Introduction
Epilepsy is one of the most common neurologic disorders. There has been significant improvement in the medical management of epilepsy. Despite the development of new drugs and treatment regimens, the prevalence of pharmacologically intractable seizures is still high, leading to significant developmental delays. Intractable epilepsy is defined as failure of more than two antiepileptic drugs and having more than one seizure per month over a period of 18 months. Advances in neuroimaging techniques and electroencephalography (EEG) have provided epileptologists with surgically resectable anatomic targets that mediate some of these medically intractable seizure disorders.1 Neurosurgeons have utilized these technologies to dramatically improve outcomes in these patients.
Within this population, there are a few notable differences between children and adults. The developing brain has a lower seizure threshold, which results in a more frequent occurrence of catastrophic epilepsy in young children. Cerebrovascular physiology is different in children. Compared to adults, infants have more of their cardiac output directed toward the brain, resulting in a greater cerebral blood volume. That distinction, coupled with the fact that children have a lower baseline mean arterial pressure than adults, puts infants at greater risk of hemodynamic instability during neurosurgical procedures. Neonates have decreased hepatic function—leading to delayed metabolism of drugs—and decreased renal function, which limits their ability to compensate for changes in fluid and solute loads. Combined, these may alter the clearance of medications administered to neonates.
Perioperative Considerations
Basics of Epilepsy Terminology
Prior to embarking on the workup and treatment of epilepsy, it is necessary to understand the basic anatomy and physiology used to describe these phenomena. The ictal onset zone refers to the region where clinical seizures originate. The epileptogenic lesion refers to the structural lesion that is causally related to the epilepsy. The epileptogenic zone refers to the region of cortex that actually generates epileptic seizures. Last, the irritation zone refers to the cortex that generates abnormal EEG discharges between seizures known as interictal spikes
Preoperative Evaluation
1. A thorough preoperative evaluation is necessary for any patient presenting to the operating suite. A history and physical examination must be carefully performed as many of these patients have significant comorbidities. One should pay careful attention to the respiratory and cardiac systems. Cardiac function should be optimized prior to surgery. A complete airway examination should be performed as some craniofacial anomalies may require special techniques to secure the airway. The evaluation should also attempt to detect underlying conditions that are leading to the seizures and to describe disabilities resulting from progressive neurologic dysfunction.2
2. Preoperative laboratory testing should include a complete blood count to assess for a baseline hematocrit level and coagulation studies to look for any unknown coagulation disorders. Given the significant blood loss that can occur during the procedure, underlying coagulopathies should be corrected preoperatively. Typed and screened or cross-matched blood should be available.
3. Serum anticonvulsant levels should be determined preoperatively to detect subtherapeutic or toxic concentrations. The anticonvulsant medications that most of these patients require often have notable side effects:
a. Valproic acid is known to cause abnormalities of hematologic function—such as abnormal coagulation, depression of red or white blood cell production, or decreased platelet counts—that can be especially concerning during intracranial surgery.3
b. Many anticonvulsants enhance the metabolism of non-depolarizing muscle relaxants and opioids via upregulation of the cytochrome P450 system. Hence, an increased amount of these drugs may be a necessary component of the anesthetic plan (Figure 13.1). Newer anticonvulsants seem to have less of an effect on the metabolism of anesthetic drugs.4
4. Special consideration should be given to patients with a history of tuberous sclerosis. This hamartomatous disease presents with cutaneous and intracranial lesions, with lesions infiltrating the cardiac, renal, and pulmonary systems. Preoperative electrocardiograph (EKG) and echocardiogram should be performed to assess for functional defects from the possible cardiac rhabdomyomas leading to obstruction of intracardiac blood flow, dysrhythmias, or abnormal conduction pathways. Renal lesions can lead to hypertension or decreased renal function.5
5. A ketogenic diet is a high-fat, low-carbohydrate diet that promotes ketosis; this has been used as an adjuvant for intractable epilepsy. However, ketosis can promote a metabolic acidosis, which can be exacerbated with the use of carbohydrate-containing solutions. Therefore, these patients should be given normal saline instead of lactated Ringer’s solution. Intraoperatively, these patients should have their acid base status and plasma glucose levels measured frequently.6
Figure 13.1. Long-term anticonvulsant therapy decreased the half-life of phenytoin and carbamazepine and their derivatives. This leads to rapid recovery of neuromuscular blockade.4
Imaging Techniques
All these patients will have an imaging study prior to surgical resection in the operating room (OR). Various imaging techniques are utilized to assess structural or functional epileptogenic zones. Magnetic resonance imaging (MRI) is the modality of choice for structural imaging and is particularly effective for focal epilepsy. That being said, no pathology is found on MRI in up to 20% of patients, necessitating other modalities.
Single photon emission computed tomography (SPECT) is the only modality able to reliably identify the ictal onset zone. In order to obtain an image, a radioactive tracer is injected during a seizure; epileptogenic areas have increased cerebral blood flow (CBF), leading to increased uptake and subsequently increased intensity on SPECT.
Positron emission tomography (PET) utilizes 18-fluorodeoxyglucose (18-FDG) as a measure of neuronal uptake of glucose, which allows the radiologist to visualize cellular metabolism; with this modality, an epileptogenic zone is indicated by a hypometabolic area. PET is best utilized during the interictal period.
Other imaging modalities include MR spectroscopy (MRS), functional MRI (fMRI), and diffusion tensor imaging (DTI). In general, the imaging modality chosen should be tailored to the clinical situation, and the number of imaging studies (and attendant general anesthetics) should be apportioned judiciously.
Magnetoencephalography (MEG) provides direct information about evoked and spontaneous neural activity; it resembles EEG, but focuses on different anatomic areas and electrochemical processes. Whereas EEG measures extracellular currents at gyral crests, MEG measures intracellular currents at fissures and sulci.
Most adults will be able to tolerate these imaging modalities without sedation. However, infants and some adolescents are uncooperative, and therefore require sedation or anesthesia in order to obtain optimal imaging. In order to take advantage of the increasingly fine resolution of the above modalities, general anesthesia is increasingly becoming the preferred technique.
Anesthesia for Diagnostic Studies
When administering a general anesthetic for the purposes of diagnostic studies, it is important to be mindful of how different medications can affect the results. Most static imaging—e.g., MRI—is not affected by the anesthetic technique, whereas dynamic studies—e.g., EEG—are significantly impacted by the choice of medication. For example, methohexital is useful for increasing the frequency and amplitude of spikes.7, 8 Mild hyperventilation can also lower the seizure threshold and improve EEG signaling during intraoperative mapping.
In the specific case of MEG, benzodiazepines drastically increase the failure rate. Propofol decreases the frequency of spikes, particularly for nonlesional epilepsy; conversely, dexmedetomidine does not produce signal artifacts, which may make it preferable to propofol for MEG.9
Surgical Resection of Seizure Foci
The goal is resection of a lesion or area of cortex that has been shown to be related to seizure generation and propagation. The most common location of seizure foci is the temporal lobe, which is involved with complex partial seizures (50% of new epilepsy cases each year) that are often related to mesial temporal sclerosis or a structural lesion of the temporal lobe. Although many epileptogenic foci are located in the region of the temporal lobe, they may be located in any area of the cerebral cortex.
A major concern for resection of seizure foci is to avoid harming brain tissue that controls vital functions such as motion, sensation, speech, and memory, referred to as eloquent cortex. Removal of this area and loss of that specific function would have a deleterious effect on neurologic outcome.
Advances in neurophysiologic monitoring (EEG and electrocorticography [ECoG]) have increased the ability to safely perform resections in functional areas of the brain. Typically low levels of anesthetic are necessary for these types of monitoring in order to map seizure foci and propagation. Sometimes, cortical stimulation of the motor cortex is performed to observe motor movement of the area of the homunculus. Here, muscle relaxants must be avoided to enable visualization of the stimulated area. Asleep craniotomy is appropriate for lesions that are not located in or deep to eloquent areas of the brain. Awake neurosurgical procedures are most important for those patients requiring interventions close to or partially overlapping eloquent brain. There are no randomized controlled trials comparing the safety or efficacy of these various techniques. Further, in the pediatric population, not all patients will tolerate awake craniotomy, and individualized patient selection is paramount. Typically, older children are better candidates, but the key is a real assessment of level of maturity and thorough preoperative discussions to ensure appropriateness.10
Awake Craniotomy
Potentially cooperative older children can assist in determination of the limits of safe cortical resection if speech and motor functions can be continually assessed intraoperatively during an awake craniotomy.
There are two basic approaches for an awake craniotomy. All procedures (line placement, local anesthesia for pinning and surgical exposure) can be done with the patient awake or with minimal sedation and the patient kept at a similar state during surgery. Alternatively, short-acting sedatives and analgesics such as propofol or fentanyl can be titrated to unconsciousness with the maintenance of spontaneous ventilation for the aforementioned procedures, after which the patient lightened to an awake or slightly sedated state for surgical resection of the seizure focus. Following surgical resection, sedatives and analgesics can be restarted or increased for patient comfort during surgical closure.
In children, with the advancement of neuromonitoring, awake craniotomy is performed less often these days.
Asleep–Awake–Asleep Technique
This technique allows the painful portions of the procedure to be performed under general anesthesia. The patient does not have to tolerate a potentially uncomfortable and claustrophobic position for a long duration as with an awake craniotomy.
Anesthesia is induced and airway control is maintained with a supraglottic device. General anesthesia is maintained for line placement, placement of head pins, and dural opening. The patient is then awakened, the supraglottic airway carefully removed, and resection of the seizure foci performed. When surgical resection has been completed, general anesthesia is again induced and the supraglottic airway reinserted for closure of the dura, skull, and skin. Following closure, the patient is awakened from general anesthesia.
There are several disadvantages to the asleep–awake–asleep approach. Airway management while the patient is in head pins can be quite difficult. Cervical spine injuries or scalp lacerations can occur during intraoperative emergence and propofol should be administered when the patient coughs or bucks while the head is immobilized in head pins. Brain swelling is a major concern as it may worsen during spontaneous ventilation from the combined vasodilatory effects of hypercapnia and volatile agents. Mannitol and furosemide can be given, but the patient then becomes very uncomfortable with sensations of thirst and urinary urgency. Hyperventilation or doxapram have been used, but the patients quickly tire of this effort. Slight elevation of the head of the bed, ensuring the neck veins are not compromised by clothing and monitors or extreme rotation, often quickly relieves the problem. If none of these maneuvers work, the patient may have to undergo general anesthesia and controlled ventilation.
With either the awake craniotomy or the asleep–awake–asleep approach, it is crucial for the anesthesiologist to have a discussion with the patient and family with respect to intraoperative needs and expectations, particularly the possibility of awareness during the surgery. It is important to communicate that not all patients are candidates for ‘‘awake’’ craniotomy.
In general, children who are younger than 10 years old or uncooperative patients of any age will likely not tolerate the awake craniotomy approach and will require general anesthesia throughout the whole procedure. In these scenarios, a variety of intraoperative electrophysiological techniques such as somatosensory evoked potentials, EEG, ECoG, and motor stimulation may be used to help localize and determine the function of the site of the planned resection.
Electrocorticography (ECoG)
ECoG is an invasive electrophysiological technique.11 It is commonly employed at 60–70% of North American centers. ECoG is obtained via an array of electrodes, which were historically rigid but are now typically flexible. Arrays of strips and grids of electrodes are useful for analysis of superficial structures, but depth electrodes can be used for analysis of deeper tissues.
The signals obtained via ECoG are large amplitude (0–50 microvolts/mm) and fall within a typical frequency range (0.5–70 Hz). The waveform varies based on electrode location, preexisting lesions, preoperative medications, and anesthetic drugs. Whereas preexisting lesions are of lower amplitude and frequency, seizures caused by anesthetic drugs are typically of higher frequency and have sharper contour. Thus, in order to localize seizure foci, it can be necessary to utilize medications to activate pathological spiking activity.
The best marker of seizure foci is interictal epileptiform activity (IEA), which can manifest as spikes, polyspikes, sharp-and-slow wave complexes, or any combination thereof. IEA usually represents the irritative zone and carries a high positive predictive value for epilepsy in otherwise neurologically normal patients. In general, a high amplitude corresponds to closer proximity to the epileptogenic focus.
Increasing depth of anesthesia changes ECoG readings in a stepwise fashion. In sequence, the obtained waves progress through alpha (8–12 Hz), beta (13–30 Hz), theta (5–7 Hz), and delta (1–4 Hz) phases, and finally burst suppression. Different medications have variable effects on waveform activity. Sedative doses of propofol and dexmedetomidine have little effect on IEAs. Although small boluses of opioids likewise have little effect, large boluses can increase interictal spikes. The halogenated volatile anesthetics tend to shift occipitally dominant alpha waves to the frontal lobe and resemble sleep spindles; nitrous oxide causes fast, frontally dominant, high-frequency activity but may suppress spikes at concentrations >50%.
If ECoG observes no spontaneous discharges, it may be necessary to activate the epileptogenic foci. The end goal is to increase frequency and distribution of spiking while being mindful of where and how different medications have an effect. All intravenous (IV) opioids activate epileptiform activity, with alfentanil being the best studied; their efficacy in this regard is attenuated by prior benzodiazepine. Although the exact mechanism is unknown, the leading hypotheses are disinhibition of gamma-aminobutyric acid-ergic (GABAergic) interneurons and inhibition of hyperpolarization-activated potassium currents.
Of the sedative hypnotic drugs, methohexital is the most commonly employed, though it may ultimately be too nonspecific. Etomidate has a high activation rate, but can also cause myoclonus and pain. Ketamine is globally active, but poorly studied. All of the inhaled agents cause activation and deterioration of spikes, and engender poorer specificity with increasing concentration. The effects of inhaled agents are exaggerated with hypocapnia.
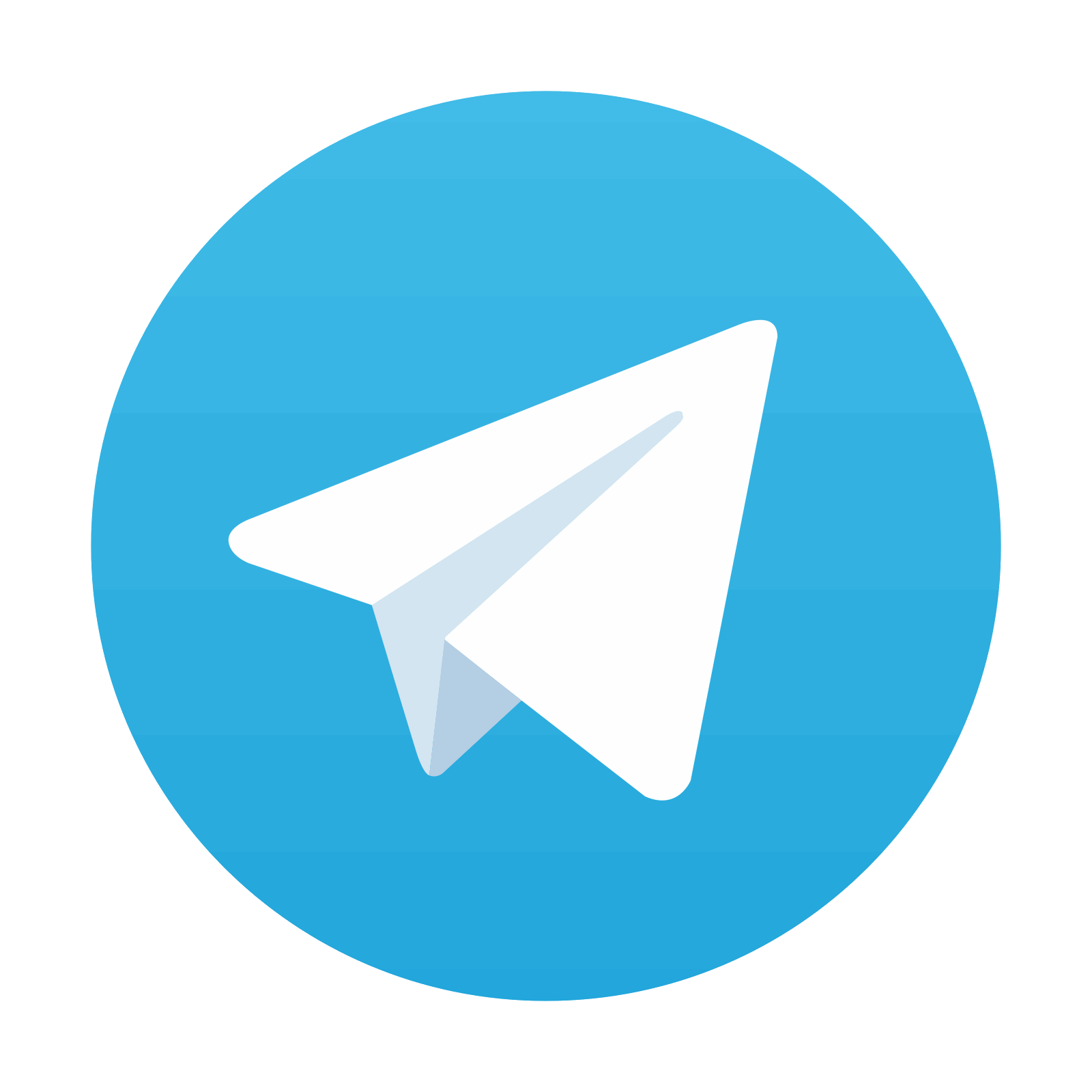
Stay updated, free articles. Join our Telegram channel

Full access? Get Clinical Tree
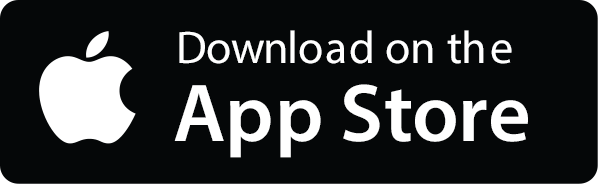
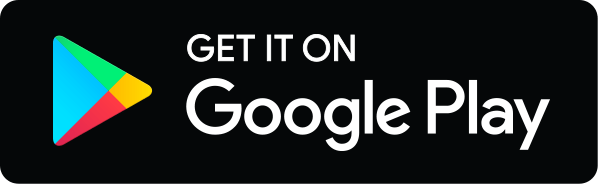