Introduction
In 2015, it was estimated that more than 10,000 new cases of cancer were diagnosed in children aged 1–14 years in the United States.1 Although deaths from cancer have declined in the past couple of decades, cancer remains the second leading cause of death in children. Currently, acute lymphocytic leukemia accounts for about 31% of childhood cancer cases, and central nervous system including spinal cord tumors around 21%.
Central Nervous System Tumors in Children
The most common types of brain tumors in children are astrocytoma, medulloblastoma, and ependymoma.
1. Astrocytomas are tumors that arise from astrocyte, star-shaped cells that make up the supportive tissue of the brain. They can appear in various parts of the brain and nervous system, including the cerebellum, the cerebrum, the diencephalon of the brain, the brainstem, and the spinal cord. The different types of astrocytomas are the following:
a. Pilocytic astrocytoma: these tumors usually stay in the area where they started and do not spread. Less known astrocytomas are cerebellar and desmoplastic infantile gangiloglioma.
b. Diffuse astrocytoma: these astrocytomas tend to invade surrounding tissue and grow at a relatively slow pace.
c. Anaplastic astrocytoma: these rare astrocytoma tumors require more aggressive treatment.
d. Astrocytoma grade IV: primary tumors in this group are very aggressive and are the most common astrocytoma grade IV. The secondary tumors originate as a lower-grade tumor and then evolve into a grade IV tumor.
2. Medulloblastomas are fast-growing and high-grade and are the most common of the embryonal tumors. They usually are located in the cerebellum, and it is unusual for them to spread outside the brain and spinal cord. Medulloblastomas are relatively rare, accounting for roughly less than 2% of all primary brain tumors.
3. Ependymomas arise from the ependymal cells that line the ventricles of the brain and the spinal cord. Ependymomas can appear in different locations within the brain and spinal column. They account for about 2–3% of the primary brain tumors. Ependymomas can be of four different types:
a. Subependymomas: typically slow-growing tumors that appear near a ventricle.
b. Myxopapillary ependymomas: slow-growing tumors that tend to grow in the lower spinal column.
c. Ependymomas: the most common of the ependymal tumors, located along or within the ventricular system.
d. Anaplastic ependymomas: these are faster growing, mostly posterior fossa tumors in children.
Considerable advances in cancer treatment have led to an increase in survival rates. In the last four decades, overall survival rates have increased from just 10% to nearly 90% today. Factors contributing to these increased survival rates include sophisticated early detection, better chemotherapeutic agents, refined surgical techniques, and improvement in radiation therapy.
The anesthesiologist remains a key part of the multidisciplinary team that cares for a child with cancer, starting from diagnosis, surgery, chemotherapy, and radiotherapy with pain management to later surveillance. Radiotherapy has an increasing role in brain tumors. Knowledge of the multiple effects of cancer process, treatment, toxic effects, and complications is important for any anesthesiologist involved in the care of these children who undergo radiation therapy.
Goals of Radiotherapy
Radiotherapy may serve as:
Primary therapy
Adjuvant or neoadjuvant therapy
Curative therapy
Palliative therapy
Therapeutic radiation can be in the form of:
Ionizing radiation
Electromagnetic radiation—characterized by high energy and absence of mass, short wavelength, greatest energy on the electromagnetic spectrum
X-rays produced when a stream of fast-moving electrons accelerated by high voltage strike the target and give up their energy (betatrons, linear accelerators)
The purpose of radiotherapy is to deliver therapeutic doses of radiation to the tumor while limiting damage to the surrounding healthy tissue. Advancements in three-dimensional imaging have produced modalities such as three-dimensional conformational radiation therapy and intensity-modulated radiation therapy,2–5 which help concentrate the energy beam on the tumor while reducing radiation to the normal surrounding tissue.
Radiosensitivity of a cell is determined by its phase in cell cycle and by its nutrient and oxygen content. Therefore, healthy tissues have by far a greater capacity to repair damage from ionizing radiation, but they need time to do so. For that reason, the total dose of radiation is divided into a series of treatments. Additionally, by applying radiation at different times, the tumor cells that are in a relatively radio-resistant phase during one treatment are likely to cycle to a sensitive phase for a subsequent treatment.
Effects of Radiotherapy on a Cellular Level
Radiotherapy exerts its cytotoxic effects by generating free radicals, which cause lysis of cell membranes, DNA strand breakage, and RNA damage by ionization. Cellular kinetics determine the speed of cell death.
Airway Problems
Tumors involving the airway are relatively rare in pediatric patients. However, central nervous system (CNS) tumors with superior vena cava syndrome, large cervical, and retropharyngeal lymph nodes from leukemic infiltration may present as stridor. Complications to maintenance of the airway may become evident when the patient is lying down or anesthetized. After radiotherapy, these patients need to be evaluated for fibrosis of tissues, as distortion of airway anatomy leads to limited mouth opening, limited neck extension, supra- and subglottic edema, stenosis, and xerostomia. Furthermore, obliteration of lymphatics can lead to postoperative edema that causes airway problems.
Toxicity from Treatment
In cancer patients, the disease process itself has certain implications for anesthesia, depending on the site, type, and extent of involvement, with additional considerations coming from the effects of chemotherapy and radiotherapy (Table 18.1). Toxicity from chemotherapy and radiotherapy is unavoidable. The developing tissues of children are particularly susceptible to these effects. It is very important to evaluate these patients carefully for anesthesia and to detail the anatomical and physiological effects from the cancer, the treatment, and the potential toxicity.6
Chemotherapy Agent | Complications |
---|---|
Anthracyclines | Myelosuppression |
Cardiomyopathy | |
Arrhythmias | |
Bleomycin | Pneumonitis |
Pulmonary fibrosis | |
Hypersensitivity | |
Cisplatin | Neurotoxicity |
Nephrotoxicity | |
Cyclophosphamide | Myelosuppression |
Cardiomyopathy | |
Arrhythmias | |
Hepatotoxicity | |
Hemorrhagic cystitis | |
5-fluorouracil | Myelosuppression |
Alopecia | |
Methotrexate | Myelosuppression |
Pneumonitis, pleural effusion | |
Mucositis | |
Renal failure | |
Procarbazine | Myelosuppression |
Pulmonary toxicity | |
Neurotoxicity | |
Leukemia | |
Vincristine | Myelosuppression |
Mucositis | |
Peripheral neuropathy | |
Syndrome of inappropriate ADH secretion |
Factors that determine the susceptibility to radiotherapy toxicity2 include:
The total and fractionated dose received
Sensitivity of the tissue
Anatomy and extent of the tissue irradiated
Course of the treatment
Recent surgery and concurrent chemotherapy
Cardiotoxicity
When chemotherapeutic agents like anthracyclines (doxorubicin, daunorubicin, and alkylating agents) and cyclophosphamide are used in chemotherapy or radiation, cardiotoxicity can manifest early to late as myocarditis, pericardial effusion, myocardial depression, arrhythmias, endomyocardial and valvular fibrosis, conduction defects, and cardiomyopathy. Subclinical cardiomyopathy can become evident during the perioperative period. Cardiac failure is the most common nonmalignant cause of death in survivors of Hodgkin’s disease. Therefore, the anesthesiologist should look for physical signs and use surveillance echocardiography during preoperative assessment in this group of patients.
Factors that predispose patients to cardiotoxicity from chemotherapy and radiotherapy include:
Chemotherapy agent and the combination used—lower the total cumulative dose to reduce risk for cardiomyopathy
Total dose administered (anthracyclines—more than 300 mg/m2)
Route of administration—systemic administration leads to multiorgan injury
Concurrent radiotherapy (anthracyclines—200 mg/m2 with mediastinal radiation)
Preexisting heart disease
Cancer survivors who underwent radiation therapy for conditions such as neurofibromatosis type 1, craniopharyngioma, and optic tumors may present with moyamoya syndrome, in which the carotid arteries narrow and develop collaterals. Moyamoya is seen usually 5 years after radiation, particularly in patients who were young at the time of radiotherapy.7
Pulmonary Toxicity
Pulmonary toxicity can be evident as an early or late effect. Cancer patients treated with busulfan, bleomycin, methotrexate, carmustine, or cyclophosphamide can present with dyspnea, dry nonproductive cough, and fever. Acutely they can present with malignant pleural effusions that require symptomatic relief with thoracocentesis before anesthesia. Acute noncardiogenic pulmonary edema, bronchiolitis obliterans, and drug-induced pneumonitis that progresses to pulmonary fibrosis are seen as late complications in patients treated with bleomycin. Anesthesiologists should be particularly cognizant of these possible effects because patients presenting for anesthesia after bleomycin chemotherapy need to be titrated carefully to the minimum oxygen possible to maintain oxygenation but at the same time avoid bleomycin-induced lung injury.
At radiation doses greater than 15–20 GY, radiation-induced pulmonary complications appear similar to chemotherapy-induced lung injury. Pulmonary changes are evident as restrictive defects on pulmonary function tests, increased alveolar-arterial gradient, reduction in forced vital capacity, and decreased carbon monoxide diffusing capacity. Anesthesiologists should keep in mind that they should review these tests to assess the severity and effects on anesthesia.
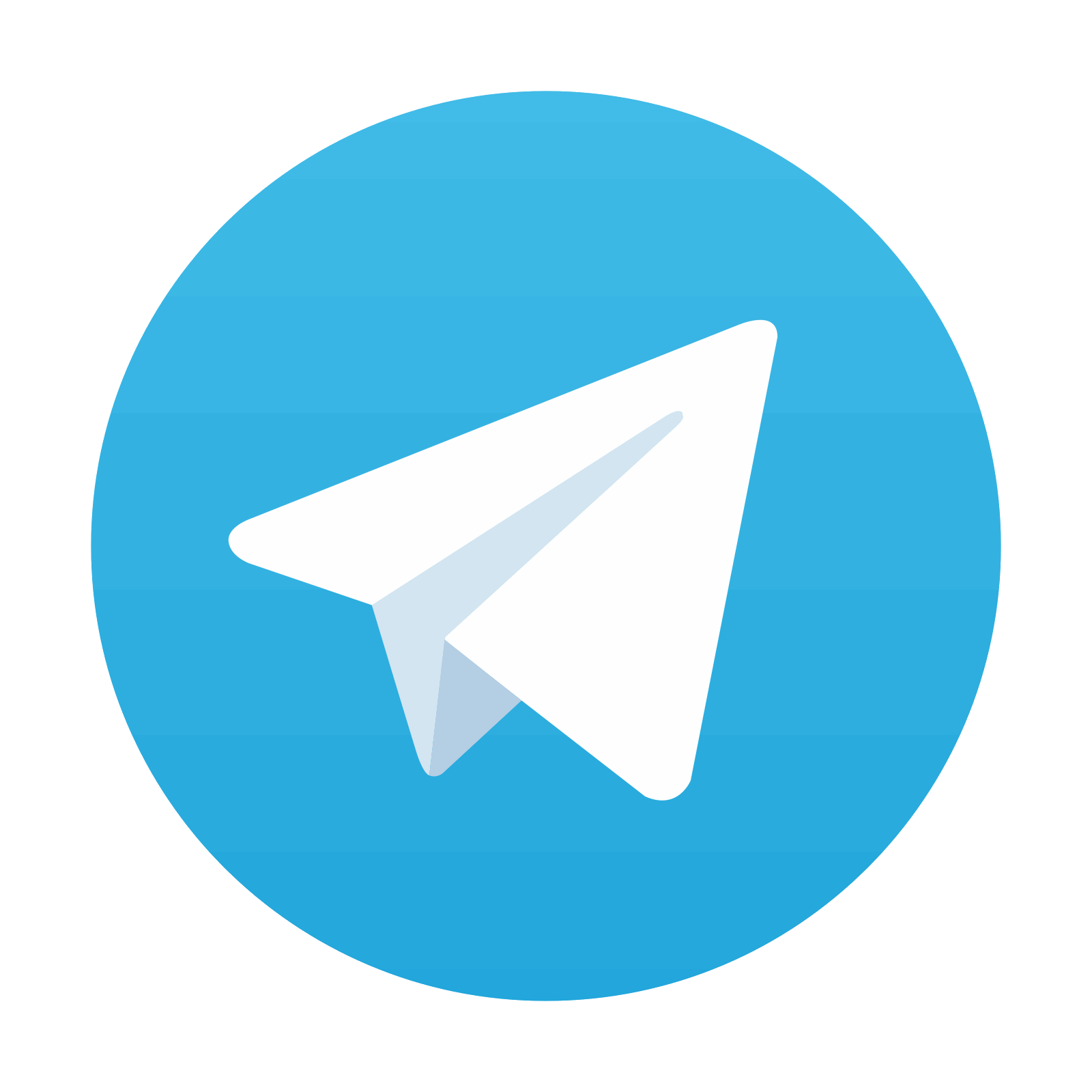
Stay updated, free articles. Join our Telegram channel

Full access? Get Clinical Tree
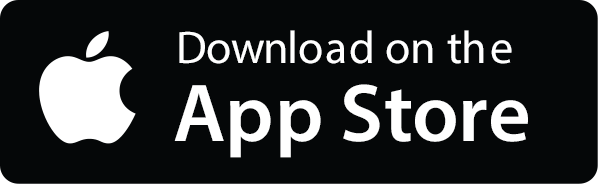
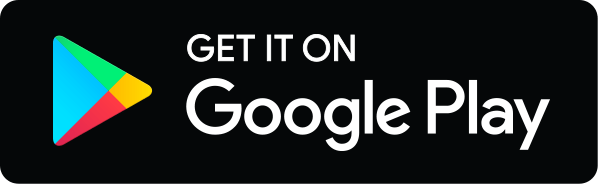
