Introduction
The overall goal of perioperative care is to prepare the patient for the right surgery, at the right time, under the right circumstances of physiology, all with the best expected outcomes for the child’s underlying condition. The prescription of intravenous fluids for children unable to tolerate oral therapy is fundamental in this perioperative period. What we want to know is how much and what type of fluid should we give.
The main aims of this chapter are to cover the essentials of perioperative fluid therapy, the pitfalls in measuring and following electrolyte levels, and the approach to postoperative dysnatremia. We want a patient who is, first, hemodynamically stable, second, receiving intravenous fluids that limits or avoids electrolyte abnormalities, and, third, adequately supplied with glucose for metabolic homeostasis. The first aim requires careful maintenance of intravascular volume. Preoperative fluid restriction may lead to blood pressure instability and even cardiovascular collapse intraoperatively, with problems carrying over into the postoperative phase. Preoperative hyperhydration may lead to abnormalities in salt balance that also carry over into the postoperative period. It is therefore important to know what has happened preoperatively and intraoperatively: the type and volume of fluid given, the estimated blood loss as a proportion of blood volume, as well as fluid inputs and outputs.
Preoperative Intravenous Normal Saline and the Threat to Homeostasis
In common hospital preoperative care, normal saline (NS) is the preferred intravenous fluid for neurosurgery cases since its osmolarity (308 mOsm/L) should minimize the occurrence of hyponatremia (serum sodium concentration [Na+] < 135 mmol/L). In children, we calculate the maintenance fluid required for a child scaled to their weight using the classic Holliday and Segar1 formula for daily fluids: 100 mL/kg for the first 10 kg in body weight; add 50 mL/kg to the 1,000 mL for the next 10 kg in body weight (i.e., weight 10 to 20 kg); add 20 mL/kg to the 1,500 mL for weight above 20 kg.
The above calculations should be placed in the context of recommended salt (NaCl) intake per day in healthy children. Normal 4- to 8-year-olds require 1.2 g/day (20 mmol/day), but observational studies show that the actual upper limit of intake is ~2.6 g/day (44 mmol/day). Now consider a 20 kg, 6-year-old boy who is placed on 1.5 times fluid maintenance therapy using NS, during the day before surgery, so as to ensure that intravascular volume is optimized for a cerebrovascular procedure. The NaCl intake from NS over 24 hours in this child is 20.2 g/day. Hence, we have taken a child with healthy kidneys and neuroendocrine physiology from a baseline NaCl intake of 2.6 g/day and increased it to 20.2 g/day—an increase from 1 teaspoon to 9 teaspoons of salt per day!
Such an abrupt intervention with preoperative intravenous fluids does result in an expanded arterial volume, which is what was wanted in preparation for surgery, but a foundation has been laid for a subsequent threat to homeostasis. In the proximal tubule, the luminal Na+ transport system and the basolateral Na+ pump are removed from membranes of individual proximal tubular cells, so that there is reduced ability to reabsorb filtered Na+ ions. Of course, the body is aiming to excrete the excess NaCl load. If, however, there is any decrease in the rate of infusion of NS, then the patient will continue to excrete Na+ because of so-called secondary renal wasting. The potential risk is illustrated by studies in the 1950s to 1990s in healthy adults that looked at transitioning from low to high NaCl intake (0.6 g/day to 20.5 g/day), and the reverse. A change to a high intake leads to a new steady state with high antidiuretic hormone (ADH), low renin, and low aldosterone, which lag behind the acute change in intake by 24–48 hours. When this new steady state of homeostasis is achieved, excess NaCl intake is balanced by increased renal wasting such that serum [Na+] remains stable. Hence, the risk for our patient is that of excess Na+ loss and hyponatremia in the 48 hours after any abrupt decrease in NaCl intake to maintenance levels.
The illustration shows an extreme example with use of 1.5 times maintenance in a preoperative child. When we care for perioperative neurosurgical patients we also need to add to this discussion the intravenous fluids used in the operating room. How long did the procedure take, and how much maintenance fluid was given? It is not unusual for long procedures to necessitate maintenance fluid up to 10 mL/kg/hr. Were any saline boluses given? When more than 60 mL/kg has been used, there is the risk of hyperchloremic metabolic acidosis, which may not be appreciated unless serum chloride concentration ([Cl–]) was measured.
Variations in Sodium and Chloride Measurements
The [Na+] in serum or plasma is quantified by using ion-specific electrode (ISE) technology to measure the activity of Na+ ions in the aqueous (water) phase of plasma. There are two methods that are used for providing clinicians with [Na+] and [Cl−] values: indirect and direct ISE. Indirect ISE technology is used in central laboratory analyzers, and involves presentation of a prediluted serum or plasma sample to the measuring electrode. The measurement assumes that the water content of plasma is constant at 93%. If lipids and/or protein are raised, then the nonwater fraction is >7%, and the diluted sample presented to the ISE contains fewer Na+ ions than would be the case if the sample had a normal proportion of water (93%), i.e., pseudohyponatremia. By similar thinking, if lipid and/or protein are reduced (or diluted by hyperhydration), then the nonwater fraction is <7% and the water fraction is >93%, resulting in falsely high [Na+], i.e., pseudohypernatremia. Direct ISE technology is used in blood gas and other point-of-care analyzers, and requires the presentation of an undiluted sample of whole blood to the measuring electrode. Direct ISE measurements are not affected by changes in lipid and protein concentration because there is no predilution of the sample. What is being reported is the actual [Na+]. Having identified the methods for measuring [Na+] in a particular patient—most likely using indirect ICE technology in the pre- and postoperative periods, and direct ICE technology intraoperatively—the clinician should consider two issues: (1) the use of population-based reference (normal) intervals in detecting abnormal changes and (2) the significance of changes in consecutive results from an individual.
First, the values of the reference interval (RI) are generated and established by your hospital’s credentialed laboratory. Sometimes the RI data are stratified according to age and sex. In order to assess how clinicians should interpret changes in measured values, i.e., using the population-based RI versus using the serial values, we need to consider biological variation. The within-person or individual coefficient of variation (CVI) and the between-person coefficient of variation (CVG) for [Na+] are 1% and 1.8%, respectively, and for [Cl−] 1.6% and 2.8%, respectively.2 The index of individuality is calculated as the ratio of CVI/CVG. A high index (≥1.0, or >1.4 for maximum sensitivity) suggests that the population-based RI is appropriate when interpreting an individual’s laboratory analyte value. In contrast, a low index (≤1.0, or ≤0.60 for maximum sensitivity) indicates that under normal circumstances individual results stay within a narrow range compared with the population-based RI. Hence, a low index would suggest that in an individual there is utility in following serial changes in analyte values, and population-based RI would be of limited usefulness.3, 4 Both [Na+] and [Cl−] measurements have a low index of individuality—0.55 and 0.57, respectively.
In view of the above, the second issue of clinical significance of changes in consecutive results from an individual must be considered. The critical significant changes between two results is called the reference change value (RCV) and is determined in healthy individuals by the following formula:

In this formula CVA is the laboratory analytical coefficient of variation, and for both [Na+] and [Cl−] it is 0.8%.2 The value of the constant k is 1.65 for a 1-tailed test and a probability risk α of 95%; k = 1.96 for a 2-tailed test. For example, this formula applied to an analyte result in real time at, say, [Na+] 140 mmol/L gives RCV = 3.5%. This translates into an RCV of 135.3–144.7 mmol/L: a difference in two measurements of [Na+], when starting at a concentration of 140 mmol/L, as large as the upper boundary minus the lower boundary of the 95% confidence interval (CI) could occur at random. If the starting point was 135 mmol/L the 95% CI is 130.3–139.7 mmol/L. When we consider a baseline [Cl−] 100 mmol/L the RCV is 5% and the 95% CI is 95–105 mmol/L. In clinical practice we are more often concerned with the 1-tailed test; e.g., have we had a significant rise or improvement in [Na+] from a hyponatremic value of 120 mmol/L since a particular intervention? Based on the above data, with k = 1.65, the increase in value in the correction of hyponatremia would have to be to ≥123 mmol/L to be certain of a real change.
Postoperative Dysnatremia
Disorders of Na+ and water homeostasis are common in postoperative neurosurgical patients. Four main Na+– and water-handling problems occur, including inappropriate intravenous fluid administration, euvolemic state of ADH excess (i.e., syndrome of ADH excess, SIADH), cerebral salt wasting (CSW), and diabetes insipidus (DI). However, before embarking on detailed analyses of Na+ and water balance, when an abnormal serum [Na+] is received from the laboratory, we first need to consider the issues discussed in the first part of this chapter, i.e., the physiological context or priming that has occurred in the pre- and intraoperative periods, and the validity of the measurements themselves. For example, has there been significant use of perioperative radiologic nonionic hyperosmolar contrast medium, which will cause pseudohyponatremia if the measurements come from indirect ICE technology (often reported as “extracellular fluid” [Na+] rather than “whole blood” [Na+])? Then, as part of the evaluation, there should be some assessment of cerebrospinal fluid losses (CSF)—for example via CSF drainage.
In the postoperative patient there is a significant risk of hospital-acquired hyponatremia, which approaches 30%.5 In the pediatric neurosurgical literature there are three recent reports describing the significant scale and impact of this problem.6–8 In this setting postoperative pain, stress, nausea, vomiting, narcotics, and volume depletion all have the potential to stimulate vasopressin production and induce a state of euvolemic hyponatremia not dissimilar to SIADH—in fact ADH secretion may well be an appropriate survival response. To date, there have been three prospective trials of intravenous fluids in postoperative patients (not specifically neurosurgical patients): two studies in the pediatric intensive care unit,9, 10 and one study in the postoperative ward.11 Unfortunately, the endpoints in these studies differed in respect to what and when: either an absolute change in serum [Na+],9 or proportion of cases with hyponatremia defined as below 135 mmol/L9 or below 130 mmol/L;11 and measurements at 8,11 12,11 or 24 hours.9–11 (There has also been a recent randomized clinical trial in nonsurgical pediatric patients,12 and since the findings do not differ from the results in postoperative patients we do not focus on these data.) The general message from the postoperative studies is that isotonic fluids (i.e., NS) prevent postoperative falls in serum [Na+] and that hypotonic fluids result in falls in [Na+]. In none of the postoperative studies was fluid overload or significant hypernatrema a complication.9–11 We do not know the current epidemiology of perioperative pediatric neurosurgical intravenous fluid management, but a survey in 2006 of practice in general pediatric surgeons and anesthesiologists using infant and child clinical scenarios showed that significant numbers of clinicians were using hypotonic fluids or fluid restriction.13 The 2007 Association of Paediatric Anaesthetists of Great Britain and Ireland guideline on perioperative fluid management in children14 identified the following two gaps in knowledge for postoperative intravenous fluid management:
1. “Consensus was not agreed on what the ideal fluid for postoperative maintenance is. Many felt that Ringer lactate/Hartmann’s solution with added dextrose was the most appropriate, but currently this formulation does not exist in the UK. 0.9% sodium chloride with 5% dextrose is the only available isotonic fluid containing dextrose within the UK at present.”
2. “Consensus was not agreed on the maintenance fluid rate in the postoperative period. Some would use the full rate as calculated using Holliday and Segar’s formula, while others would restrict to 60–70% of full maintenance and additional boluses of isotonic fluid given as required.”
Modeling the Effect of Too Much Water versus Too Much Salt
The debate about volume and type of intravenous fluids arises because it is not clear what is maintenance requirement for the postoperative child. We have all seen the edematous child who has received too much free water, or who has received too much Na+? What is worse?
Figure 20.1 illustrates the arguments for and against volume and concentration by modeling Na+ and water handling.15, 16 Figure 20.1A shows responses in extracellular (Ve) and intracellular (Vi) volumes in a closed system with two compartments separated by a membrane that permits the movement of water, but is impermeable to Na+ and potassium (K+), which are the sole Ve and Vi cations. If the Vi component of the model functions as a perfect osmometer, then the sloping lines represent isopleths, lines of constant overall volume (Ve + Vi). The numbers have been normalized to body water, with loss or gain in a percentage for each compartment, relative to the origin (point O), which is steady-state euvolemia. The two vectors represent an accumulated positive balance in the system, either 20 mL/kg of water (vector OW) or 20 mL/kg of NS (vector OS). In this model, which is applicable between 10 and 30 kg body weight, the effect of gaining 20 mL/kg of saline is an 8% increase in Ve, without any change in Vi or serum osmolarity. The effect of gaining 20 mL/kg of water is an expansion in both Ve and Vi, each by over 3% with an associated fall in serum osmolarity. (The vector SW represents a desalination of 1.5 mmol/kg, and any movement along this same axis above horizontal can be considered as net loss of Na+ from a particular initial condition.)
Figure 20.1. (A) Model of fluid shifts due to 20 mL/kg intravenous water or NS (N, saline). (B) Model of fluid shifts due to different tonicities of NS (0.18%, N/5; 0.45%, N/2; and 0.9%, N) and different volumes (i.e., full or two-thirds maintenance).
The homeostatic challenges are different for the two conditions OW and OS. In the case of gain in NS, the theoretical system must deal with the acute NaCl load, the change in interstitial compliance as Ve increases, and resulting edema. These issues are not trivial. For example, in healthy adults, in the supine position, it takes 2 days to regulate the amount of salt and water provided by an acute saline infusion.17, 18 Experimentally, generalized edema will accelerate after ~15% expansion in Ve, although this response will be different in different tissues as well as being influenced by plasma colloid osmotic pressure.19–21 In the case of gain in water, the main difference to gain in NS is that the system must defend changes in Vi induced by the hypoosmolality. Is this threat real for the range of values typically seen in postoperative neurosurgical children on maintenance intravenous fluids? In other words, which tissues function as perfect osmometers, like the model?
Experimental studies show that skeletal muscle, which contains the bulk of an organism’s water and K+, is important as a buffer during acute hyponatremia since it serves as a near-perfect osmometer.22, 23 In contrast, within the brain, increases in water content are less than expected, but there are regional differences.24, 25 For example, during acute hyponatremia ([Na+] reduced from 138 ± 1 to 123 ± 2 mmol/L) the water content of the whole brain and white matter increases, but it is less than expected for perfect osmotic behavior, whereas in the thalamus, water content increases as expected for a perfect osmometer.23 These data provide the experimental context for interpreting the significance of change in electrolytes summarized in the previous section. It is true that symptomatic hyponatremia with encephalopathy in previously well children has the potential for high neurologic morbidity.25, 26 How likely is this complication in the first 24 hours after an operation and admission to the intensive care unit?
Figure 20.1B uses similar principles to those in Figure 20.1A but, in addition, the model takes account of mass balance for Na+ and K+, where the input comes from intravenous fluid and the output in water and electrolytes is considered as solely via urine.27 The three prospective studies of postoperative and intensive care children provide information about urine tonicity and volume.9–11 Maintenance fluids were calculated using the Holliday and Segar equation1 in all three studies. Urine tonicity across the studies is almost fixed: in those receiving NS at full maintenance urine tonicity is ~200 mOsm/L; in those receiving any other prescription urine tonicity is lower, ~160 mOsm/L. Only one study reports urine output,11 and it appears that irrespective of fluid prescribed mean output over 12 to 24 was ~1.2 mL/kg/hr. These data have been used to generate the four vectors for the 12-hour cumulative fluid and tonicity balance in Figure 20.1B. Starting from the origin (point O), the greatest increase in Ve is seen with full maintenance NS. The likelihood of increased Vi and hypoosmolarity is in the following order: NS, two-thirds restricted half-NS (67% N/2), full maintenance half-NS (100% N/2), and two-thirds one-fifth-NS (67% N/5). The severe derangement occurring with 67% N/5 is nearly three times the theoretical expansion of Ve and hypoosmolality occurring during the other prescriptions. The problem of hypernatremia is not seen in the model, but more complexity in the volume relationships can be added with other variables common in postoperative neurosurgical children, e.g., the sequestration of extracellular water within injured tissues necessitating NS replacement, neuroendocrine stress physiology, and consideration of gains, losses, and urinary desalination.28–31
Postoperative Renal Na+– and Water Handling
The three main renal Na+– and water-handling problems that occur in such children are euvolemic states of ADH excess (i.e., SIADH), CSW, and DI. Therefore, regular monitoring of intravascular volume, urine output and tonicity, and serum electrolytes is needed during the period of administering intravenous fluids.
Syndrome of Inappropriate ADH Secretion
The risk of postoperative hyponatremia is a major concern since it may go unrecognized until the onset of a seizure.32 If the cause is perioperative SIADH, and not inadequate NaCl prescription, it has occurred because of free water retention followed at the same time as natriuresis that maintains fluid balance at the expense of serum osmolarity. Because of this risk, many clinicians avoid hypotonic solutions altogether in the perioperative period. It should be noted that Ringer lactate Na+ content (130 mmol/L) might result in a fall in serum [Na+]. This fluid is often used intraoperatively as it is a balanced solution with a physiologic amount of base, calcium, and K+, and will limit the hyperchloremic acidosis that occurs with large volumes of Na.
The treatment of SIADH is to reduce free water excess by fluid restriction and diuretics. If a hyponatremic seizure occurs, then 3% hypertonic saline (3%HTS) should be used to correct serum [Na+]; the level to be targeted is that at which the seizure comes under control, often ~130 mmol/L. Taking 0.6 L/kg body weight as the apparent volume of distribution for Na+, one should anticipate an immediate increase of 3 to 5 mmol/L in serum sodium concentration with a rapid intravenous bolus of 4 to 6 mL/kg body weight of 3%HTS.33
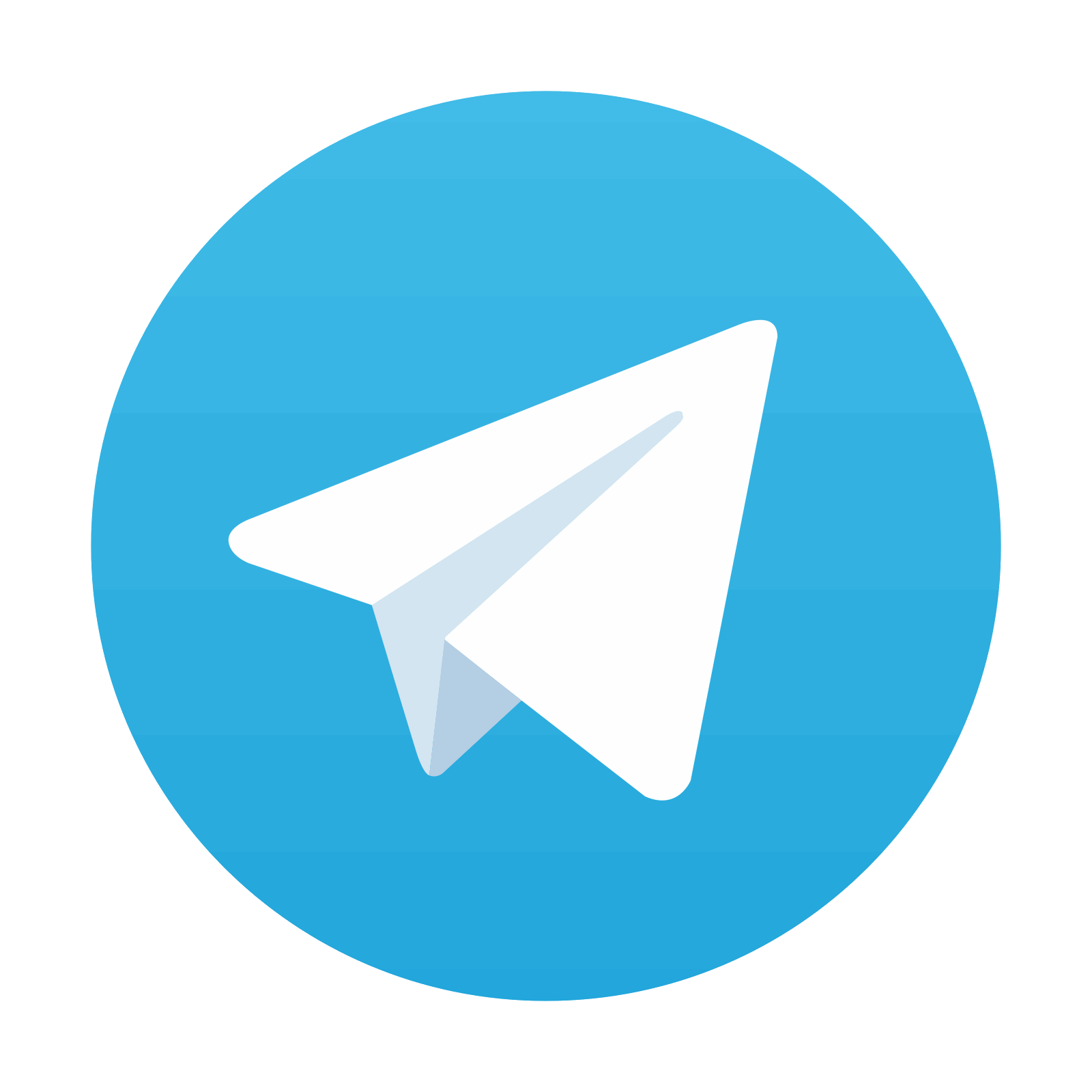
Stay updated, free articles. Join our Telegram channel

Full access? Get Clinical Tree
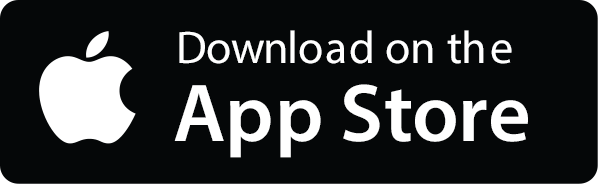
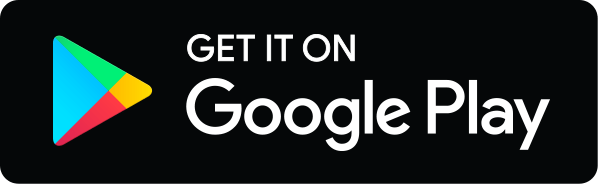
