Introduction
Traumatic brain injury (TBI) is a leading killer of children over 1 year of age, most often due to falls, and second most often due to motor vehicle crashes. However, the incidence of nonaccidental abusive head trauma (AHT) and sports-related head injury continues to rise in children. The sequelae of TBI affect the patient and the entire family unit for years after the initial injury. Mortality is highest after severe TBI, but the public health burden is from the large number of patients with mild TBI. In the acute phase TBI can present along a continuum from a brief change in mental status or consciousness (concussion or mild TBI classified by Glasgow Coma Scale score [GCS] > 8; Table 14.1) to an extended period of unconsciousness or amnesia after the injury (severe TBI classified by GCS < 9). After TBI, patients may have seizures, motor or sensory deficits, inability to protect the airway, respiratory insufficiency, and hemodynamic instability. In the healthy brain, homeostasis is maintained through a complex and well-coordinated system that includes, but is not limited to, an intact blood-brain barrier, cerebrovascular autoregulation with good matching of local cerebral blood flow with cerebral metabolic rate for oxygen (CMRO2), and neurohormonal output that can modulate blood osmolarity, cardiac output, and ventilation. TBI can result in derangements in any of these homeostatic mechanisms. The sequela of TBI are the result of both the “primary injury,” caused by direct mechanical insult to the brain parenchyma or vascular supply, and the “secondary injury,” marked by diffuse cerebral swelling due to second insults caused by edema, hypoxia, hypotension, intracranial hypertension, and inflammation. The goals of anesthetic and perioperative management are focused around providing adequate surgical conditions while best preserving normal physiologic function, thereby limiting secondary injury. Achieving these goals requires an understanding of the various manifestations of TBI, and the interventions available to limit their progression and to compensate for their dysfunction.1 Given that many children with TBI will have polytrauma, a multisystem assessment is imperative. Basic life support algorithms including Advanced Trauma Life Support (ATLS) and Pediatric Advanced Life Support (PALS) provide a framework for the initial resuscitation and should be immediately applied to ensure optimal oxygenation and circulation.2 The 2012 Pediatric Guidelines for managing children with severe TBI have been published by a multidisciplinary group and provide evidence-based guidance on the treatment of pediatric patients.3
Glasgow Coma Scale | Pediatric Coma Scale | Infant Coma Scale | Score |
---|---|---|---|
Eyes | Eyes | Eyes | |
Open spontaneously | Open spontaneously | Open spontaneously | 4 |
Verbal command | React to speech | React to speech | 3 |
Pain | React to pain | React to pain | 2 |
No response | No response | No response | 1 |
Best verbal response | Best verbal response | Best verbal response | |
Oriented and converses | Smiles, oriented, interacts | Coos, babbles, interacts | 5 |
Disoriented and converses | Interacts inappropriately | Irritable | 4 |
Inappropriate words | Moaning | Cries to pain | 3 |
Incomprehensible sounds | Irritable, inconsolable | Moans to pain | 2 |
No response | No response | No response | 1 |
Best motor response | Best motor response | Best motor response | |
Obeys verbal command | Spontaneous or obeys verbal command | Normal spontaneous movements | 6 |
Localizes pain | Localizes pain | Withdraws to touch | 5 |
Withdraws to pain | Withdraws to pain | Withdraws to pain | 4 |
Abnormal flexion | Abnormal flexion | Abnormal flexion | 3 |
Extension posturing | Extension posturing | Extension posturing | 2 |
No response | No response | No response | 1 |
Approach to the Pediatric Trauma Patient
Acute Resuscitation
Definitions of hypotension have varied across the published TBI literature, but most agree that systemic hypotension is associated with poor outcomes. Systolic blood pressure (SBP) < 5th percentile for age, and SBP < 70 + 2 (age) are the most commonly used definitions of systolic hypotension. This roughly corresponds to a SBP < 90 mmHg for children over 11 years of age. With polytrauma, hypotension and hypovolemic shock are often due to blood loss from solid organs, large vessels, and long bone injuries. Infants can present with hypovolemic shock even with an isolated TBI due to the relatively large head-to-body ratio and the vascularity of the scalp. Additionally, both TBI and spinal cord injury can lead to neurogenic shock and hypotension refractory to volume resuscitation, requiring the use of vasoactive agents. Hypoxia during the preoperative period can compound the adverse effect of hypotension.4 In the context of polytrauma, a multidisciplinary team including the relevant surgical specialists, anesthesiologists, radiologists, and intensivists should triage which surgical interventions are required following a “damage control” approach. Appropriate hemodynamic resuscitation has resulted in a reduction in hospital mortality and low GCS at discharge.5 Acute traumatic coagulopathy is also associated with high mortality,6 and blood loss should be treated with timely and specific blood component therapy.
Cerebral Perfusion Pressure
An important reason to correct systemic hypotension is to optimize cerebral perfusion pressure (CPP),2, 3 which is defined as mean arterial pressure (MAP) minus the greater of the intracranial pressure (ICP) or the central venous pressure (CVP). CPP represents the driving pressure for flow through the brain. Since arterial pressure and ICP are objective data points often available in TBI patients, CPP has become a target of interest as a clinical endpoint, with clinicians targeting a range of CPP that avoids cerebral hypoperfusion and hyperemia. Both under- and overperfusion to the injured brain can worsen secondary injury following TBI, the former by worsening ischemia and the latter by worsening edema or hemorrhage. There is paucity of data to suggest the best target CPP in pediatric TBI based on age, but there is some evidence an age-related continuum does exist. On the other hand, studies aiming to determine the lower limit of autoregulation (LLA) show that even young children may have an LLA of 60 mmHg, close to the resting MAP and that the LLA varies within age (see Chapter 2).7, 8 Cerebral autoregulation is often compromised in TBI and is associated with a poor prognosis.9 Perioperative assessments of cerebral autoregulation have been attempted in acute TBI, but these experimental modalities are not widely available.10–12 Therefore, in general, hypotension secondary to trauma should be aggressively treated. This can be achieved by ensuring large bore intravenous access and restoration of fluid deficits with crystalloid and blood products, as well as with vasopressors. Level III evidence from the multidisciplinary Pediatric Guidelines for TBI recommend that CPP < 40 mmHg be avoided after severe TBI.13, 14 Additional Level III evidence supports keeping CPP between 40 and 50 mmHg.3 Given the variability of the LLA, especially in TBI, empiric CPP management may not have an expected effect on CBF.7, 10 In the absence of ICP monitoring, supranormal SBP may be targeted to ensure an adequate CPP. Maintenance of CPP > 40 mmHg during craniotomy for TBI is associated with improved discharge survival, and TBI guideline adherence overall is associated with survival benefits.15
Airway Management
Children with a GCS score < 9 are considered unable to protect their airway and are at high risk for upper airway obstruction and/or pulmonary aspiration. These patients require tracheal intubation followed by emergent neuroimaging to delineate the extent of the injuries.16 Immobilization of the cervical spine is essential to avoid secondary spinal cord injury, especially during manipulation of the airway, until clinical and/or radiological clearance is confirmed. It should be noted that infants and young children are predisposed to C1–2 cervical disruptions, which may be difficult to diagnose in cervical spine x-rays. There should be minimal cervical spine manipulation during tracheal intubation of infants with TBI. Spinal cord injury without radiological abnormalities (SCIWORA) manifests as neurological deficit without fracture due to ligamentous and paraspinous muscle laxity. Nasotracheal intubations are relatively contraindicated in these patients because of the possibility of basilar skull fractures.
Abusive Head Trauma (AHT)
Infants with AHT often present with a myriad of chronic and acute subdural hematomas.17, 18 When compared to nonintentional TBI, patients with AHT tend to be younger and present with apnea and seizures.18 As with all TBI, the presence of other coexisting injuries, fractures, and thoracic and abdominal trauma should be identified and stabilized.19 Patients with injuries out of proportion to the history of TBI should be considered at risk of AHT.
Concussion
A concussion may lead to secondary injury due to worsening functional and biochemical changes in the brain and can lead to diffuse axonal injury. The impact of general anesthesia in pediatric patients with a recent concussion or mild TBI is unknown and is a major knowledge gap in perioperative medicine.20, 21 Although neuroimaging studies (computed tomography (CT) and magnetic resonance imaging (MRI)) are normal in 78% of pediatric patients with concussions, intracranial and intraparenchymal hemorrhages, nonhemorrhagic contusion, and nonspecific white matter changes are noted in the rest.22 General anesthesia is frequently associated with alterations of blood pressure and arterial partial pressure of carbon dioxide, and large volumes of intravenous fluids may be administered in response to surgical blood loss and hypotension due to anesthesia-induced vasodilation. Significant systemic hypotension, hypo- or hypercarbia, intracranial hypertension, and hyperglycemia have been observed in a cohort of pediatric patients with TBI undergoing extracranial surgery.23 A recent study of hospitalized children with sports-related concussion demonstrated impaired cerebral autoregulation.24 Therefore, close intraoperative monitoring of these hemodynamic variables is essential in preventing secondary injury to a vulnerable brain.23, 24 Many clinicians consider it best practice to delay elective surgery in patients with known concussion until the patient is free of symptoms.
Indications for Surgery
Refractory Intracranial Hypertension
The goal of neurosurgery in TBI is to terminate the vicious cycle of brain swelling and intracranial hypertension. Brain edema worsens intracranial hypertension, which reduces CPP, cerebral blood flow (CBF), and brain oxygenation. Physical examination and neuroimaging will dictate the need for emergent surgery and placement of ICP monitors. Indications for expediting neurosurgical interventions include the following:
1. Cranial
a. Epidural hematoma
b. Subdural hematoma
c. Penetrating injury
d. Medically refractory intracranial hypertension with or without herniation
2. Extracranial
a. Long bone fractures
b. Abdominal visceral injury
c. Thoracic injuries
d. Spine injuries
Patients with severe brain swelling with sulci effacement and midline shift on CT or refractory intracranial hypertension are candidates for decompressive craniectomy. A randomized trial comparing decompressive craniectomy versus advanced medical management in patients with severe and refractory intracranial hypertension resulted in decreased mortality and ICP, but increased adverse events and disability in the surgical cohort.25 The increased frequency of diffuse swelling in the pediatric population makes children more frequently candidates for such treatment.26 Decompressive craniectomy with duraplasty may be considered when intracranial hypertension reaches or approaches medical refractoriness in salvageable patients where the ICP elevation and its effects are felt to be the major threat to recovery.3 Unilateral craniectomy is appropriate for lateralized swelling. Bifrontal decompression is selected for diffuse edema, which in a mixed population of head injured patients resulted in favorable outcome in patients with (1) preoperative ICP < 40 mmHg, (2) treatment within 48 hours of presentation, and (3) age < 18 years.27 Early decompressive craniectomy within 24 hours after the time of injury is associated with a significant decrease in ICP and improved 6-month outcome in children.28 Bifrontal or frontotemporal craniectomy for management of refractory intracranial hypertension from AHT resulted in a significant decrease in mortality in the craniectomy group when compared to the medically managed children.29 Early decompressive craniotomy resulted in 16 out of 23 children surviving, with 81% of the survivors returning to school with a reasonable quality of life.30 Other studies in children demonstrate the utility of decompressive craniectomy in reducing refractory intracranial hypertension.31 Therefore, decompressive craniectomy may have a dual role as an early and late attempt at managing moribund head injured infants and children.32
Skull Fractures
Skull fractures are the most common head injuries in pediatric patients and are frequently caused by falls. Most asymptomatic linear skull fractures do not require treatment and are observed. Compound, open, or depressed skull fractures may cause dural and/or parenchymal injury and need to be surgically explored, debrided, and repaired.
Mass Lesions
Surgical evacuation of mass lesions in comatose patients should be performed expeditiously. Epidural hematomas can expand rapidly and should be evacuated in comatose patients. Subdural hematomas that are greater than 10 mm thick or produce a midline shift of > 5 mm are associated with herniation. Enlarging intraparenchymal mass lesions manifest as progressive neurological deterioration and can lead to refractory intracranial hypertension. If there is minimal or no intracranial mass effect, penetrating injury may be managed with local debridement and watertight closure. Reduction of severe, refractory intracranial hypertension may warrant surgical resection of hemorrhagic and edematous brain tissue.
Surgical Decision Making and Perioperative Communication
Neurosurgical intervention for TBI often occurs early in the acute phase when active resuscitation is ongoing. Clear and ongoing communication between the entire perioperative team should address whether there is a need to stage the procedure, the extent of ongoing blood loss, systemic stability, and any other unanticipated events that might alter the procedure, including the decision to abort if necessary. Intraoperative blood transfusion greater than 40 mL/kg is associated with an increased incidence of 30-day mortality, clearly demonstrating a correlation between the volume of red blood cells transfused and incidence of adverse outcomes.33 This finding provides a metric for terminating the surgery and stabilizing the patient if prudent. Once the neurosurgical procedure is completed, tracheal intubation and mechanical ventilation should be maintained, and the patient must be managed in an intensive care unit with full hemodynamic monitoring.
Cerebral Resuscitation
Intracranial Pressure Monitoring
The use of ICP monitoring in infants and children with severe TBI with a GCS ≤ 8 may be considered (Level III evidence).3, 34 However, a recent report related that routine ICP monitoring in pediatric TBI did not improve functional outcome.35 Symptoms of increased ICP are nonspecific in children. Intermittent apnea may be the first sign of raised ICP in infants. Generally, low thresholds are maintained for monitoring unconscious patients since physiologic parameters are less sensitive than mental status changes. In infants, widening sutures and protuberant fontanels are evidence of increasing ICP. With TBI, the CMRO2 and oxygen extraction can be low, and if intracranial hypertension develops, there is a corresponding decrease in CBF.36 These findings reveal that cerebral hemodynamics and CMRO2 are in flux after TBI. Level III evidence recommends that the treatment may be considered for an ICP threshold of 20 mmHg. ICP measurements from ventricular catheters and fiberoptic intraparenychymal transducer have a good correlation.37 Ventricular catheters have the advantage of acting as a conduit to withdraw cerebrospinal fluid, which can be a therapeutic option to reduce ICP.
Medical Management of Raised ICP
Intracranial hypertension can be medically managed initially. Therapeutic interventions to lower ICP include measures to improve venous drainage, encourage fluid shifts out of the brain parenchyma, and reduce the cerebral metabolic rate. Improvements in venous drainage can be achieved by elevating the head of the bed, ensuring appropriately fitted neck immobilization collars or endotracheal tube ties, and minimizing intrathoracic pressures (by minimizing ventilation pressures and the use of positive end expiratory pressure [PEEP] or with neuromuscular blockade). Hyperosmolar therapy can encourage free water shifts out of the brain parenchyma. Mannitol can be given at a dose of 0.25 to 1.0 g/kg intravenously. However, repeated dosing may theoretically worsen cerebral edema.38 The administration of hypertonic saline has been shown to improve various clinical parameters but not survival rate.39 Hypertonic saline (3% NaCl) decreases ICP and increases CPP, with a loading dose of 3–5 mL/kg followed by an infusion of 0.1–1.0 mL/kg/hr.40 Its utility to maintain serum osmolarity below 360 mOsm/L is supported by Level III evidence.3 A reduction in the CMRO2 may be accomplished with a variety of sedative-hypnotic drugs. High-dose barbiturate therapy can be titrated to produce a burst suppression pattern on the electroencephalogram, which results in a reduction in CMRO2. Refractory intracranial hypertension can be treated with thiopental infusions, but volume loading and inotropic support may be needed to counter myocardial depression and hypotension. The empiric administration of steroids in not supported by Level II evidence.
Hyperventilation has traditionally been utilized to acutely treat intracranial hypertension.41 Current guidelines recommend maintaining normocapnia except in the presence of impending herniation when brief hyperventilation can be used as a tool to temporize the situation.3 Hyperventilation, and the resultant cerebral vasoconstriction, and increased CMRO2 may worsen the supply demand perfusion ratio to tissue already compromised by injury.42, 43 Aggressive hyperventilation in the absence of cerebral herniation is associated with poor discharge survival.15 Thus, it is particularly important to recognize that small children are subject to inadvertent overventilation and that hyperventilation-associated cerebral ischemia can occur. Careful monitoring of blood gases, minute ventilation, and use of end-tidal carbon dioxide tensions are recommended. If these maneuvers fail to control elevated ICP, decompressive craniectomy should be considered (see above). Neurogenic pulmonary edema and acute respiratory distress syndrome can compromise oxygenation and should be treated expectantly.
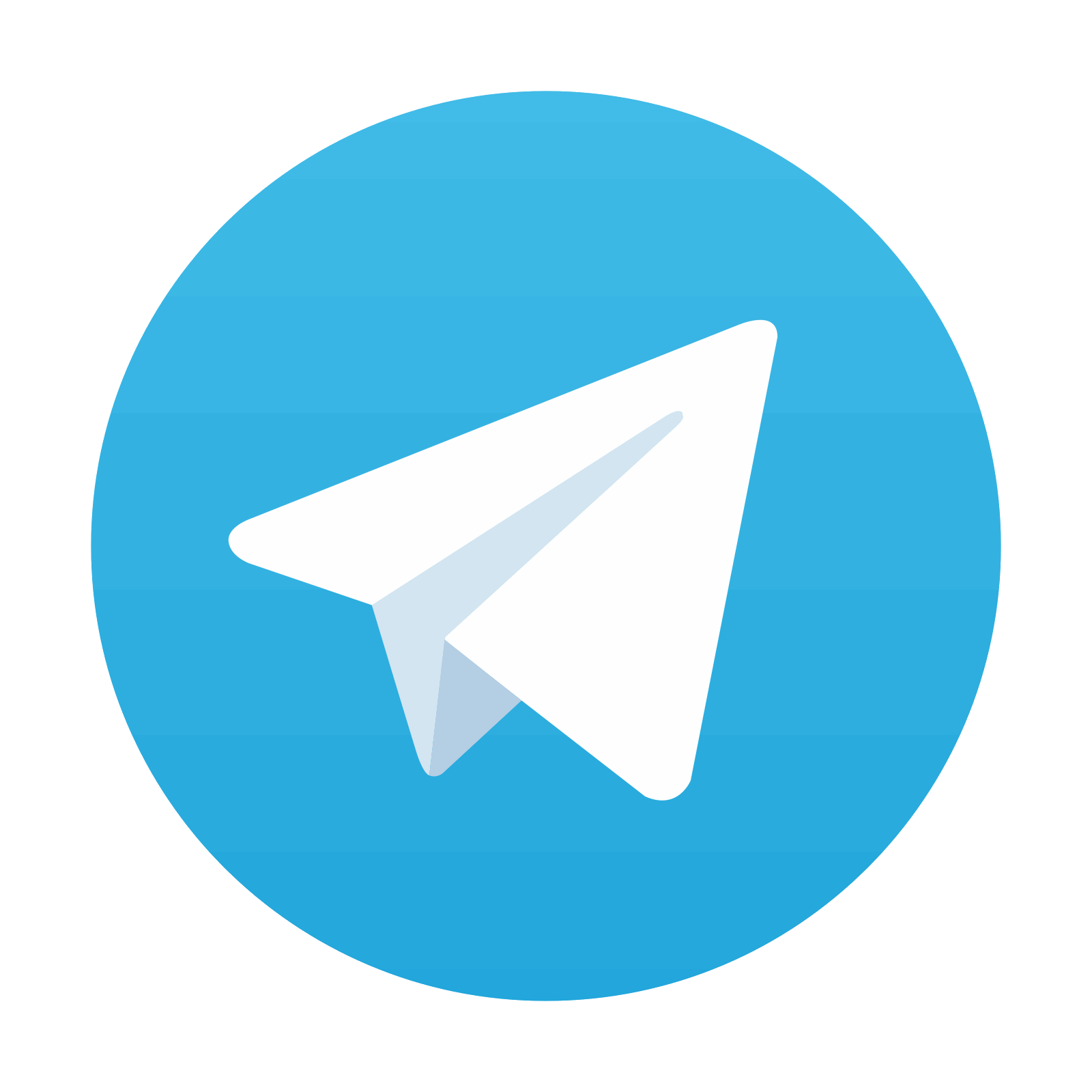
Stay updated, free articles. Join our Telegram channel

Full access? Get Clinical Tree
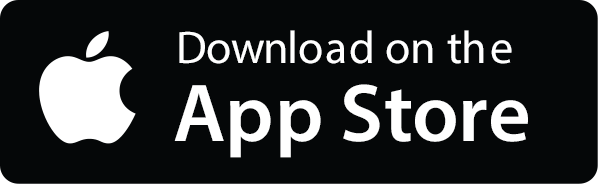
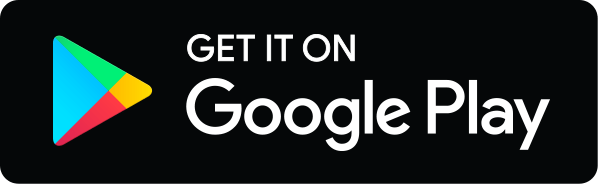
