Introduction
Despite advances made in surgical techniques, infants and children undergoing neurosurgical procedures remain at increased risk for perioperative bleeding and frequently require blood product transfusions. Among the surgeries performed, hemispherectomies, craniotomies, and cranioplasties are associated with the highest blood product transfusion rates.1, 2 Multimodal and multidisciplinary bleeding management approaches have been developed over the past decade and aim to maintain hemodynamic stability, preserve oxygen carrying capacity, and define optimal transfusion strategies based on both surgical and patients’ characteristics. Prevention, detection, and goal-oriented treatment of hyperfibrinolysis and coagulopathy play a central role and are imperative in improving outcome. In this chapter, we review the background and the step-by-step approach for the prevention and management of significant blood loss in infants and children undergoing neurosurgical procedures.
Scope of Neurosurgical Bleeding
Among the surgical procedures regularly performed in children, hemispherectomies, tumor resection, traumatic subdural/epidural hematoma decompression, resection of cerebral aneurysms, and arteriovenous malformations present the highest risk for significant blood loss, with high exposure rates to allogeneic blood products. Highly vascular neoplasms, such as meningiomas or glomus tumors, can be associated with substantial blood loss. Tumor involvement and extension into cranial sinuses and veins increase the likelihood of massive blood loss. In children undergoing craniotomy for brain tumor resection, age <4 years, surgery duration >270 minutes, and preoperative hemoglobin <12.2 g/dL have been defined as important predictors for massive bleeding and blood products transfusion.3 Despite improvements in surgical techniques such as functional vs. anatomical hemispherectomy, blood loss for hemispherectomies can exceed more than one blood volume.4, 5 Cranial vault remodeling for craniosynostosis repair is associated with substantial bleeding, with blood losses varying between 0.5 to 4 blood volumes.6 In this specific population, age <18 months, body weight <10 kg, American Society of Anesthesiologists (ASA) physical status classification ≥ 3, craniofacial syndromes, pansynostosis, operating time > 5 hours, redo operations, and the presence of high intracranial pressure have been shown to significantly increase the bleeding risk.7
Etiology of Hemostatic Derangement during Surgery in the Bleeding Child
Hemostatic changes observed during pediatric neurosurgery may be caused by a variety of factors. In children less than 1 year old, the potential immaturity of the hemostatic/coagulation system can increase perioperative bleeding. Indeed, some pivotal clotting factors are either not effectively produced or not fully active, minimizing the abilities of natural clotting and, therefore, increasing perioperative bleeding.8 For example, levels of coagulation factors II, VII, XIII are reduced to about 50% of normal adult values at birth.9 Neonatal platelet levels are similar to normal adult values at birth, but platelet function is decreased. In addition, other factors, like preexisting coagulation disorders (e.g., acquired or congenital), intraoperative activation of fibrinolysis, tissue factor activation through large surfaces of tissue injury, activation of coagulation and consumption of coagulation factors (e.g., fibrinogen), and the presence of surgical bleeding may lead to a significant amount of blood loss that further activates coagulopathy through the increased loss of coagulation factors and the development of a dilutional coagulopathy.
Neurosurgical patients may have a hypercoaguable state perioperatively.10 However, during major neurosurgery, the coagulation and fibrinolytic systems are activated and the delicate balance between clot formation and clot lysis can be disrupted, increasing clot instability and bleeding tendency. Tissue injury and destruction of the blood-brain barrier can lead to vascular liberation of tissue activators (tissue plasminogen activator, tissue thromboplastin, urokinase, and kallikrein) that elicit the conversion of plasminogen into plasmin, an important trigger of the fibrinolytic activation.11 When activated, the fibrinolysis decreases clot stability and increases bleeding tendency, by increasing consumption of fibrinogen and coagulation factors. In addition, plasmin induces many other responses that contribute to coagulopathy and bleeding, including activation of thrombin generation, platelets, and the inflammatory response. Together, this cascade can place the child into a vicious cycle of deteriorating hemostasis12 (Figure 5.1).
Figure 5.1. Cycle of potential hemostatic derangement in major pediatric surgery. BBB = blood-brain barrier; t-PA = tissue plasminogen activator; u-PA = urinary plasminogen activator (urokinase); FDP = fibrin degradation products.
When the child starts bleeding, the administration of crystalloids and/or colloids may lead to a dilutional coagulopathy, defined as the loss and consumption of coagulation factors and the dilution-dependent reduction of these factors in circulating blood. Its severity depends on the degree of blood loss and the concomitant amount and type of fluids administered.13 Acquired fibrinogen deficiency may be the leading determinant in the development of perioperative dilutional coagulopathy; fibrinogen being the first coagulation factor that reaches a critically low value during massive blood loss,14 while platelet count will reach a plateau when 50% of the circulating blood is lost due to the mobilization of the platelets pooled in the body.15 It is also important to keep in mind that extremely severe degrees of hemodilution (e.g., up to 95%) are required before the effect of other clotting factors will be affected, and thrombin generation will be abolished.16
Last but not least, the hemostatic potential might be further deteriorated by hypothermia, acidosis, and hypocalcemia.
Scope and Associated Morbidity and Mortality of Transfusion
In a bleeding child, the transfusion of blood products may be life-saving and crucial in maintaining hemodynamic stability, preserving oxygen delivery to vital tissues and organs, and treating coagulopathy. Given that the most common identifiable cause of anesthesia-related cardiac arrest in children is hypovolemia due to blood loss, timely and appropriate management of blood loss in the bleeding child is imperative.17 However, inappropriate transfusion of blood products may also be associated with nonnegligible complications. Despite improvements in hemovigilance and the significant reduction in the risk of transfusion-related infections, the reported incidence of noninfectious transfusion-associated complications has increased in children.18 The Serious Hazards of Transfusion (SHOT) report states an incidence of 13 adverse events per 100,000 units of red blood cells transfused in adults, while the incidence is 18:100,000 in patients <18 years old and 37:100,000 in children <1 year.19 Furthermore, there is strong evidence that transfusion-related side effects are associated with increased morbidity and mortality in children, with two cases of death reported every 100,000 blood products transfused.20, 21 Transfusion-related acute lung injury (TRALI), transfusion-related acute circulatory overload (TACO), and hemolytic transfusion reactions are the main culprits, with mortality rates as high as 15–30%. Other risks include transfusion-transmitted infection and transfusion-related immunomodulation (TRIM), in addition to the risk of transfusion errors, which remains a major issue. Massive blood transfusion in pediatric trauma, surgery, and critical care has been identified as an independent predictor of multiple organ failure, systemic inflammatory response syndrome, increased infection, and increased mortality.22, 23 Furthermore, transfusion volume strongly correlates with an increased morbidity and mortality.24 As a consequence, to avoid excessive and inappropriate transfusion, all efforts should be made to define preventative measures and ensure early recognition and appropriate, timely treatment of major blood loss. Before each transfusion, a careful assessment of the balance between the risk of nontransfusion, the risk associated with blood product administration, and the effectiveness of the transfusion should be recommended.
Patient Blood Management
Patient blood management (PBM) is the timely application of evidence-based medical concepts designed to optimize hemoglobin concentration, maintain hemostasis, and minimize blood loss through the development of multimodal and multidisciplinary (transfusion medicine specialists, surgeons, anesthesiologists, hematologists, and critical care specialists) management strategies.25 Multimodal preventive strategies are emphasized to identify, evaluate, and manage anemia, to reduce iatrogenic blood losses, and to optimize hemostasis, utilizing pharmacological modalities and electronic ordering decision guidelines for appropriate blood product administration.26
PBM is recognized by the World Health Organization as a means to “promote the availability of transfusion alternatives.”27 To achieve these goals, European and North American health care institutions and accreditation and regulatory agencies have focused on blood management to improve clinical outcomes and patient safety. The United States Joint Commission developed PBM performance measures.28 Following the guidelines published by the European Society of Anaesthesiology,29 the American Society of Anesthesiologists Task Force on Perioperative Blood Management recently published updated guidelines that incorporate PBM initiatives.30 The American Association of Blood Banks and the Society for the Advancement of Blood Management also have well-established guidelines for PBM.31 PBM programs reduce the need for allogeneic blood transfusions, reduce health care costs, and decrease morbidity and mortality.32, 33
Blood Conservations Techniques
Preoperative
Even though the effectiveness of routine anemia screening and the implementation of specific preventive measures for children without anemia-related signs or symptoms has not been demonstrated,34 high-risk neurosurgical children would benefit from the preoperative detection and treatment of anemia. This would allow for a preoperative optimization of the hemoglobin level, through the administration of oral or intravenous iron, sometimes in combination with recombinant human erythropoietin. Anemia is defined as a hemoglobin concentration less that the 5th percentile for the patient’s age.35 At least 25% of preschool-age children in industrialized countries are anemic,36 with a global prevalence in children being 42.6%. The incidence of anemia in hospitalized children has not been reported, but up to 37% of neonates in US hospitals are anemic.37, 38 Severe anemia has a prevalence of 0.9 to 1.5% and is associated with substantially worse mortality and cognitive and functional outcomes. The common etiologies of preoperative anemia in children are nutritional, iron deficiency anemia, and iatrogenic blood loss (due to frequent in-hospital blood draws). Preoperative anemia is associated with increased mortality,39 and has been shown to be an independent risk factor for postoperative mortality in neonates40 (Figure 5.2) and children.41
Figure 5.2. Distribution of preoperative hematocrit for neonates undergoing noncardiac surgery in US hospitals for survivors and nonsurvivors.
Early detection and treatment of preoperative anemia include enteral or intravenous iron therapy. The dose of iron should be calculated based on the iron daily intake, and varies between 3 to 6 mg/kg/day divided in 3 doses. The maximal dose should not exceed 150 to 300 mg per day. Erythropoiesis stimulating agents (ESAs), such as recombinant human erythropoietin, increase the hemoglobin preoperatively, and may be a useful adjuvant in certain situations such as children at higher risk of bleeding and blood product transfusion requirement (e.g., weight < 10 kg, or a syndromic patient with comorbidities). ESA clinical protocols of epoetin alfa 600 IU kg/week subcutaneously for 3–4 weeks in divided doses, with iron, vitamin B12, vitamin E, and folic acid oral supplementation, have been described.42 However, the risk-benefit ratio (including risks of blood transfusion vs. risks of ESA) needs to be taken into account, since no large randomized studies have assessed the safety and efficacy of ESAs in the pediatric neurosurgical population. ESAs may cause rare complications such as red blood cell aplasia, infections, seizures, possible malignancy, hypertension, and venous thrombosis, which have led to its restricted use and a Food and Drug Administration (FDA)-mandated black box warning. Transfusion of blood may be a therapeutic modality to consider, but may also be associated with some important risks. Therefore, alternative strategies should be first and foremost utilized. The frequency and volume of blood draws and invasive procedures should be limited. Reducing blood draws directly correlates with reduced transfusion.
Careful preoperative preparation and optimization such as preoperative tumor vessel or arteriovenous malformation embolization or neoadjuvant chemotherapy, when appropriate, are important preemptive strategies.43 Choosing an endoscopic approach, when feasible, is less invasive than an open operation. Surgical approach planning can be supplemented with preoperative imaging and 3D models of vessels and structures to plan appropriate, less invasive surgical options. A surgical navigation system can also be used to interface with magnetic resonance imaging (MRI) and computed tomography (CT) scans for preoperative planning.
Preoperative autologous blood donation may be offered in an older child. Most programs have a minimum weight of 20 kg, with a usual donation volume between 6 and 10 mL/kg. Disadvantages with this process include issues with rendering the patient anemic preoperatively, incomplete donations, wastage, clerical error, and blood storage issues. This technique is associated with substantial risks and costs, and therefore its routine use is not recommended, and should be limited to very specific conditions (e.g., alloantibodies).44
Intraoperative
During the intraoperative period, a number of options should be used, alone or in combination, to decrease the bleeding risk and the need for blood products transfusion.
Surgical techniques that can help control bleeding include the use of an intraoperative navigation system to localize and define vessels and lesions, minimally invasive surgery or endoscopic surgery, and the use of topical hemostatic agents. Surgical skills should be implemented to maintain meticulous hemostasis and control major vessels. Techniques to decrease bleeding in the surgical field include electric cautery, clips, careful dissection, and proper patient positioning to avoid venous congestion. Infiltration of a local vasoconstrictor and utilizing topical hemostatic agents, such as Gelfoam®, Floseal®, Fibrin Glue®, and Surgicel®, are other adjuncts to minimize local bleeding; however randomized trials to prove efficacy in this area are lacking.45
Intraoperative blood salvage is another option that involves collecting autologous blood from the surgical site to be processed and given back to the patient during the surgery. Recently developed devices have a capacity as low as 30 mL, and are effective in reducing allogeneic blood transfusion in patients weighing as low as 10 kg.46 The use of cell salvage is controversial in cancer surgery due to the potential risk of dissemination of tumor cells through the reinfusion of cell salvaged blood. However, recent studies have suggested that cell salvage can be used safely in cancer surgery47 by utilizing white blood cell depletion filters and irradiating (50 Gy) cell salvage concentrate to minimize risks of tumor cell transfer. Contraindications include the use of agents that would result in red blood cell lysis (sterile water, alcohol, hydrogen peroxide), clotting agents (Surgicel and Gelfoam), contaminants (urine, bone, infection), and irrigating solutions (Betadine).48
Acute normovolemic hemodilution (ANH) and hypervolemic hemodilution (HH) are additional strategies. ANH involves removal of the patient’s blood prior to surgery after induction of anesthesia and replacement with crystalloid or colloid to maintain normovolemia. It may be useful in older children and those who are difficult to cross match due to multiple antibodies. In younger patients this may not be feasible due to hemodynamic instability and anemia, and HH may be an option. HH involves initially hemodiluting the patient’s blood to an acceptable Hct (usually about 25%) with approximately 15 mL/kg of colloid without blood removal and then subsequent fluid restriction during the case. HH is a technique that is useful when the starting Hct is >40% (as with ESA treatment preoperatively).
Anesthetized children are particularly prone to becoming hypothermic. Hypothermia, in combination with acidosis, inevitably leads to an impaired coagulation process and may worsen bleeding. Thus, forced-air warming should be rigorously used and temperature measured continuously.
Monitoring of Coagulopathy and Point of Care
Standard coagulation has been considered as the “gold standard” for the diagnosis of congenital and acquired coagulopathies, and to guide the administration of anticoagulation both in adults and children. Activated partial thromboplastin time (PTT), international normalized ratio (INR), and prothrombin time (PT) remain widely used to assess coagulation status pre-, intra-, and postoperatively. In addition, the standard Clauss method is also used to measure the plasmatic fibrinogen concentration. Unfortunately, those tests were not designed to monitor perioperative coagulopathy or to guide the administration of hemostatic agents in bleeding situations. As it takes 30–45 minutes to obtain the results from standard coagulation assays, limited information is provided by these tests in the context of acute bleeding.49 In addition, standard laboratory tests are performed on platelet poor plasma (PPP) and do not allow for a global assessment of coagulation, giving no information about clot firmness and clot lysis.
Over the past decade thromboelastography (TEG®) and thromboelastometry (ROTEM®) have become more popular for the assessment of coagulation and to guide the administration of blood products in bleeding patients. With the thromboelastometry method, different assays are available and allow for assessment of the intrinsic pathway (INTEM), the extrinsic pathway (EXTEM), and the fibrinogen function (FIBTEM) (Figure 5.3). Thromboelastography also allows for the assessment of the intrinsic activation and the fibrinogen function. More recently, other tests have been developed to assess platelet function. Among the large number of parameters, viscoelastic assays measure clot initiation (e.g., clotting time), clot firmness (e.g., clot amplitude measured after 5, 10, 20, 30 minutes as well as the maximal amplitude), and clot stability (e.g., percentage of lysis after 30 or 60 minutes). This approach allows for a rapid assessment of the coagulopathy, and a recent study suggested that early values of clot amplitudes measured as soon as 5, 10, or 15 minutes after clotting time could be used to predict maximum clot firmness in all ROTEM® tests.50
Figure 5.3. Thromboelastometry (ROTEM) tracings. EXTEM (screening test for extrinsic hemostatic system) and FIBTEM (fibrinogen assay) are shown.
Viscoelastic assays are now integrated in transfusion algorithms, and their use is recommended in all European and American guidelines. In children undergoing neurosurgery, both thromboelastography51 and thromboelastometry52 have been used successfully. A significant decrease in plasma and platelet transfusions after the implementation of a transfusion algorithm based on thromboelastometry and the use of factor concentrates (e.g., fibrinogen concentrate and concentrate of FXIII) have recently been reported.53
Antifibrinolytics
Antifibrinolytic drugs include the lysine analogs that competitively inhibit the activation of plasminogen to plasmin, tranexamic acid (TXA) and epsilon aminocaproic acid (EACA). TXA is the most common antifibrinolytic agent used since EACA is not available in many countries (such as Canada, New Zealand, and most of Europe). There is strong evidence that the implementation of a PBM strategy involving intraoperative prophylactic administration of TXA (and weaker evidence for EACA) is useful for decreasing blood loss and transfusion requirement in children undergoing major surgery12 including craniofacial surgery.54, 55 TXA has been used successfully in high-risk pediatric neurosurgical tumor surgery.56 Even though different dose schemes have been described, recent pharmacokinetic data57 and pharmacodynamic data58 support the use of lower doses. Based on recent data, a TXA dose of 10 mg/kg LD followed by a continuous infusion of 5 mg/kg/hr is recommended57 (Figure 5.4). Regarding EACA, a loading dose of 100 mg/kg followed by a continuous infusion of 40 mg/kg/hr is recommended in infants undergoing craniofacial surgery.59 A retrospective study supports the efficacy of EACA craniofacial surgery, but a well-designed prospective trial is yet to be done.60
Figure 5.4. Tranexamic acid plasma concentration curve over time in pediatric craniofacial surgery. Dosage recommendations shown (A–D) for target tranexamic acid plasma levels 20 μg/mL and 50 μg/mL. Recommended dose of 10 mg/kg loading dose and 5 mg/kg/hr infusion for the duration of the surgery (5 hours on average).
TXA and EACA have side effects such as seizures; the incidence is low and likely dose related. Antifibrinolytics should be considered if massive blood loss is anticipated such as in hemispherectomy surgery. TXA has been used successfully in choroid plexus papilloma resection surgery, a highly vascular tumor of infancy, as part of a multimodal blood conservation strategy.56 There may be a role for TXA in treating subarachnoid hemorrhage in the prevention of rebleeding and improvement in neurological outcome, and an ongoing trial may answer this question.61, 62 Investigating further the efficacy, safety, appropriate dosing scheme, and pharmacokinetic profile of antifibrinolytics in children having neurosurgical procedures with a high potential for bleeding will have a positive impact on patient care.
Fluid Management
The goal of intraoperative fluid management should be to maintain normovolemia while avoiding hypervolemia, to minimize swelling, edema, and hemodilution. Recent studies have suggested that an intraoperative positive fluid balance may increase the need for transfusion and the incidence of postoperative fluid overload, both shown to significantly affect patients’ outcomes.63–65 Even though most of these studies were performed in children undergoing cardiac surgery, fluid overload should be avoided in children undergoing neurosurgery, and a careful monitoring of fluid administration should be recommended. Normal saline is commonly used as maintenance in intracranial neurosurgical procedures as it is mildly hyperosmolar (308 mOsm) and therefore may minimize brain edema.66 However, if given in large volumes (> 60 mL/kg), it may lead to hyperchloremic metabolic acidosis.67 Ringers lactate (273 mOsm) and PlasmaLyte (294 mOsm) are preferred especially when used in extracranial cases as they are associated with less severe acidosis. While some data suggested that colloid administration is associated with better hemodynamic effect with a less positive balance,68 most of the studies were performed in children undergoing cardiac surgery and critically ill patients, and no randomized controlled trial has reported the superiority of colloids over crystalloids for perioperative fluid management in children undergoing neurosurgical procedures.29 Certainly in the scenario of massive blood loss, colloids may have the advantage over crystalloids to better replace the volume lost and better restore normovolemia, preventing and treating hypotension together with other adjuncts such as vasopressors and blood product transfusion. Notably, in patients with a disrupted blood-brain barrier, caution regarding the liberal use of colloids should be mentioned, as the SAFE (Saline versus Albumin Fluid Evaluation) trial showed that in adult intensive care unit (ICU) patients, colloid administration was associated with a higher mortality in the head trauma subgroup.69
Transfusion of Red Blood Cells
While the international blood transfusion guidelines recommend against a single transfusion trigger, maintenance of a hemoglobin (Hb) level >8 g/dL is an accepted standard intraoperatively in an actively bleeding child,29, 30 while in stable critically ill or anesthetized children, a threshold of 7 g/dL is sufficient.70 This trigger is supported by recent trials reporting no difference in morbidity and mortality when a restrictive transfusion strategy was compared with a more liberal one in critically ill children.71, 72 Caution should be mentioned regarding neonates as the optimum transfusion Hb trigger has not been determined by evidence-based prospective trials and a higher target may be necessary.73
Preoperative calculation of the circulating blood volume could help clinicians to estimate the importance of the blood loss, and help guide the administration of fluids and blood products. The blood volume of a preterm infant is 90–100 mL/kg, while the blood volume of a term neonate is 80–90 mL/kg. Above 3 months and until 1 year of age the blood volume will vary between 70 and 80 mL/kg, and will be considered as being 70 mL/kg in children older than 1 year. In addition to the estimation of the child’s blood volume, the maximum allowable blood loss (MABL) is usually calculated using the circulating blood volume (CBV), the initial hematocrit or hemoglobin, and the minimum acceptable value: MABL = CBV × (initial hematocrit – minimum acceptable hematocrit) / initial hematocrit.
This approach allows for the determination of the minimum hematocrit or hemoglobin tolerable for each patient and should be based on the child’s comorbidities, ability to maintain adequate cardiac output and oxygen delivery, metabolic needs, and tolerance to anemia. In addition to the MABL, the CBV can also be used to estimate the volume of allogeneic red blood cell needed to reach a certain hematocrit or hemoglobin level.
RBC needed = (targeted hematocrit – current hematocrit) × CBV / hematocrit of the packed red blood cell.
Transfusion volume should be limited to the volume required to achieve a certain target and to correct intraoperative acute anemia. Overtransfusion should be avoided as adverse events are directly related to the volume administered, with higher incidences of complications reported following the administration of 40 mL/kg or more.74 As a rule of thumb, the required transfusion volume in children could also be calculated as follows: bodyweight (kg) × desired increment in Hb (g/dL) × 5. The goal is to restore/maintain tissue oxygenation to vital organs and tissues and to consider the clinical status of the patient.
Transfusion of Platelets
In children undergoing neurosurgery, a preoperative platelet count varying from >50,000 to 100,000 cells/µL is suggested based on the bleeding risk, the type of procedure, and the patient’s comorbidities. In case of active bleeding, platelet transfusion is recommended to maintain a minimal platelet count above 80,000 cells/µL. Platelet count can be expected to rise by approximately 50,000 cells/µL after transfusion of 5–10 mL/kg of an apheresis platelet concentrate. The transfusion of platelet concentrate carries the highest risk of side effects of all allogeneic blood products (bacterial contamination of platelet components is the second most common cause of transfusion-related deaths) and therefore should be performed cautiously.
Transfusion of Fresh Frozen Plasma
Accepted practice guidelines suggest replacing coagulation factor deficiency with fresh frozen plasma (FFP) when there is ongoing clinical microvascular bleeding and the PT >1.5 times normal, or INR>2, or PTT >2 times normal or correction of excessive microvascular bleeding secondary to coagulation factor deficiency in patients transfused more than one blood volume (when laboratory values can’t be obtained in a timely fashion) at a dose of 10–15 mL/kg.30 However, this value may be too low, and therefore expert consensus recommends as much as 15–30 mL/kg FFP to replace coagulation factors in an actively bleeding child. Recent European guidelines highlight that while FFP transfusion is recommended to treat severe bleeding by several guidelines, high-quality evidence is lacking regarding the indication and dosing.29 Certainly, severe risks have been reported including the noninfectious serious hazards of transfusion: TRALI, TACO, TRIM, and multiple organ failure.
The 2010 American Association of Blood Banks (AABB) Evidence-Based Practice Guidelines for plasma transfusion state, “We cannot recommend for or against transfusion of plasma for patients undergoing surgery in the absence of massive transfusion (quality of evidence = very low).” The AABB recommends that plasma be transfused to trauma patients requiring massive transfusion (quality of evidence = moderate). But the organization “cannot recommend for or against transfusion of plasma at a plasma: RBC ratio of 1:3 or more in trauma patients during massive transfusion (quality of evidence = low).” Furthermore these guidelines state, “It is unclear how this strategy pertains to managing massive blood loss in the pediatric surgical patient.”75
However, prophylactic transfusion of plasma should not be recommended in a nonbleeding child and should certainly not be considered before an invasive procedure (e.g., lines removal) even in the presence of prolonged coagulation tests. Indeed, plasma remains frequently transfused in critically ill children or before a major surgery in the absence of bleeding. A recent audit of plasma transfusions in critically ill patients concluded that one-third of transfused patients were not bleeding and that plasma transfusion significantly improved the INR only in those patients with a severe derangement, indicated by an INR > 2.5.76 Furthermore, coagulation tests are not sensitive to the increase in coagulation factors resulting from plasma transfusion. FFP is not recommended for volume expansion.
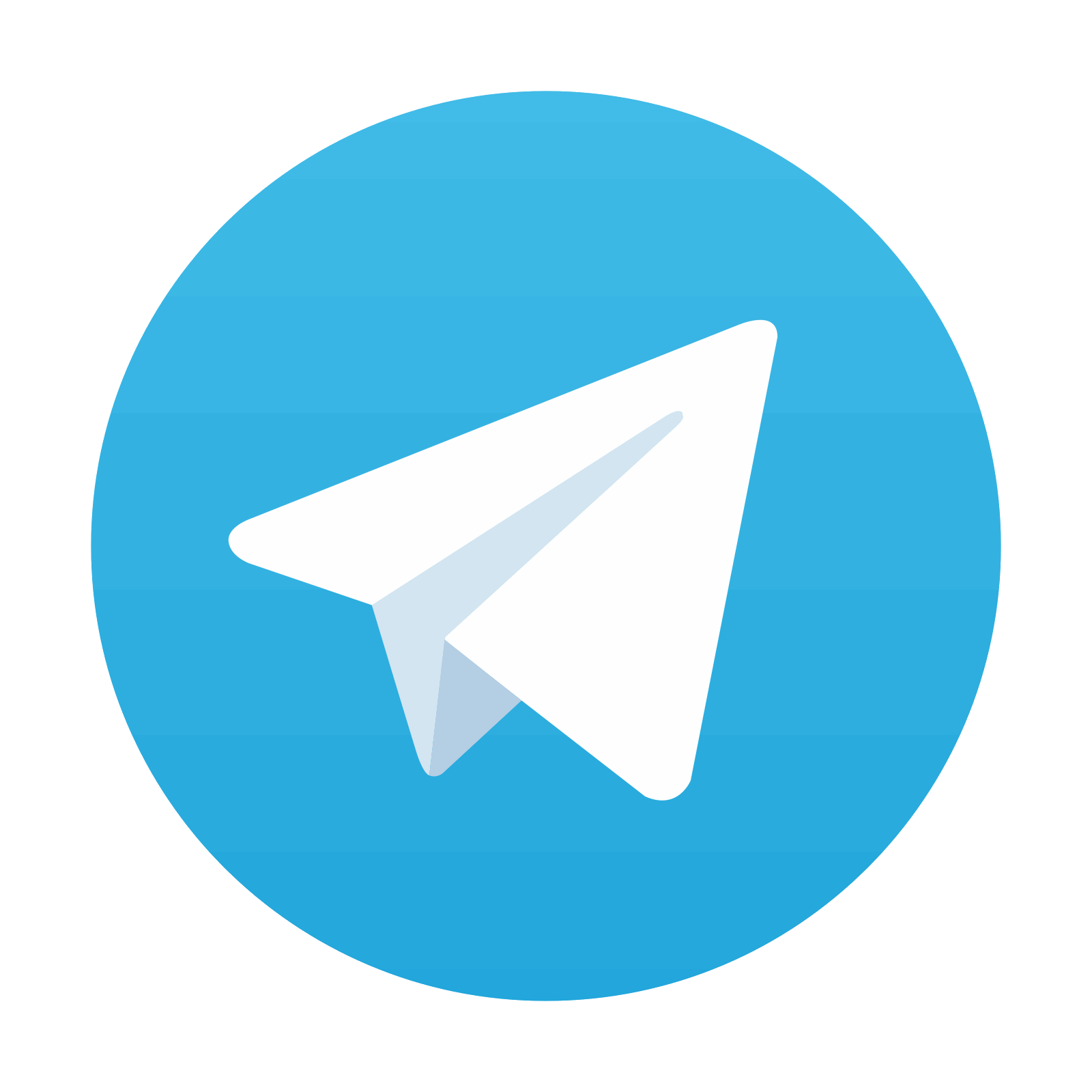
Stay updated, free articles. Join our Telegram channel

Full access? Get Clinical Tree
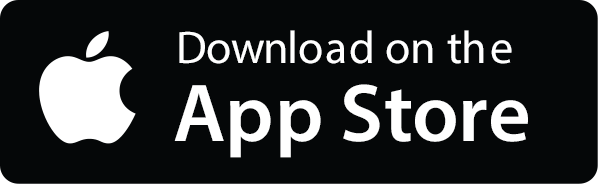
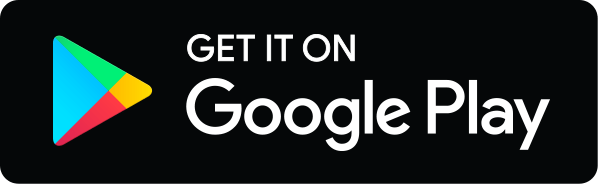
