The patient then starts pedalling without any resistance for 1–3 minutes before resistance is added to the cycle ergometer at a predetermined rate. The rate of work increment during the CPET is determined by set formulae outlined next so that the CPET is standardised between 6 and 10 minutes. Patients can terminate the testing at any time, but should be provided with verbal encouragement to reach a true AT where possible (Agnew, 2010).
Work rate formulae (Agnew, 2010):
Work rate increment = peak VO2 –VO2 unloaded /100 (Wmin−1)
Peak VO2 men = height (cm)-age (yr) × 20 (mL min−1)
Peak VO2 women = height (cm)-age (yr) × 14 (mL min−1)
A reduced preoperative fitness level is indicated by a low AT and has been associated with an increased incidence of perioperative complications. Older and colleagues identified a cut-off AT value of 11 mL/kg/min below which there was a significant increase in the perioperative mortality of elderly patients undergoing major abdominal surgery (Older et al., 1993). The same AT cut-off was also identified as a useful tool to risk-stratify the need for post-operative critical care beds (Older, Hall and Hadder, 1999). In a recent study of 506 patients undergoing abdominal aortic aneurysm (AAA) repair, an AT <10.2 mL kg−1 min−1 was an independent predictor of 30-day mortality whereas a peak VO2 <15 mL kg−1 min−1 was a predictor of 90-day mortality. Patients managed CPET testing with AAA of 63.1±10.1mm (Grant et al., 2015).
For patients with lung cancer considering radical treatment with lung resection, CPET has also been used to risk-stratify post-operative outcomes. It is suggested that a VO2max of less than 50–60 per cent predicted increases in the perioperative mortality risk. Patients who can achieve a VO2 max >20 mL/kg per minute are likely to have an acceptable rate of post-operative complications. Those with a value <10 mL/kg per min are probably best managed non-surgically. For those with VO2 max values in between 10 and 20 mL/kg per minute, surgical resection is not absolutely contraindicated, but the patient must be counselled about the higher risk of post-operative complications (Wyser et al., 1999).
Other variables measured with CPET include minute ventilation, tidal volume, respiratory rate, ventilatory equivalents for oxygen and carbon dioxide and the oxygen delivered per heart beat, which may assist in identifying respiratory or cardiac exercise limitation. ECG evidence of cardiac ischaemia with incremental workload is also an important indicator of perioperative myocardial infarction risk.
CPET is non-invasive and has been demonstrated to be safe in high-risk patient groups where the risk-benefit balance of surgery is unclear. Interest is now growing in using CPET to assess the role of training to improve cardiorespiratory fitness prior to surgery (Agnew, 2010).
Contraindications to CPET can be divided into absolute and relative shown in the table (ATS/ACCP Statement on Cardiopulmonary Exercise Testing, 2003) (Table 6.1).
Table 6.1 Contraindications to cardiopulmonary exercise testing.
Absolute | Relative |
---|---|
Acute myocardial infarction (3–5 days) | Left main coronary stenosis or its equivalent |
Unstable angina | Moderate stenotic valvular heart disease |
Uncontrolled arrhythmias causing symptoms or haemodynamic compromise | Severe untreated arterial hypertension at rest (>200mmHg systolic, >120mmHg diastolic) |
Syncope | Tachyarrhythmias or bradyarrhythmias |
Active endocarditis, myocarditis or pericarditis | High degree atrioventricular block |
Symptomatic severe aortic stenosis | Hypertrophic cardiomyopathy |
Uncontrolled heart failure | Significant pulmonary hypertension |
Acute pulmonary embolus or pulmonary infarction | Advanced or complicated pregnancy |
Thrombosis of lower extremities | Electrolyte abnormalities |
Suspected dissecting aneurysm | Orthopaedic impairment that compromises exercise performance |
Uncontrolled asthma | |
Pulmonary oedema | |
Room air desaturation at rest < or = 85% | |
Respiratory failure | |
Acute non-cardiopulmonary disorder that may affect exercise performance or be aggravated by exercise (i.e. infection, renal failure, thyrotoxicosis) | |
Mental impairment leading to inability to cooperate |
Although CPET has been established as the most reliable and objective assessment of functional capacity, for those in whom CPET is not feasible/available, or for those in whom AT is not reached, alternative assessments should be considered. Examples include questionnaires such as the EuroSCORE questionnaire for cardiac operative risk evaluation, and the Duke Activity Status Index, alongside conventional measures of lung function and echocardiography.
The distance achieved in functional walk assessments such as the 6-minute walk test and the incremental shuttle test have been shown to correlate well with CPET assessments. However, the precise cut-offs predictive of morbidity remain ambiguous, with significant variability identified between the different studies. A preoperative 6-minute walk distance of less than 300 m was associated with a poor prognosis following aortic valve replacement (de Arenaza et al., 2010), whereas an incremental shuttle test performance of more than 350 m was associated with a low mortality post oesophagogastrectomy, and above 250 m was associated with reduced mortality following major abdominal surgery (Murray et al., 2007; Nutt and Russell, 2012).
Asthma
Asthma is a chronic inflammatory disorder of the airways within the lungs due to complex interactions between inflammatory cells, mediators and airways cells. This leads to airway hyper-reactivity on exposure to a variety of stimuli, leading to a variable degree of airway obstruction. This obstruction can reverse spontaneously (with removal of the stimulus) or with treatment.
The clinical features of asthma include cough, shortness of breath, wheeze and chest tightness. Classically these are intermittent, worse on exercise and in the early hours of the morning and associated with particular stimuli (e.g. house dust mites, pollen, cat dander etc.).
The diagnostic hallmark of asthma is the presence of reversible airways obstruction. It is therefore useful to see if there is any change in the airway indices following administration of a bronchodilator such as 2.5 mg of nebulised salbutamol. A positive response in adults is defined as a 12 per cent increase in baseline (pre bronchodilator) FEV1, with an increase of 200 mL or more following the administration of a bronchodilator. A negative test does not necessarily mean that the patient will not derive any benefit from bronchodilator therapy. This is when the patient’s history, examination and home PEFR charts become useful in guiding treatment.
If the diagnosis of asthma is uncertain, bronchial challenge testing with bronchoconstrictors such as methacholine may be considered. A 20 per cent fall in FEV1 in response to small doses of a bronchoconstrictor is indicative of asthma.
If the diagnosis of asthma is secure, patients will tend to be on inhaled therapy in the form of short- or long-acting bronchodilators, and possible inhaled corticosteroids. A diagram illustrating the recommended stepwise management of asthma can be seen next. (Fig 6.9)
If a patient with asthma is seen preoperatively, and is stable and compliant with his or her established treatment, it is important for him or her to be prescribed and continue the inhaled therapy till surgery. This is with the exception of theophylline, which should be discontinued the evening prior to surgery. Continuation of inhaled medications, including inhaled corticosteroids, has been shown to reduce the incidence of post-operative pulmonary complications. It is important to note that patients with controlled or stable asthma may have apparently normal spirometry or flow volume curves.
If a patient with asthma is less stable (with a PEFR that is below his or her best), then it is prudent to eliminate the possibility of an infectious or non-infectious exacerbation. If this proves not to be the case, then a good history and examination should provide confirmation of a secure diagnosis and a glimpse into the severity of the disease (Table 6.2). Questions about allergen exposure and compliance with treatment are essential. Checking the patient’s inhaler technique is also crucial. Once this is done, and the patient’s treatment is optimised according to a stepwise management plan, the patient needs to be given a period of time (at least 2 weeks) to continue on his or her established therapy. Repeat testing can then be carried out looking for improvement. If there is little or no improvement, seeking the specialist advice of a respiratory physician is recommended.
Legend: PEFR=peak expiratory flow rate, A & E=accident and emergency, ATS=American Thoracic Society, ACCP=American Cardiothoracic Society.
In general, a PEFR ≤80 per cent of predicted should be evaluated further with spirometry, unless the value is known to be consistent with good control in that individual. While formal guidelines are lacking, the approach to the perioperative management of asthma is based on the clinician’s assessment of the severity and degree of control of the patient’s asthma, based on history, examinations and investigations performed.
For patients with asthma who are not well controlled and will require endotracheal intubation for high-risk surgery, a course of supplemental glucocorticoids administered preoperatively can be helpful. This usually is in the form of prednisolone 40 mg once daily for 5 days. Intravenous hydrocortisone (100 mg every 8 hours) can be used as an alternative for patients unable to tolerate oral medications. This recommendation is based on a prospective study of inpatients with untreated, severe bronchospasm which showed that 5 days of oral steroid therapy along with 5 days of inhaled short-acting beta agonist significantly decreased post-intubation bronchospasm compared with either medication alone (Silvanus, Groeben and Peters et al., 2004). Studies have also demonstrated that systemic glucocorticoids have not been shown to increase post-operative infection, wound infection, delayed wound healing or asthma exacerbation (Su et al., 2004).
Patients with severe refractory allergic asthma are now treated with a monoclonal antibody to immunoglobulin E (Ig-E) called omalizumab. Omalizumab is given subcutaneously every 2 to 4 weeks. The dosage is determined by the concentration of serum Ig-E and body weight. If patients undergoing Omalizumab therapy are seen preoperatively, it is important that they continue with this therapy in the perioperative period, in order to decrease the risk of pulmonary complications secondary to asthma.
Chronic Obstructive Pulmonary Disease
COPD is characterised by fixed airflow obstruction. There is minimal or no reversibility with bronchodilators and little variation in day-to-day symptoms. There is usually a slowly progressive and irreversible variation in lung function, leading to progressively worsening symptoms. About 95 per cent of COPD is caused due to smoking, but other causes include environmental exposure to dusts, chemicals and pollutants.
In COPD there is mucus gland hyperplasia, squamous metaplasia and loss of cilial function. This, together with the chronic inflammation and fibrosis of small airways and emphysema due to alveolar wall destruction, leads to the clinical features of dyspnoea, wheeze, productive cough and decreased exercise tolerance. Patients with severe COPD can become chronically hypoxic over a period of time. This precipitates pulmonary arteriolar wall remodelling, which can lead to increased pulmonary vascular resistance (PVR) and pulmonary hypertension.
COPD is a clinical diagnosis that is characterised by a minimally reversible (<10% on bronchodilator testing), obstructive pattern on spirometry and flow volume curves (see Figures 6.2 and 6.5). The FEV1 is the measurement of choice to assess the progression of COPD. The FEV1 can be expressed as a percentage of the predictive value, which allows classification of the severity of the impairment (Table 6.3).
Table 6.3 GOLD classification the severity of COPD.
COPD Stage | Severity | Postbronchodilator FEV1/FVC | FEV1 % pred |
---|---|---|---|
0 | At risk # | >0.7 | ≥80% |
I | Mild COPD | ≤0.7 | ≥80% |
II | Moderate COPD | ≤0.7 | 50%–80% |
III | Severe COPD | ≤0.7 | 30%–50% |
IV | Very severe COPD | ≤0.7 | <30% |
Legend: FEV1 = forced expiratory volume in first second, FVC = forced vital capacity, GOLD = global initiative for chronic obstructive lung disease, COPD = chronic obstructive pulmonary disease, % = per cent
The FEV1, however, is known to correlate weakly with the degree of dyspnoea and a decline in the patient’s health. This has precipitated the development of a simple multidimensional grading system for COPD, using BMI, airflow obstruction, dyspnoea and exercise capacity as scoring variables. (See Table 6.4.) It has been shown to be better than FEV1 alone at predicting risk of hospitalisation and death in patients with COPD (Celli et al., 2004).
Table 6.4 Calculating the BODE score.
Point on BODE index* | ||||
---|---|---|---|---|
0 | 1 | 2 | 3 | |
FEV1 (% of predicted) | > or = 65 | 50–64 | 36–49 | < or = 35 |
Distance walked in 6 minutes (m) | > or = 350 | 250–349 | 150–249 | < or = 149 |
Dyspnoea scale score | 0–1 | 2 | 3 | 4 |
Body mass index measure | >21 | < or = 21 | – | – |
* Values range from 0 (best) to 10 (worst)
Legend: BODE = body mass index, obstruction, dyspnoea, exercise, FEV1 = forced expiratory volume, % = per cent, m = metre
Management of COPD normally requires a multidisciplinary team approach and includes smoking cessation, patient education, pulmonary rehabilitation, diet and psychosocial support. Smoking cessation is the only intervention proven to decrease smoking-related decline in lung function. Patients should be encouraged to stop smoking in the perioperative period, with the assistance of adequate nicotine replacement therapy.
Pharmacological management of COPD is usually in the form of inhaled therapy, including bronchodilators and corticosteroids. Please see Figure 6.10. Oral steroids are used less as maintenance therapy, but more to treat infective and non-infective exacerbations. Patients with severe COPD need oxygen either as symptomatic relief on exertion, or as long-term oxygen therapy (LTOT). The latter is for patients in respiratory failure, with a PaO2 <7.3kPa or a PaO2 of 7.3–8kPa with signs of secondary polycythaemia or pulmonary hypertension. Patients are asked to use LTOT for 15 hours a day. Small changes in oxygen retention with oxygen therapy can be tolerated in an asymptomatic patient with no respiratory acidosis.
Patients with severe COPD, with severe distal airway collapse, are generally poorly tolerant of surgery. This is because in these patients, mechanical ventilation is associated with a requirement for increased inflation pressures, which can lead to barotrauma and pneumothoraces. This increased inflation pressure leads to increased intrathoracic pressure, which leads to decreased venous return to the heart, and therefore decreased cardiac output. This, in patients who have been long-term smokers and therefore have a generalised vasculopathy, can precipitate ischaemia in the brain, gut, liver or kidneys (precipitating renal failure).
A review of the literature has revealed a retrospective cohort study in which patients with severe COPD, when compared with patients who did not have COPD, were six times more likely to have major post-operative pulmonary complications after abdominal or thoracic surgery (Kroenke et al., 1993). Important predictors of post-operative pulmonary complications, in a multivariate regression model, were age 65 or greater (OR 1.8), smoking 40 pack-years or more (OR 1.9) and maximum laryngeal height of 4 cm or less (distance from the top of the thyroid cartilage to the suprasternal notch at end of expiration, which is known to be an accurate sign of COPD) (Straus et al., 2000).
Therefore when preoperatively assessing a patient with COPD, it is not only important to obtain a history and examination and consider factors such as smoking history and environmental dust exposure, current smoking status, use of inhaled therapy, inhaler technique, frequency of exacerbations; attention also needs to be paid to the patient’s BMI, dyspnoea (can be quantified using the Medical Research Council scale) and functional exercise capacity. This together with data acquired from spirometry and flow volume curves, the pO2 and pCO2 on an arterial blood gas (ABG) and an echocardiogram can guide the clinician with regards to the risk of post-operative pulmonary complications.
In patients with severe COPD (as defined by FEV1 criteria or BODE score), it is useful to establish them on nebulised bronchodilator therapy (salbutamol and ipratropium bromide) and inhaled corticosteroids in the perioperative period. If patients are established on LTOT, they need to continue this in the perioperative period. If they have a tendency towards respiratory acidosis, post-operatively they will need weaning onto non-invasive ventilation (normally, bi-level ventilation with positive airway pressure). In this case they might need to be recovered in a suitable clinical setting – be that in a high-dependency unit (HDU) or intensive care unit (ICU), which will need to be booked beforehand. In these cases it is essential to know their baseline pO2 and PCO2 to define treatment goals.
Mucolytics and respiratory physiotherapy are essential for patients post-operatively. This is to decrease the probability of post-operative atelectasis and pneumonia.
Smoking Cessation
Preoperative smoking was associated with an increased risk of post-operative complications, including pulmonary complications (RR 1.73, 95% CI 1.35–2.23), in a 2014 meta-analysis of 107 cohort and case-control studies (Grønkjær et al., 2014). Smokers with a greater than 20 pack-year smoking history have a higher incidence of post-operative pulmonary complications than those with a lesser pack-year history.
Smoking is a known risk factor for impaired wound healing and increases the risk of post-operative wound infection. A randomised control trial assessing the impact of smoking cessation on wound-related complications in 120 patients identified a significant reduction incidence of complications in the smoking cessation group (5 vs. 31%, p = 0.001) (Theadom and Cropley, 2006). Furthermore, it has been suggested that stopping smoking as little as 4 weeks before surgery may reduce post-operative complications, and smoking cessation 6–8 weeks prior to surgery had a beneficial effect on wound healing (Lindstrom et al., 2008; Theadom and Cropley, 2006).
Motivating patients to stop smoking is challenging, and currently there is no clear proven method to enhance patients’ adherence. It is clear that nicotine replacement therapy is often a useful adjunct alongside a supervised nicotine withdrawal program. The choice of nicotine replacement therapy is best tailored on an individual basis to suit patients’ needs. No single method of nicotine replacement treatment has demonstrated to be more effective than another.
Further information about the lung function of patients with COPD can be acquired through more detailed tests. These are called pulmonary function tests (PFTs). The interpretation of PFTs requires knowledge of respiratory physiology. In the following section we will look at these tests more closely.
Pulmonary Function Tests
Indications for pulmonary function tests are listed in the table that follows (Table 6.5).
Table 6.5 Indications for pulmonary function tests.
Performing pulmonary function testing is generally safe, but specific contraindications exist. These are listed in Table 6.6. Pulmonary function testing is generally effort dependent and therefore pre-testing counselling to stress its importance and impact is essential in order to obtain patient cooperation and reproducible and optimal results.
A sitting position is typically used at the time of testing. This is to prevent the risk of injury and a fall in the event of a syncopal episode. Patients are advised not to eat a large meal 2 hours before testing, not to smoke for at least 1 hour of testing and not to wear tight clothing, as under these circumstances results might be affected. False teeth are left in place unless they prevent the patient from forming an effective seal around the mouthpiece. The PFTs should be performed three times to ensure that the results are reproducible (less than 200 mL variation) and accurate. Dynamic studies are performed first (spirometry, flow volume curves, PEFRs), followed by lung volumes and diffusion capacity.
Dynamic testing has been previously discussed. Static lung volumes can be measured through the use of whole-body plethysmography (most common), nitrogen washout or helium dilution. In body plethysmography, the patient sits in an airtight box. He or she inhales or exhales to a particular volume (usually the functional residual capacity (FRC) – the volume of air in the lungs following maximal expiration). Then a shutter drops across the breathing tube. The patient makes respiratory efforts against the closed shutter. Measurements are then taken. These are based on Boyle’s law, which states that the volume of a given mass of gas varies inversely with pressure, at constant temperature. Therefore the increase in the volume of the patient’s chest decreases the volume of air in the box surrounding the patient, thereby increasing the pressure. Static lung volumes can be obtained either by measuring the changes in pressure in a constant volume box, or volume in a constant pressure box.
One such volume that is measured is the residual volume (RV), which is the amount of air remaining in the lungs after a maximal expiration (Figure 6.11). In patients with obstructive lung diseases where there is significant air trapping, the RV will be increased. Patients with a high RV who require surgery and mechanical ventilation require high perioperative inflation pressures. This may result in barotrauma, a pneumothorax and reduced venous return due to high intrathoracic pressures. The RV can also be expressed as a percentage of total lung capacity (TLC – the total volume in the lungs after a maximal inspiration); and values in excess of 140 per cent significantly increase the risks of these complications (Wilde, Nair and Madden, 2007).
TLC is the sum of the RV and vital capacity (the difference in volume of air in the lung between maximal inspiration and maximal expiration). The TLC may therefore be increased in patients with obstructive defects such as emphysema and decreased in patients with restrictive abnormalities, including chest wall abnormalities and kyphoscoliosis.
The measurement of the diffusion capacity of carbon monoxide (DLCO, also known as transfer factor) gives important information regarding the surface area and integrity of the alveolar capillary membrane. Carbon monoxide gas is used because it is soluble, it binds to haemoglobin and its uptake is limited by diffusion only. Therefore the DLCO is determined by the thickness and surface area of the alveolar membrane, and the pulmonary vascular bed. The value is frequently corrected for the patient’s haemoglobin (DLCOc). It is measured by a single-breath technique where 10 per cent helium and 0.3 per cent carbon monoxide are rapidly inspired, held for 10 seconds and then expired, with measurement of the remaining carbon monoxide by an infrared analyser. Comparison of the inspired and expired CO concentrations allows calculation of DLCOc (Ranu, Wilde and Madden, 2011).
DLCOc is reduced in conditions such as emphysema, pulmonary fibrosis or pulmonary emboli, where the surface area of the alveolar membrane is reduced. The transfer coefficient (KCO) is calculated. This is the DLCOc corrected for alveolar volume. In patients with a pneumonectomy, DLCOc will be reduced due to the loss of approximately half of the surface area of the alveolar membrane, but KCO will be normal. The remaining lung has normal function of the alveolar capillary membrane. In this way, the reduced DLCO is appropriate for the loss of alveoli units. In pulmonary diseases that have a heterogeneous effect on the lungs, such as COPD (upper lobe predominance), alpha 1 antitrypsin emphysema (lower lobe predominance), interstitial lung disease or pulmonary oedema, the DLCOc will be lower than KCO. Pulmonary emboli should be considered in patients with an isolated reduction in DLCOc without any other obvious respiratory cause, and should be investigated with a CT pulmonary angiogram.
A normal DLCOc with an elevated KCO may suggest an extra-pulmonary restrictive defect such as chest wall disease, respiratory muscle weakness and obesity. Finally, DLCOc may be elevated in pulmonary haemorrhage, asthma and left-to-right cardiac shunts. Description of pulmonary function tests in number of pulmonary diseases is provided in the table that follows (Table 6.7).
In patients with a reduced DLCOc due to an interstitial lung disease, both mechanical ventilation and post-operative weaning can prove challenging. The decreased lung compliance can lead to the use of increased inflation pressures when the patient is ventilated, which might precipitate perioperative complications, including pneumothoraces. Patients undergoing procedures such as coronary bypass grafting can be prone to an inflammatory driven acute respiratory distress syndrome (ARDS), which in itself can be extremely challenging to manage.
If a patient with suspected interstitial lung disease is seen preoperatively, it is essential to ensure that a formal diagnosis is made, once the relevant investigations are carried out, usually under the guidance of a respiratory physician. The patient might need to be initiated on immunosuppression or anti-inflammatory agents, depending on the underlying aetiology and severity of the interstitial lung disease. Only then should the patient be considered for cardiothoracic surgery.
Preoperative pulmonary function testing for risk stratification is highly controversial. The literature suggests that these tests in most cases just confirm the clinical impression of disease severity, and add little to the clinical estimation of risk. Concerns have therefore been raised about the overuse of preoperative PFTs, and consequently cost-effectiveness. The American College of Physicians guideline recommends that clinicians not use preoperative spirometry routinely for predicting the risk of post-operative pulmonary complications, based on a systematic review in 2006 (Qaseem et al., 2006). However, it is important to note that these recommendations were based on studies that are now relatively old (1989 and 1997), and focus on spirometry in patients undergoing abdominal surgery. Therefore these findings might not necessarily apply to patients undergoing other types of surgery.
A sensible approach thus needs to be adopted when dealing with PFTs. They need not be ordered routinely prior to surgery, and should not be used as a primary factor to deny surgery. However, in a patient with asthma or COPD, when clinical evaluation alone proves insufficient to optimise patients, PFTs may identify patients who will benefit from more aggressive preoperative management. Also, when a patient is unduly breathless or has poor preoperative exercise capacity, PFTs are useful in ruling out pulmonary disease and identifying cardiac disease, deconditioning or, indeed, pulmonary hypertension.
Table 6.7 Pulmonary function in different pulmonary disease groups.
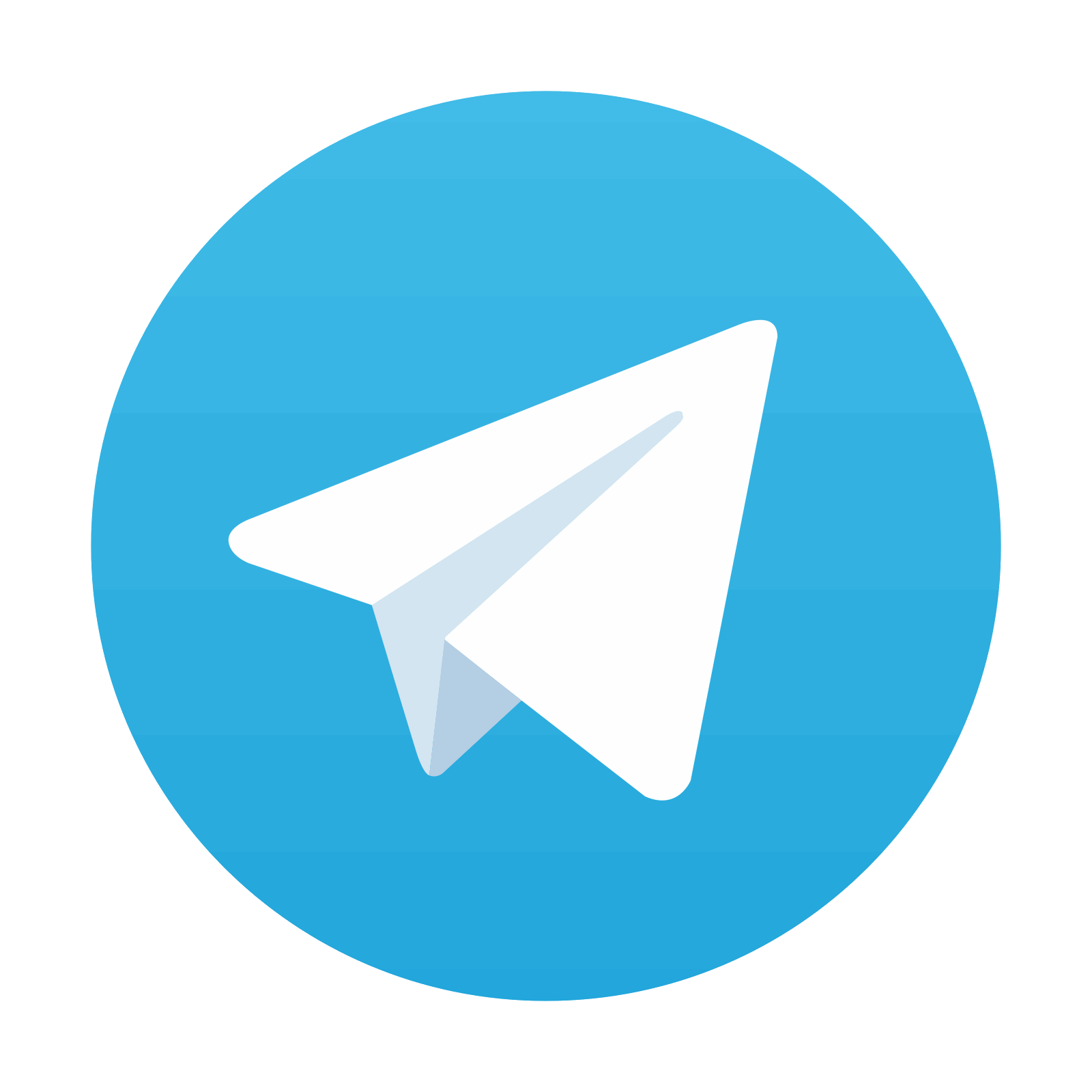
Stay updated, free articles. Join our Telegram channel

Full access? Get Clinical Tree
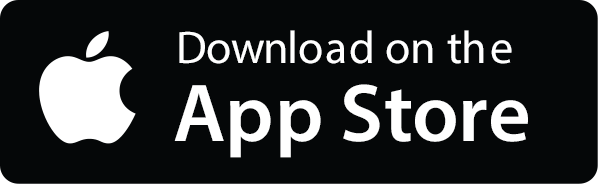
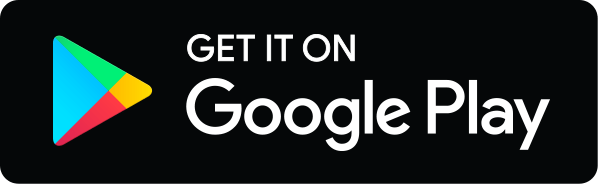
