Introduction
Pediatric patients who have undergone intracranial and other complicated neurosurgical procedures are often best managed in an intensive care unit (ICU). These patients are at risk of acute changes in hemodynamic, respiratory, and neurologic status postoperatively and will therefore need frequent assessment to ensure a stable recovery. Further, these patients need to be in an environment where diagnostic and therapeutic interventions can be initiated immediately upon recognition of a change in status. Specialized neurocritical care teams have been demonstrated to improve patient outcomes for both adult and pediatric populations in higher volume centers.1 The transition from operating room to ICU should begin with clear communication of the patient’s history, intraoperative course (including relevant events such as airway issues, bleeding, and brain edema), and anticipated postoperative course including potential concerning focal neurologic deficits. Formalized sign-outs can be quite useful for this purpose.
All patients will require an immediate, thorough physiologic and neurologic assessment on arrival to the ICU. These thorough assessments should then be repeated frequently, as changes in the neurologic exam will be sensitive indicators of potential postoperative complications.
Respiratory Support
Most often, the primary plan for patients undergoing neurosurgical procedures will involve an extubated, comfortable, and cooperative patient at the conclusion of the surgery. While this is certainly ideal, not all patients who have undergone neurosurgical procedures meet extubation criteria prior to admission to the ICU. Therefore, clinicians should understand some of the unique considerations in the ventilator management of postoperative neurosurgical patients. In this situation, postoperative mechanical ventilation aims to support gas exchange while permitting ongoing neurological assessment. These sometimes competing goals present clinicians with challenges. Certain ventilatory modes including triggered modes (i.e., pressure support) offer a method for providing respiratory support without losing the patient’s intrinsic drive. This may serve as a marker of neurologic function while also minimizing respiratory muscle deconditioning. Although positive end expiratory pressure (PEEP) is commonly applied to most mechanically ventilated patients, it must be used with caution, especially in smaller children. Even small amounts of PEEP can result in hemodynamic problems.2 These problems include impairment of venous return, depressed cardiac output, and ultimately impaired cerebral perfusion.3 In the end, a balance between maintenance of adequate cardiac output and oxygenation must be reached that still allows for adequate cerebral perfusion. PEEP does not seem to increase intracranial pressure (ICP) unless fairly high levels of PEEP are employed. In infants with open fontanelles, there is no association between mean airway and intracranial pressures.
Hemodynamic Support
From a neurocritical care perspective, the primary goal of hemodynamic support is to maintain adequate cerebral perfusion pressure (CPP). Certainly, patients in a critical care setting often have coexisting pathologies that will require a balanced and thoughtful approach to maximizing therapeutic benefit for the patient as a whole. However, this section focuses on hemodynamic goals and support as they relate to the central nervous system (CNS) in critically ill children.
Children of all ages and developmental stages can need neurosurgical procedures that will require a stay in the ICU. In sick neonates, intermittent pressure-passivity of the cerebral circulation is present and can predispose to intracranial hemorrhage. This risk can be exacerbated by significant fluctuations in mean arterial pressure (MAP) and rapid administration of intravenous fluids. However, care must be taken to ensure an adequate CPP. Occasionally this support will require the use of vasopressors and/or inotropes. Even in very low birth weight infants, both dopamine and epinephrine are effective in supporting systemic pressure and restoring cerebral blood flow. Concepts surrounding factors that regulate cerebral perfusion are changing somewhat from classical teaching. It is becoming clearer that the process of cerebral autoregulation is a complicated system that involves much more than simply blood gas tensions and MAP values. Rather, there is synergism and interdependence between these systems as well as a variety of neurogenic mechanisms. Further, it is understood now that cerebral autoregulation does not necessarily maintain a constant perfusion over a range of pressures and that this autoregulation is not controlled solely through the muscles in the pial arterioles. Finally, the autonomic nervous system plays a vital role in modulating cerebral autoregulation and acts as a buffer in surges to perfusion pressure.4 In fact, recent evidence would suggest that at lower levels of MAP, cerebral autoregulation behaves in a much more pressure-passive manner, whereas at higher levels of blood pressure, autoregulatory mechanisms maintain much tighter control over cerebral blood flow.
Implications for the Neurosurgical Patient
Despite the changing concepts of cerebral autoregulation and its implications on clinical care, we must still have basic guidelines and targets for a given patient.5 When increased ICP is present, it is generally agreed upon that critical CPP for preschool children (2–6 years) is approximately 50 mmHg, rising to 55–60 mmHg in older children. In neonates and premature babies, the lower limit of pressure autoregulation is approximately 30–35 mmHg, depending on the adjusted postgestational age of the infant. A common rule of thumb for adequate MAP in these infants is to use the gestational age in weeks as a guideline for the lower limit of MAP. Keep in mind, this is not necessarily supported by clear evidence, so individual patients need to be treated accordingly. There are some recent data that would support this idea of much lower limit of mean arterial pressures to provide adequate cerebral blood flow in infants, at least under sevoflurane anesthesia.6 It must be mentioned that sevoflurane decreases cerebral metabolic requirement for oxygen (CMRO2), so practitioners should extrapolate with caution.
Fluid Management
Meticulous fluid management is crucial in order to optimize the postoperative outcomes of neurosurgical patients. Pediatric patients are predisposed to a variety of postoperative fluid and electrolyte problems. Factors that contribute to this vary with developmental age but include small size, immature renal function, and other comorbidities. These dispositions are further magnified in neurosurgical patients by the disruption of normal homeostatic controls (see Chapter 20).
Overall, more than 10% of all children experience postoperative hyponatremia, and this percentage is likely higher after neurosurgery.7–9 The mechanisms for this hyponatremia are varied and often involve the routine administration of hypotonic solutions to children recovering from surgery. In addition, elevated antidiuretic hormone (ADH) levels can result from a variety of factors including surgical stress, manipulation of structures involved in regulation of fluid and serum sodium status, postoperative pain and nausea, and finally fluid shifts and intravascular hypovolemia. Since sudden, unrecognized decreases in serum sodium can provoke seizures, it is prudent to follow serum electrolyte levels closely throughout the perioperative period. For a detailed discussion of perioperative electrolyte disorders and problems with serum sodium regulation, please refer to Chapter 20.
Concerns and Risks
Nonosmotic secretion of ADH increases the risk of hyponatremia after neurosurgical procedures, despite intraoperative fluids that are high in sodium and isotonic—or slightly hypertonic—to plasma (lactated Ringer’s, 272 mOsm/L; PlasmaLyte, 295 mOsm/L; normal saline, 308 mOsm/L). Elevated levels of ADH can simply be part of an appropriate stress response to surgical stimulation. Alternatively, there are pathologic conditions of inappropriate ADH secretion that must be considered after many neurosurgical procedures, especially those near the sella turcica. When significant hyponatremia occurs, treatment may include hypertonic saline with free water excesses addressed through fluid restriction and administration of diuretics. It is recommended to avoid hypotonic solutions altogether in the perioperative period.
Management of serum glucose can be challenging as well. Small premature infants, with limited reserves of glycogen and limited gluconeogenesis, require continuous infusions of glucose at 5–6 mg/kg/min in order to maintain serum levels. Such infants are at particular risk for hypoglycemia. The stress of critical illness and resulting insulin resistance can produce hyperglycemia, which, in adults, is associated with poor outcomes. Various strategies to mitigate this phenomenon have been devised. Previously, tight glycemic control has been widely recommended in adults, but recent evidence paints a controversial picture surrounding the concepts of tight or standard glucose control. Hyperglycemia in patients with brain injury has been associated with increased rates of infection, longer ICU stays, and poorer neurologic outcomes. However, the effects of hypoglycemia can be deleterious to patients with brain injury as well. In pediatrics, hyperglycemia has been linked to poor outcome, but it remains unclear that tight glycemic control offers significant benefits to children. There is a growing volume of evidence suggesting that tight control may carry undue risk of hypoglycemia and its deleterious effects.10 Given the clear evidence that both hyper- and hypoglycemia can be dangerous to patients who have suffered brain injuries, close monitoring of serum glucose should be part of routine neurologic care in the ICU. However, it also remains unclear how to best manage perturbations in glucose homeostasis in these at-risk patients. A conservative approach that maintains random serum glucose levels below 180 mg/dL may be utilized.
Sedation
Pain control and sedation present unique challenges in the pediatric ICU. Ideally, postoperative neurosurgical patients are comfortable, awake, and cooperative with their care. In pediatrics, some level of sedation is often necessary to ensure a safe recovery. This is true for both patients who have been successfully extubated at the conclusion of the neurosurgical procedure as well as those requiring mechanical ventilation. While the ideal sedation regime would include short-acting or reversible agents that can be withdrawn intermittently to permit neurologic assessment while presenting minimal side effects and development of tolerance, a single agent that fits these criteria and is suitable for children has yet to be developed.
Agents and Their Implications for the Neurosurgical Patient
Propofol
Propofol is a common sedative hypnotic agent that is often used to provide sedation in perioperative and critical care settings. It exerts its effect acting as an agonist at gamma-aminobutyric acid (GABA) receptors. Common side effects of propofol include hypotension, hypoventilation, and apnea. Propofol is metabolized via conjugation in the liver. Small doses of propofol have relatively short clinical effect. This is because propofol is rapidly redistributed from the CNS. Thus, propofol is commonly assumed to be short-acting. The actual half-life is about 1–3 hours. However, continuous infusions of even a few hours will fill the compartments and result in a longer sedation effect. The context-sensitive half-life of propofol infusions is about 40 minutes. It is a potent sedative-hypnotic effect that is extremely useful in adult neurocritical care with limited utility in the pediatric ICU because of its association with the propofol infusion syndrome.11 This is a fatal syndrome of bradycardia, rhabdomyolysis, metabolic acidosis, and multiple organ failure when propofol is used over extended periods in small children. The mechanism of this disastrous and life-threatening complication remains unclear, although its incidence appears related to both the duration of therapy and the cumulative dose. These difficulties are much less common in adults. If propofol is utilized in pediatric patients, continuous infusions of limited duration are recommended.12
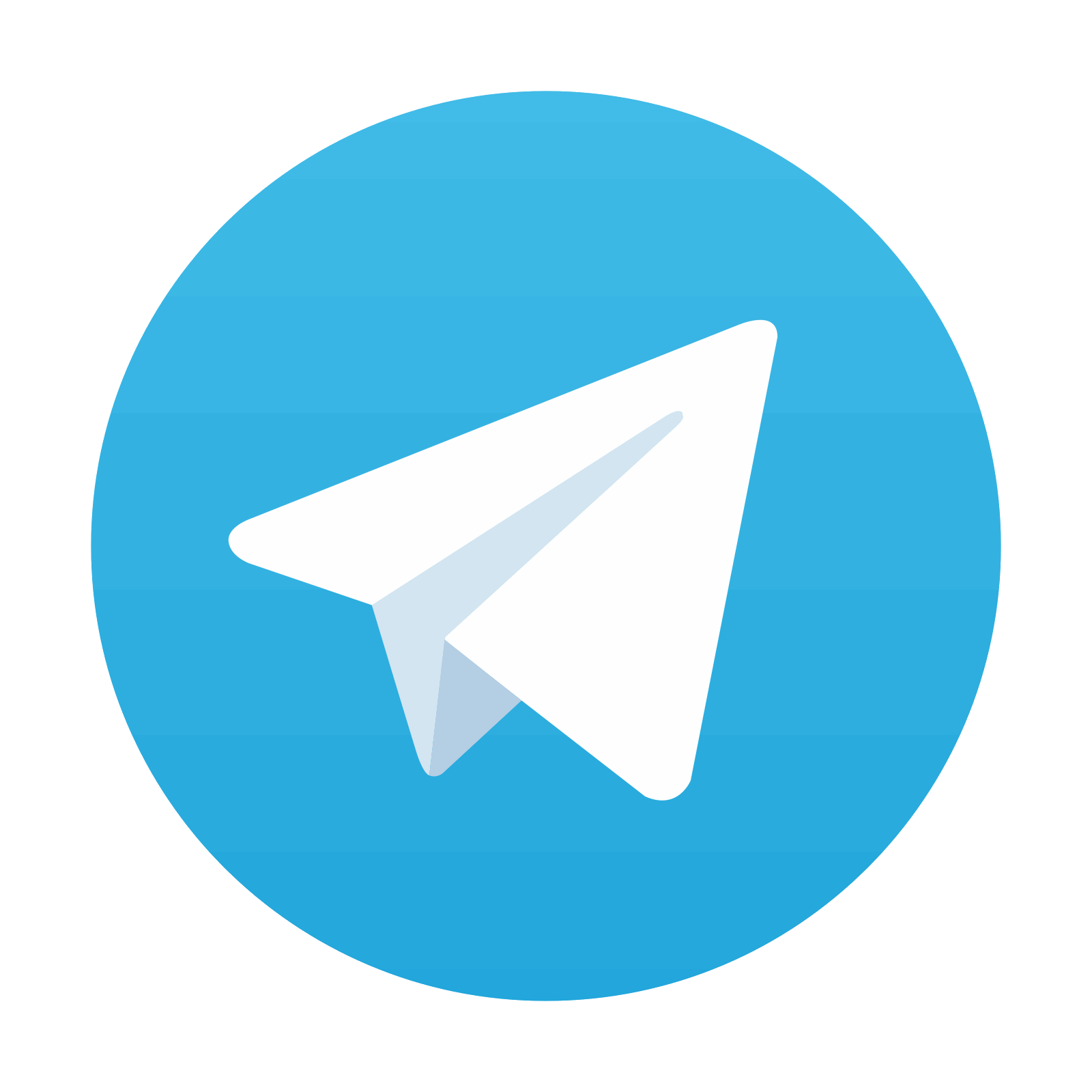
Stay updated, free articles. Join our Telegram channel

Full access? Get Clinical Tree
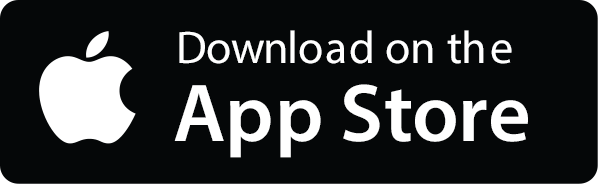
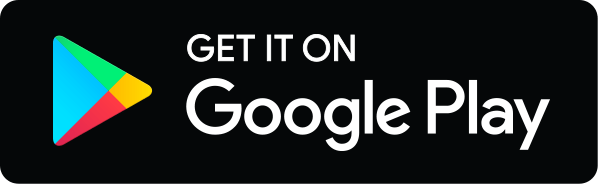
