Introduction
The safe conduct of anesthesia during the neonatal period and infancy is associated with more complications than during later periods of childhood. Many neonates require immediate surgery during the period in which their circulation and respiratory systems are transitioning from fetal life to postnatal life. Blood pressure is generally at its lowest nadir at this time, and the depressant effects of general anesthesia may further decrease blood pressure to the point of affecting cerebral perfusion.1 Major congenital defects occur in approximately 3% of all births, with cardiac defects accounting for almost one-half of all serious congenital defects.2 These anomalies are the leading cause of infant mortality in the United States, with the vast majority of infants born with a serious congenital anomaly requiring surgery in the first year of life. Mortality and morbidity after anesthesia are approximately tenfold greater in neonates compared to older infants, with the mortality after intraoperative cardiac arrest in neonates being 92%.3 Several studies across many developed countries reveal that approximately 12% of all children will have a general anesthetic before the age of 3, which translates to millions of very young children receiving general anesthesia every year.4, 5 In addition to being concerned about the immediate neurophysiologic effects of general anesthesia on the well-being of our youngest patients, pediatric anesthesiologists are also concerned about the possible neurotoxic effects of general anesthesia on these fragile patients. Laboratory evidence of anesthetic and sedative drugs causing immediate neurotoxic damage and later neurocognitive deficits is incontrovertible. The evidence for direct neurotoxic damage in young humans is much less certain.
This chapter reviews the expanding literature about neurotoxicity in preclinical in vitro and in vivo studies as well as provides an update on the clinical data available.
Laboratory Findings
Developmental neurotoxicity had been demonstrated in laboratory models after exposure to the majority of anesthetic and sedative drugs that are currently used in pediatric anesthetic practices.6–11 Other types of medications that cause similar laboratory effects include anticonvulsant medications and ethyl alcohol.12 The implicated general anesthetics include N-methyl-D-aspartate (NMDA) antagonists such as ketamine and nitrous oxide and gamma-aminobutyric acid (GABA) agonists such as the volatile anesthetics, propofol, barbiturates, and benzodiazepines. Susceptibility to these anesthetics in laboratory animals begins in the late fetal life through early development, which in most species is the time of maximal synaptogenesis or the “brain growth spurt.” Three distinct neurodevelopmental histologic abnormalities have been shown in juvenile animals: an abnormal degree of neuroapoptosis, abnormal neurogenesis, and altered dendritic formation. Brain development in mammals involves neurogenesis and synaptogenesis. Neurons that do not make synapses are considered redundant and undergo a programmed cell death known as neuroapoptosis. However, general anesthetics administered to young animals that are undergoing brain growth spurts can lead to a fiftyfold increase in neuroapoptosis with a resultant overall loss of neurons compared with young animals that were not exposed to general anesthesia.6, 7 The time frame for rapid synaptogenesis varies from species to species, with young rats demonstrating maximal vulnerability from postnatal days 1 through 14 with a peak at postnatal day 7. In rhesus monkeys the period appears to be the later third of gestation to the first postnatal week. In humans, the brain growth spurt is believed to be during the last trimester of gestation to the first 3 years of life.13 Exposure to noxious stimuli and stressors such as maternal deprivation, hypoxia, hypoglycemia, and ischemia during these time frames can also induce similar neurodegenerative changes in young animals.
Characterization of Anesthesia-Induced Developmental Neurotoxicity (AIDN)
Pathological Apoptosis
An increase as well as acceleration in neuroapoptosis is the most significant finding after general anesthesia exposure in young animals. Although apoptosis is a normal process by which the body prunes itself of abnormal or redundant neurons, in young animals the apoptotic pathway can be activated by prolonged or repeated exposure to general anesthetics. Apoptosis differs from other types of cell death in that it is almost always executed by caspase enzymes, which are cysteine-dependent aspartate proteases that either initiate the apoptotic process (caspases 2, 8, 9, and 10) or affect the process (caspases 3 and 7). The first pathway usually activated is the intrinsic pathway, which is mitochondrially activated. When the mitochondria are under stress, they can release proapoptotic proteins such as cytochrome C, procaspases, smac/diablo, bin G, adenylate kinase-2, and apoptosis inducing factor (AIF). Stress causes the outer mitochondrial membrane to become porous, and these proapoptotic proteins are released from the space between the inner and outer mitochondrial layers. AIF is unusual in that it can induce cell death without activating the caspase enzyme system.14 A family of proteins known as the Bcl-2 family includes proteins that act as anti- or proapoptotic regulators within the cytosol. Cellular stress leads to an increase in the BAX proteins (Bcl-2 proapoptotic), which causes an increase in mitochondrial outer wall permeability and release of cytochrome C and other proapoptotic proteins, which activates caspase 9. Volatile anesthetic exposure can cause impaired mitochondrial function and activation of the intrinsic apoptotic pathway. Mitigators that restore mitochondrial integrity such as reactive oxygen species scavengers can ameliorate this response.
The extrinsic pathway involves the activation of the Fas receptor on the cell wall. Tumor necrosis factor (TNF), when it binds the death receptor protein, forms a ligand known as the TNF-related apoptosis inducing ligand or TRAIL. Both these receptors can activate procaspase 8 and initiate the caspase cascade.
Abnormal Neurogenesis
In very young rats, volatile agents and propofol have been shown to cause a loss of neural stem cells with a resultant decrease in neurogenesis. In contrast, isoflurane exposure causes a brief increase in abnormal neurogenesis in the adult rat followed by a loss of these abnormal cells.15 Isoflurane exposure is also associated with impaired glial cell neurogenesis.16 The inflammatory response elicited by general surgical trauma is also implicated in causing a decrease in neurogenesis in young animals. The evidence points to a twofold negative effect of general anesthesia exposure in young animals, a decrease in neural stem cells, and a decrease in the remaining stem cells to proliferate and mature into neurons.17, 18
Altered Dendritic Development
Maximal anesthetic neurotoxicity generally occurs developmentally around the time of maximal synaptogenesis. Very young rodents from postnatal day 5 to day 14 generally exhibit abnormal neuroapoptosis after anesthetic exposure or a decrease in synapse formation. In slightly older animals, abnormal dendritic spines are formed. A dendritic spine generally receives input from a single neuron and is necessary for synaptogenesis. Rat species are generally susceptible to forming abnormal and increased number of dendritic spines at a postnatal age of 15–20 days.19, 20 Implicated anesthetics include propofol, midazolam, volatile agents, and ketamine. The impact of both a decrease for younger rats and an increase in slightly older rats of dendritic spine formation is unknown. New learning tasks in rats that are not exposed to anesthetics are associated with new dendritic spine formation. The altered dendritic spine formation after anesthetic exposure in the different developmental age groups in rats highlights how anesthetic neurotoxicity differs with age.
Aberrant Glial Development
In addition to neuronal apoptosis, neural glial cells are negatively affected by anesthetic exposure at a young age. Glial cells are crucial developmentally because by creating the scaffolding for neurons, they guide the migration of neurons from progenitor layers of neuronal substrate to necessary locations to make appropriate synapses. Isoflurane has been found to decrease the release of brain-derived neurotrophic factor (BDNF) by astrocytes.21 This factor encourages developing neurons to develop axons to form synapses. Astrocytes are impaired during neural development by exposure to isoflurane. In rhesus monkeys and fetuses, isoflurane is also associated with an increased level of apoptosis.10, 22
Anesthetic Effects on Spinal Cord
The effects of general anesthesia exposure have been noted to cause neurotoxicity in the spinal cord of young animals. Exposure to isoflurane and nitrous oxide causes an increase in neuroapoptosis of the spinal cord especially in the ventral horn in very young rat pups.23 However, when these animals were allowed to mature there were no motor functional abnormalities detected. Intrathecal ketamine injections cause an increase in neuroapoptosis and microglial activation in the spinal cords of postnatal day 3 rats and later motor functional disabilities.24 In contrast, intrathecal morphine and bupivacaine in very young rats given in analgesic and anesthetic doses did not lead to an increased level of spinal cord neuroapoptosis.25
Neuroinflammation
Inflammatory conditions in premature human infants are known to lead to poor long-term neurocognitive outcomes. Premature infants that are born small for gestational age, which can be a proxy for intrauterine inflammation, are at an even higher risk for developmental disabilities than age-matched cohorts who were born at a normal weight for gestational age.26 In adults, activation of the neuroinflammatory cascades such as seen with surgical trauma has been implicated in postoperative cognitive dysfunction. The effects of general anesthetics on activating or deactivating these cascades are mixed. Sevoflurane had been shown to increase neuroinflammatory markers in young but not adult mice.27 Other studies in young rats have shown that ketamine can ameliorate the neuroapoptotic effects of painful stimuli.28
Neurocognitive Function
Most of the studies examining the long-term decrements in neurocognitive function are based on rodent models. In this species, fetal, neonatal, and infant exposure is associated with both long- and short-term memory loss, as evidenced by poor performance on the Morris water navigation test and radial arm test. After exposure to ketamine and sevoflurane in utero or in early infancy, rhesus monkeys show increased anxiety to the human intruder test and decreased motivation in their performance on the operant test battery.29
Relevant Anesthetic Durations and Concentrations
It is clear that anesthetic duration and concentration matter in terms of neurotoxicity for young mammals. Although both are important metrics, the anesthetic duration appears to have a higher impact than anesthetic concentration. Most studies that have shown an effect of anesthetic exposure involved an exposure of at least 4 hours, and in some studies involving monkeys and ketamine, an exposure of 24 hours was necessary before a neurotoxic effect was noted on psychometric testing.30 There are some studies that have shown that exposure to 0.25 to 0.5% minimum alveolar concentration (MAC) for 6 hours or greater is associated with an uptick of neuroapoptosis as demonstrated by an increase in caspase 3 histologic staining on brain slides.31 The data are mixed as to whether the volatile anesthetics are equally neurotoxic.20, 32 Some studies suggest that desflurane may be more neurotoxic than sevoflurane or halothane, but other studies in young mice demonstrate an equivalent level of apoptosis for equivalent MAC levels of the volatile anesthetics. There is a suggestion that an anesthetic mixture of a volatile anesthetic with nitrous oxide is more neurotoxic than a volatile anesthetic alone. It is unclear whether this is due to an additive or synergistic effect.
In general opioids do not increase neuroapoptosis, but under some experimental conditions, repeated morphine administration over 7 days is associated with increased apoptosis in the sensory cortex and amygdala of neonatal rats. However, a single dose of morphine given to postnatal day 7 rat pups did not increase neuroapoptosis.33 Furthermore, daily administration of morphine for 9 consecutive days did not alter dendritic morphology. These areas of the brain are different from those affected by volatile and intravenous anesthetics, which preferentially affect the learning and memory areas (hippocampus) of developing brains.
Alleviation of AIDN
Apoptosis serves a very important function throughout the life span of mammals. During development it is a method of pruning redundant, abnormal, and useless cells of the organism in order to facilitate differentiation within the organ systems. Later it is chiefly a method of ridding cells that are abnormal in order to prevent tumors and other illnesses from occurring. As such it is logical that there are many different molecular pathways for apoptosis to occur and thus many different potential ways to block these pathways. Medications such as lithium, melatonin, estradiol, carnitine, erythropoietin, and dexmedetomidine have been shown to block pathways that lead to apoptosis. Dexmedetomidine, an adjunctive anesthetic agent, is particularly promising as an agent that may be partially neuroprotective during anesthetic exposure. It has been shown in rat pups to decrease isoflurane-induced neuroapoptosis and behavioral deficits.34 However, extremely high doses of this drug will induce neuroapoptosis in very young rats. Its protective mechanism is unknown, but it is known to induce cell survival signaling pathways at clinically relevant doses. Although the animal data are concerning, there is some evidence that an enhanced and stimulating environment is more important to normal rat development than volatile anesthetic exposure.35
NMDA Receptor Antagonists
Ketamine
Neuroapoptosis induced by ketamine has been demonstrated in mice, rats, and rhesus monkeys, with peak effect occurring during the period of peak synaptogenesis. In monkeys this effect occurs in the last trimester of fetal development and the first 6 days postnatally.30 By 35 days postnatally in monkeys, there is no neuroapoptotic effect found even in monkeys that were exposed to 24-hour infusions of ketamine. The long-term developmental effects of early exposure to ketamine include decreased motivation, color discrimination, anxiety, and short-term memory when the animals are tested at age 2. The doses used to sedate the monkeys were approximately 10 times the dose that is conventionally used to sedate humans and resulted in serum levels 10 times higher. However, the dose of 25–50 mg/kg/hr was the minimal dose required to sedate the monkeys and prevent them from biting their handlers. These differences in dosing requirements between humans and nonhuman primates highlight the difficulties in extrapolating results between the two closely related species. Similar findings have been demonstrated in rats. Repetitive doses of ketamine at 20–50 mg/kg given every 90 minutes for 7 or more doses will result in histologic evidence of increased neuroapoptosis.36, 37 Repetitive doses of 4 or less did not increase the level of neuroapoptosis. Mice appear to be more sensitive to the neurotoxic effects of ketamine, with a single dose of 10 mg/kg resulting in increased neuroapoptosis and later learning disabilities in P7 mice.38
The neurotoxic effects of ketamine are amplified when the drug is combined with a GABAergic agonist such as thiopental or propofol. Mice pups at postnatal day 10 demonstrated neurotoxicity and later learning deficits when exposed to ketamine at 25 mg/kg and either thiopental 5 mg/kg or propofol 5 mg/kg.39 There were no deficits in the group of mice exposed to a single dose of ketamine.
Ketamine appears to have salutary neuroprotective effects in some situations. Painful inflammatory stimulation in P7 rats such as injection of Freund’s adjuvant into the hind paw will induce an uptick in neuroapoptosis seen in the cortical and subcortical regions of the brain, which is ameliorated by concurrent low-dose ketamine sedation.28
Nitrous Oxide
Nitrous oxide exposure has been demonstrated to cause an increase in neuroapoptosis in neonatal mice but not rats.40 This degree of neuroapoptosis is greatly increased when nitrous oxide is combined with isoflurane in both neonatal rats and mice. However, like ketamine, it does have some neuroprotective properties. Juvenile animals show less neuronal cell death after a hypoxic ischemic injury if the injury occurs while the animal is being exposed to nitrous oxide.41 Adult humans have less chronic pain after major surgery when nitrous oxide is used as part of a general anesthetic.42
GABAergic Agonists
Anesthetic agents that are GABA agonist agents have been extensively studied in juvenile animals and all have been implicated in causing an increased level of neuroapoptosis and later neurocognitive deficits. These drugs include barbiturates, benzodiazepines, propofol, and the volatile anesthetics. The neurotoxic injury induced by GABAergic agents generally occurs in different brain locations than the NMDA antagonists, which may explain that in some studies combinations of these two classifications of general anesthetics lead to worse outcomes. One of the theories of the etiology of neurotoxicity of GABAergic agonists is that the GABA receptor in young animals is excitatory rather than inhibitory.43 Animal studies of young rat pups given sevoflurane will show some epileptiform activity.43
Benzodiazepines
High doses of benzodiazepines have induced neuroapoptosis in neonatal mice and rats. A single dose of 5 mg/kg of diazepam will cause neuroapoptosis in young mice but not young rats.44 This dose was not associated with later learning deficits though. Doubling the dose to 10 mg/kg resulted in neonatal rats exhibiting neuroapoptosis. Similarly, neonatal mice were susceptible to midazolam at a dose of 9 mg/kg, but neonatal rats were not. Clonazepam administered intraperitoneally or subcutaneously has also been implicated in causing neurotoxicity in neonatal rats.
Barbiturates
Pentobarbital in doses of 5–10 mg/kg and phenobarbital in doses of 40/100 mg/kg induce neuroapoptosis in 7-day-old rats.44 Thiopental, however, even in doses up to 25 mg/kg, did not induce apoptosis.39 Simultaneous administration of estradiol ameliorates the neurotoxicity of pentobarbital and phenobarbital in rats. The protective effects of estradiol are believed to be due to an increase in the concentration of survival proteins within the cell cytosol.45
Propofol
Subanesthetic doses of propofol administered to neonatal rats and mice have been implicated in increased levels of neuroapoptosis.46 Intraperitoneal propofol doses of 50–60 mg/kg cause increased neuroapoptosis and are associated with later neurocognitive deficits.46
Volatile Agents
Volatile anesthetic agents have both GABAergic as well as some NMDA antagonistic properties. However, the GABAergic properties predominate. Neonatal mice are susceptible to the neurotoxic effects of halothane and enflurane after only one-half-hour exposure prenatally. Fetal rats demonstrate learning deficits after a 2-hour prenatal exposure to halothane. In slightly older juvenile rats, prolonged exposure to low doses of halothane is associated with dendritic and synaptic density alterations.
Isoflurane exposure to P7 rats and mice is associated with neuroapoptosis after a 4-hour exposure when given in a concentration of 0.75%. Combinations of midazolam and nitrous oxide with isoflurane lead to a potentiated neurotoxic effect in both rats and guinea pigs.6
Sevoflurane even in subclinical doses when given for 2 hours or more has been associated with increased neuroapoptosis in mice pups. Six-hour exposures are associated with abnormal learning and social behaviors.47
Mechanisms
Several mechanisms have been postulated to explain anesthetic neurotoxicity in young animals. Understanding the mechanism for neuroapoptosis can aid in the development of ameliorative strategies to protect the developing brain.
Developing cells require external signals to guide their differentiation and synapse formation. General anesthesia can interfere with normal development by interrupting the cells’ ability to receive signals as well as the ability of neurons to send signals to other neurons. Cells that are isolated from trophic signaling will upregulate intracellular proteins that can send the cell into a death spiral. In contrast to ischemic cell death, apoptosis cell death is noninflammatory in nature and generally involves single scattered cells rather than groups of cells. Since the cell wall remains intact and intracellular contents are not released into the extracellular fluid, the inflammatory response to cell death is limited. General anesthetics, sedatives, and some anticonvulsants block or alter GABA and NMDA receptors involved in synaptic transmission, putting the neuron at risk for apoptosis. Additional stressors on neurons such as ischemia can also bias a cell toward apoptosis. With rare exceptions, apoptotic cell death is mediated by the caspase enzyme system in the cell cytosol. Staining for activation of the end products of this enzyme system allows for quantification of degree of apoptosis occurring within an organism. Apoptosis can be activated extrinsically by the death receptor pathway or intrinsically by release of proteins from the mitochondria.
Anesthetic drugs can activate the intrinsic pathway by causing the mitochondrial membrane to increase in permeability and release cytochrome C into the cytosol, which then activates the caspase system. There is an intracellular family of regulator proteins known as the Bcl-2 proteins that have two branches—proapoptotic proteins (BAX) and prosurvival proteins (Bcl-2). Activation of the intrinsic system is fairly rapid occurring within 2 hours of exposure. Melatonin has been found to stabilize the mitochondrial membrane, thereby decreasing the release of cytochrome C and thus maintaining the cell in a prosurvival state.48, 49
NMDA antagonists like ketamine and nitrous oxide are believed to induce cell death by a variety of mechanisms. Antagonism of the NMDA receptor in either a sustained fashion or repetitively causes upregulation of the NR1 subunit of the receptor. These upregulated neurons then require more trophic stimulation to maintain homeostasis. Lack of trophic stimulation leads to an increase in Ca2+ influx into the cell and eventually leads to neuronal cell death. Aberrant cell cycle entry has been implicated in cell death in cells exposed to ketamine. Isoflurane and nitrous oxide exposure alters BDNF and akt signaling, leading to activation of both the intrinsic and extrinsic pathways.21 Isoflurane also has been implicated in activation of the p75NTR receptor, which leads to neuroapoptosis.
Although there are many medications that have demonstrated the ability to block some of the neuroapoptotic pathways in immature anesthetically exposed cells, the clinical relevance of drugs is uncertain. The neurotoxicity of general anesthetics given to young infants has not been proven, and the efficacy and feasibility of administering these neurotoxic blockers to young children remain to be elucidated.
Mitigating Factors
Estradiol
Estradiol given to neonatal rats increases the intracellular levels of phosphorylated extracellular signal regulated kinase and akt. These enzymes are prosurvival enzymes. This hormone does not affect GABA and NMDA neuronal receptors in rats. (The positive effects on immature rats raise the specter that there may be gender-related differences in the neurotoxicity of general anesthetics.)
Melatonin
Melatonin, perhaps by stabilizing mitochondrial membranes, is effective in decreasing the anesthetic-induced neuroapoptosis in the anterior thalamus and cerebral cortex in 7-day-old rat pups.48, 49 It is plausible that since the intrinsic apoptotic pathway involves release of cytochrome C from mitochondria, stabilization of the mitochondrial membranes might lead to some protection from neuroapoptosis. Upregulation of the prosurvival proteins from the Bcl-X family may be another means by which melatonin is protective.
L-Carnitine
L-carnitine decreases the expression of the proapoptotic proteins in the BAX family and weakly increases the expression of the prosurvival proteins in the Bcl-X(L) family, thereby stabilizing the mitochondrial membranes.53 Neonatal rats given a combination of nitrous oxide and isoflurane for 6 hours along with L-carnitine were protected from AIDN.54
Lithium
Lithium increases the phosphorylation of the extracellular signal regulated protein kinase (ERK) in cells that are exposed to ketamine or propofol. Five-day-old rats were spared AIDN after exposure to these anesthetics when they were pretreated with lithium.55
Hypothermia
Moderate hypothermia decreases both normal background neuroapoptosis as well as anesthesia-induced neuroapoptosis.56 Rat pups that were cooled to 29°C showed no apoptotic response to isoflurane and ketamine exposure. The long-term effects of suppressing normal apoptosis by hypothermia are unknown and in fact may be deleterious.
Dexmedetomidine
The most promising AIDN ameliorating agent is dexmedetomidine. Although it is approved by the Food and Drug Administration (FDA) for sedation in adults, it is being used off label as a sedative in the neonatal and pediatric intensive care units. This medication is an α-2 selective adrenergic agonist with sedative, anxiolytic, and analgesic properties and is used as a general anesthetic adjunct and sedative. Most of the α-2 selective adrenergic receptors are located in the locus ceruleus which, when activated, cause sedation and anxiolysis. Other locations for these receptors include the spinal cord which, when activated, cause analgesia and the autonomic nerves, which, when activated, cause hypotension. The primary action of the α-2 receptors of the heart are chronotropic causing bradycardia, and dexmedetomidine causes a dose-dependent decrease in heart rate. The pharmacodynamic effects of this medication are initial bradycardia with a mild increase in blood pressure followed by bradycardia and hypotension. In addition, there are case reports of sustained severe hypertension in young children given glycopyrrolate to counteract bradycardia.57 It is not associated with neuroapoptosis in murine studies and may attenuate the apoptosis seen with isoflurane, although in some studies dexmedetomidine is associated with a modest increase in neuroapoptosis.54
Narcotics
Opioids are generally considered less neurotoxic than other sedatives. In young rats given narcotics in antinociceptive doses, there is no evidence of apoptosis in the spinal cord.
Local Anesthetics
Local anesthetics have been implicated in both in vitro and in vivo experiments in causing neuroapoptosis. They are associated with apoptotic and necrotic cell death in a dose-dependent manner. A study of rat neuron cells derived from the dorsal ganglion of P7 rat pups revealed that the earliest manifestations of lidocaine neurotoxicity were complete loss of mitochondrial membrane potential, followed by release of cytochrome C into the cytosol at concentration and caspase activation. Another experiment found that many local anesthetics investigated were neurotoxic at concentrations observed intrathecally after spinal anesthesia in humans.58 The in vitro toxicity of the local anesthetics correlated with their octanol/buffer partition coefficient and thus from a study examining a cell line derived from human neuroblastoma cells their relative clinical potency with the following order of apoptotic potency from high to low toxicity (tetracaine > bupivacaine > prilocaine = mepivacaine = ropivacaine > lidocaine > procaine = articaine).59 The neuroapoptotic potential of either ketamine or bupivacaine administered intrathecally to P7 rat pups revealed apoptosis in the ketamine but not the bupivacaine-exposed rats.25
Clinical Studies
There are several difficulties in extrapolating the results from rat and small mammal studies to the human population. In many of the animal studies, there has been a high mortality of the rat or mice pups while under anesthesia, fortunately a finding not found with human anesthetics. The reasons for this are clear; it is simply impossible to monitor the health of these tiny neonatal animals as carefully as is done in the most premature human infants. Several studies have tried to address this confounder with measuring serum glucoses and venous gases perioperatively.60 In addition, there is no certainty about when the time of potential vulnerability in humans would occur.
There are other formidable hurdles to adequately studying the issue of anesthetic toxicity in human infants. In general, the need for surgery or radiologic imaging studies in human infants is associated with underlying pathology, so prospective cohort studies or retrospective epidemiologic studies can be confounded by this underlying pathology. In addition, the traumatic effects of surgery such as perioperative fasting, transport, hypothermia, hemodynamic instability, and stress response secondary to surgical stimulus may independently affect neurologic outcomes. The magnitude of the effects of these confounders on eventual neurocognitive outcomes may mask any subtle deleterious effects that general anesthesia may cause in humans. The degree of presurgical morbidity may be one of the reasons that some infants had surgery for patent ductus arteriosus (PDA) and necrotizing enterocolitis rather than medical therapy. In children undergoing inguinal herniorrhaphies, a possible confounder might be a complicated respiratory neonatal course, which has been linked to both a higher incidence of inguinal hernias and poorer neurologic outcomes.61 These studies can also be affected by informational biases because of their retrospective nature. Prospective cohort studies, which can generally avoid informational biases, are also confounded by surgical and premorbid conditions of the participants. A meta-analysis of 23 clinical reports of patients undergoing neonatal surgery for major noncardiac congenital anomalies revealed lower cognitive scores in a median of 23% of the patients.62 Randomized controlled trials are very difficult to perform in children who require surgery because for most procedures there are not alternatives to general anesthesia. A single large prospective randomized trial known as the GAS trial compared the effects of general anesthesia with regional anesthesia in very young infants undergoing inguinal herniorrhaphies.63 A clear limitation of this trial is that the anesthetic exposure duration was limited.
Prenatal Human Exposure to General Anesthesia
There are very few studies examining the prenatal effects of general anesthesia on the neurocognitive outcomes of humans. As of this time, there are no studies looking at the effects of general anesthesia on development in which the general anesthetic was administered well before labor and delivery. A single nonrandomized study from Japan showed that 159 infants exposed to maternal nitrous oxide during the last stages of delivery had statistically different behaviors on postnatal day 5 compared to nonexposed infants.64 These infants exhibited weaker habituation to sound, stronger muscular habituation, less cuddling, and fewer smiles.
An epidemiologic study based on a birth cohort from Olmstead County in Minnesota found that children exposed to general anesthesia for elective cesarean section deliveries are not more likely to develop learning disabilities than those born of vaginal deliveries.65 One of the limitations of this study is that it involved two different modes of delivery. A separate study, using this same database, found that there was no impact of neuroaxial labor analgesia on the incidence of childhood learning disabilities.66
Neonatal and Young Childhood Human Exposure to General Anesthesia
In the literature are several studies that demonstrate an association between surgery and poor neurodevelopmental outcomes. In premature infants born before 27 weeks’ gestation, the Victorian Infant Collaborative Study Group found in a case control study design that infants who underwent surgery for PDA ligation, inguinal herniorrhaphy, gastrointestinal surgery, neurosurgery, and tracheotomy had an increased incident of cerebral palsy, blindness, deafness, and Wechsler Preschool and Primary Scales of Intelligence (WPPSI) < 3 SD below the mean.67 Several small studies as well as a large study involving almost 3,000 extremely low birth weight infants with a diagnosis of necrotizing enterocolitis found that there was a higher incidence of cerebral palsy and poor neurocognitive outcomes in those infants who were treated surgically compared with those treated with peritoneal lavage.68–71 However, the difficulties with adjusting for confounding by indication are illustrated by studies that show that infants undergoing isolated tracheoesophageal fistula repair in the neonatal period do not differ from the general population in terms of intelligence quotient (IQ) measurements.72, 73 Many studies involving infants with serious congenital heart disease have demonstrated an increased incidence of cerebral palsy, speech and language impairment, and motor dysfunction and lower IQs.74–77 A recently appreciated confounder in studies of cardiac infants involves the relative immaturity of brain development of these infants compared with normal infants.78 Serial neurocognitive examinations of a prospective cohort of infants born with transposition of the great vessels who were randomized to either circulatory arrest or cardiac bypass during the surgical repair found that the entire group was below population expectations for academic achievement, fine motor function, visuospatial skills, working memory, hypothesis generating and testing, sustained attention, and higher order language skills.74–76
Several studies have been published recently specifically examining whether the exposure to general anesthesia in infancy is associated with later learning difficulties. Researchers at the Mayo Clinic in Rochester, Minnesota, published a retrospective cohort study of over 5,000 children, of whom approximately 12% had one or more general anesthetics before the age of 4.4 This study found that there were significantly more reading, written language, and math learning disabilities in children who had been exposed to two or more general anesthetics but no increase in disabilities in those children who had been exposed to a single anesthetic. The risk of learning disabilities also increased with the cumulative duration of the general anesthesia. In another epidemiologic study, a birth cohort of 5,000 patients was identified from the New York State Medicaid billing codes.79 Of this group, 383 patients underwent inguinal herniorrhaphy at a young age. After controlling for gender and low birth weight, the authors found nearly a twofold increase in developmental and behavioral issues. A pilot study to test the feasibility of using a validated child behavior checklist in 314 children who had urologic surgery determined that there was more disturbed neurobehavioral development in children who underwent surgery prior to 24 months compared with those who underwent surgery after 24 months of age, although the differences between the two groups were not statistically significant.80 These studies are provocative, but the data do not reveal whether anesthesia itself may contribute to developmental issues or whether the need for anesthesia is a marker for other unidentified factors that contribute to these.
Another epidemiologic study from the New York State Medicaid dataset examined the developmental behavioral outcomes of 304 children with no risk factors for neurodevelopmental difficulties exposed to anesthesia before age 3 and compared them with a cohort of 10,450 siblings and found a 60% greater incidence of developmental or behavioral problem in children exposed to general anesthesia.81 A subsequent analysis of both New York and Texas Medicaid databases by the same group reaffirmed an increased rate of mental and developmental delays and attention-deficit disorder in children undoing surgery before 5 years of age. However, the incidence of these disorders was equivalent if the surgery occurred before or after 2 years of age, thereby refuting the practice of delaying surgery to reduce the potential of adverse neurocognitive deficits.82 A small retrospective study of 53 children who were exposed to general anesthesia before the age of 4 compared with children matched on age, gender, handedness, and socioeconomic status found that the exposed group of children had lower listening comprehension and performance IQ measurements.83 These decreased measurements were associated with lower gray matter density in the occipital cortex and cerebellum. The duration of anesthesia exposure for the children in this study averaged less than 1 hour.
Separate big data analysis from educational achievement scores in large cohorts (up to 34,000 pediatric patients) from Canada and Sweden divulged a slightly increased odds ratio of decreased education attainment in children exposed to surgery and anesthesia at age greater than 2–4 years.84–86 Scrutiny of these populations reveals a lower percentage in academic achievement scores for toddlers undergoing ear, nose, and throat surgery.86 This finding suggests that early derangements in hearing and speech may have an impact on subsequent cognitive domains assessed by school performance.87
In contrast to these findings, at least two other large studies have shown no association between receipt of general anesthesia and academic performance. A very large epidemiologic study comparing the academic performance of 2,689 children who had undergone inguinal herniorrhaphy in infancy to a randomly selected, age-matched control group consisting of 5% of the population derived from the Danish Civil Registration System from 1986 to 1990 found that after adjusting for known confounders, there was no statistically significant difference between the exposure and control groups.88 A similar finding was reported in a Mayo cohort study, which found no increase in learning disabilities in children exposed to a single anesthetic.4 Bartels et al. from the Netherlands also found no difference in the educational achievements of 1,143 identical twins who were discordant in their exposure to general anesthesia before the age of 3.89
In 2007, the GAS trial was initiated and enrolled 722 infants less than 60 weeks postmenstrual age over a period of 6 years. This trial is a randomized controlled equivalence trial comparing general anesthesia with sevoflurane with regional anesthesia with bupivacaine in infants undergoing inguinal herniorrhaphies. The interim 2-year follow-up results have been published and show that there were no differences on the Bayley–III examinations and other neurocognitive tests between the two types of anesthesia.90 The primary outcome measure—neurocognitive testing at age 5—has not yet been completed. The PANDA trial published results on 105 sibling pairs who were discordant for general anesthesia exposure before the age of 3 years.91 This small trial found no difference in mean IQ scores between exposed and nonexposed sibling pairs.
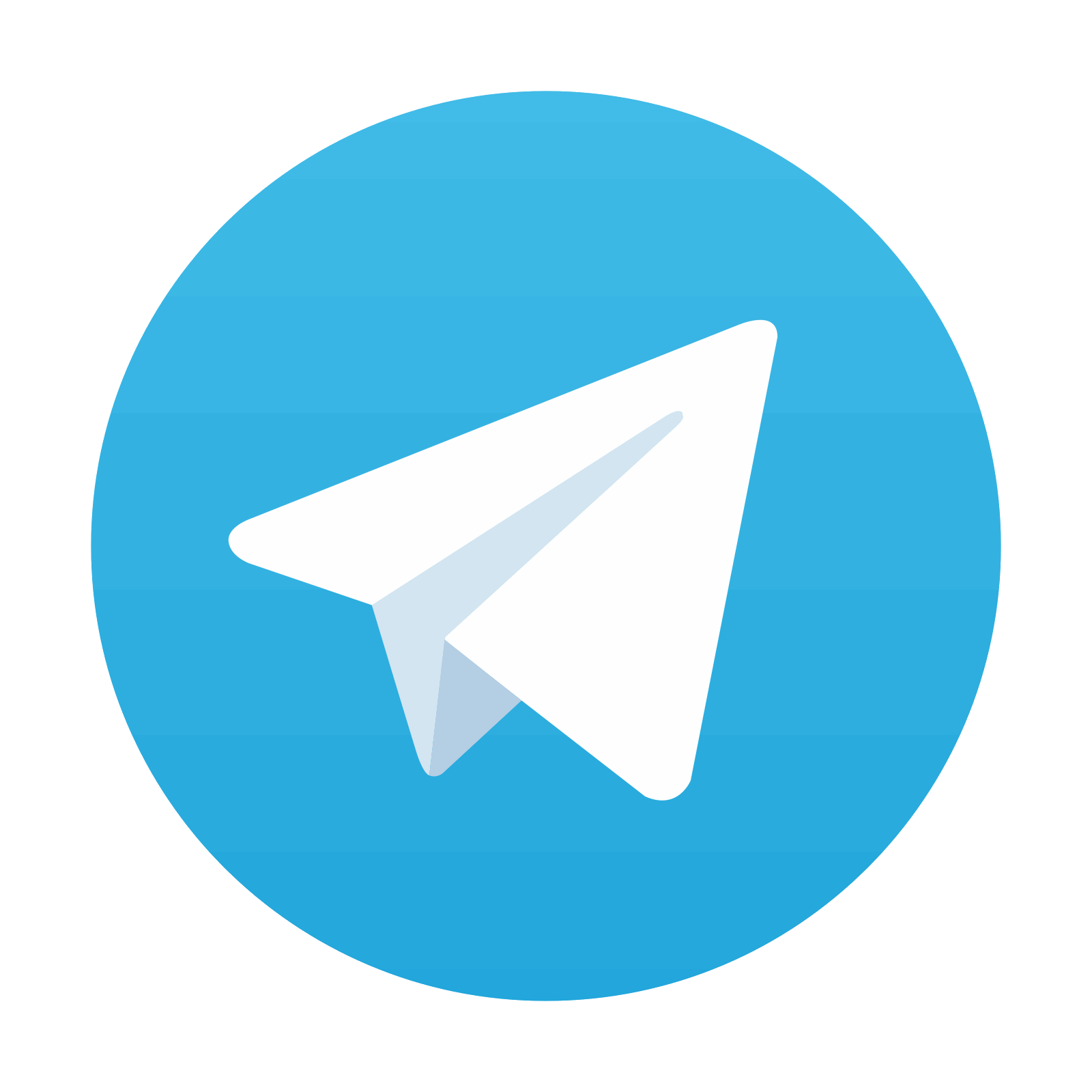
Stay updated, free articles. Join our Telegram channel

Full access? Get Clinical Tree
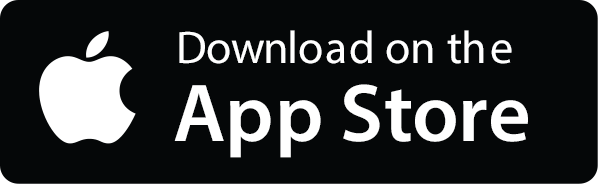
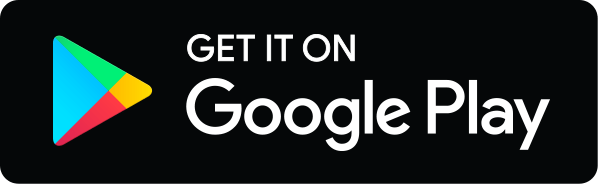
