Summary
Maintaining fluid and electrolyte balance is one of the mainstays of anesthesia. Often patients present to the operating room with intravascular volume depletion secondary to nil per os (NPO) status, acute bleeding, and recent bowel preparation, as well as with electrolyte abnormalities due to coexisting disease. Physical examination is useful in determining volume status, coupled with static and dynamic hemodynamic monitoring.
Introduction
Maintaining fluid and electrolyte balance is one of the mainstays of anesthesia. Often patients present to the operating room with intravascular volume depletion secondary to nil per os (NPO) status, acute bleeding, and recent bowel preparation, as well as with electrolyte abnormalities due to coexisting disease. Physical examination is useful in determining volume status, coupled with static and dynamic hemodynamic monitoring.
Evaluation of Intravascular Volume
Determining the intravascular volume status can be difficult for the clinician. However, it is very important to be able to assess intravascular volume derangements in order to improve perfusion to vital organs. Patient history is the first step in evaluating volume status by assessing the NPO status and for the presence of persistent diarrhea and vomiting, intravenous fluid administration, recent blood administration, nasogastric suctioning, and hemodialysis. Physical examination further assists in assessing the volume status by assessing capillary refill time, skin turgor, heart rate, blood pressure, and intake and output and for the presence of jugular distension, as well as the presence of peripheral edema. Laboratory analysis can also provide some insight with serum lactate levels, as well as arterial blood gases, both of which will assess end-organ perfusion, which can be used as a surrogate for volume status.
Physical Examination and Laboratory Markers for Fluid Status
Hypovolemic patients may present with lethargy, hypotension, decreased skin turgor, and reduced jugular venous pressure. Laboratory values also display elevated blood urea nitrogen and serum creatinine levels, hyperkalemia or hypokalemia, hypernatremia or hyponatremia, and metabolic alkalosis as well as acidosis.
Hypovolemia is one of the most important volume derangements and can lead to perioperative complications, including acute kidney injury, acute cerebrovascular accidents, and decreased global tissue perfusion. This loss of intravascular volume may be due to “third spacing,” as well as loss of sodium-containing fluids through the skin, gastrointestinal (GI) tract, or urine. The decreased intravascular volume can have many causes, including excessive preoperative fasting, mechanical bowel preparation, acute and chronic GI bleeding, sympathetic blockade secondary to neuraxial anesthesia, and myocardial depression and vasodilatation secondary to inhaled anesthetics.
Hypervolemic patients may present with elevated jugular venous pressure, edema of the lower extremities, S3 heart sound, and orthopnea. Laboratory findings include elevated B-type natriuretic peptide (BNP) and N-terminal pro-BNP (NT-proBNP) levels, in addition to radiographic findings of Kerlyy “B” lines due to increased pulmonary vascular and interstitial markings.
Hemodynamic Measurements
Static hemodynamic parameters that can be used to monitor intravascular fluid status include noninvasive blood pressure, heart rate, urine output, mixed venous oxygen saturation, and central venous pressure. These parameters are best used based on their trends to determine volume status. These parameters have many limitations, including in a hypovolemic patient unable to increase their heart rate secondary to beta-blocker use, as well as surgical stress and anesthetic gases leading to decreased urine output in a euvolemic patient.
Dynamic hemodynamic parameters are typically used as a guide for goal-directed fluid therapy, and compared to static parameters, they provide improved assessment of fluid challenge responsiveness. These parameters include arterial pressure waveform variations (stroke volume variation, pulse pressure variation, systolic blood pressure variation), transesophageal echocardiography (TEE), and serum lactate levels, as well as noninvasive devices (Vigileo FloTrac).
Intravenous Fluids
The two types of intravenous fluids include crystalloids and colloids. Crystalloid solutions consist of ions in water that are able to freely cross capillary membranes. Colloids consist of high-molecular-weight substances that cannot cross capillary membranes. Colloid solutions are beneficial in maintaining plasma oncotic pressure, whereas crystalloid solutions are able to equilibrate rapidly with the extravascular fluid space. There is no ideal resuscitation fluid; however, the choice of fluid, as well as the dose, may affect patient-centered outcomes. Unfortunately, systematic reviews, as well as randomized controlled trials, have not been able to prove increased safety or effectiveness of any one resuscitation fluid or shown a decrease in mortality.
Crystalloids
These solutions are widely available and low cost, and are typically the first-line resuscitation fluid for septic shock, hemorrhagic shock, burn injury, cardiac arrest, and brain trauma. Isotonic crystalloids can be separated into two types: balanced (lactated Ringer’s, Plasma-Lyte) and saline (0.9% sodium chloride). Balanced solutions have a composition that more closely resembles that of extracellular fluid. This is achieved by including buffers that are excreted, as well as metabolized. They also contain sodium, chloride, and possibly potassium, as well as have a more similar composition of acids and bases to extracellular fluid, as shown in Table 12.1.
Table 12.1 Crystalloid solutions and their composition
Electrolyte | Plasma (mEq L−1) | ICF (mEq L−1) | ECF (mEq L−1) |
---|---|---|---|
Sodium | 142 | 10 | 140 |
Potassium | 4 | 150 | 4.5 |
Magnesium | 2 | 40 | 2 |
Calcium | 5 | 1 | 5 |
Chloride | 103 | 103 | 117 |
Bicarbonate | 25 | 7 | 28 |
ICF, intracellular fluid; ECF, extracellular fluid.
Normal saline contains solely sodium and chloride, with a chloride concentration of 154 mEq L−1 that is 50% more than is contained in extracellular fluid. This can lead to dilutional hyperchloremic acidosis after large volume administration due to its increased sodium and chloride content. As chloride concentration increases, a decrease in plasma bicarbonate concentration is also observed.
Balanced crystalloid solutions include lactated Ringer’s, Plasma-Lyte, Normosol, and Isolyte. These solutions also contain sodium and chloride, but also have varying mixtures of added electrolytes (potassium, magnesium, and calcium) and buffers (lactate and gluconate). The addition of these electrolytes and buffers allows these fluids to have a more similar acid–base composition to extracellular fluid. There is long-standing debate regarding the effectiveness of balanced crystalloids versus saline for fluid replacement. Recent studies have indicated that balanced crystalloids can decrease the incidence of death, persistent renal dysfunction, and new renal replacement therapy.
Colloids
These solutions are higher cost and contain higher-molecular-weight molecules in a carrier fluid that are typically unable to pass through the semipermeable capillary membrane. This allows colloids to maintain and expand intravascular volume as they are less able to leave the intravascular space and instead maintain colloid oncotic pressure. This leads to a decreased volume required for resuscitation, also known as the volume-sparing effect. When resuscitating with colloids versus crystalloids, three times the amount of crystalloids are needed versus colloids to replace the lost intravascular volume. The two main types of colloids include human derived (albumin) and semisynthetic (starches).
Albumin consists of human protein removed from blood and added to saline. It is then heated in order to decrease the risk of viral transmission. Administration increases the intravascular oncotic pressure, which then leads to movement of fluid from the extravascular compartments into the intravascular compartments. In severe sepsis and critically ill patients, crystalloid solutions should be used for initial management, with albumin infusions considered if solely crystalloids are ineffectual, as per the Surviving Sepsis Campaign guidelines.
Semisynthetic colloids include hydroxyethyl starch solutions and dextran solutions, as well as others. These solutions are currently not in use in the United States but have found use in Europe, and as a first-line resuscitation fluid in military theaters. Current evidence is poor regarding clinical benefit, and risks of use include possible nephrotoxicity and changes in coagulation.
Perioperative Fluid Replacement
Preexisting fluid deficits, fluid and blood losses during surgery, and patient comorbidities can all increase the complexity of proper fluid replacement in the perioperative patient. Historically, a more liberal approach to intravenous fluid therapy was employed in order to replace fluid lost to “third spacing.” Loss of fluid into the third space leads to fluid accumulation in the extravascular extracellular space. More recently, consensus has shifted towards a more goal-directed approach to fluid replacement therapy due to patients receiving excessive amounts of fluids in the perioperative period. The objective is to avoid underresuscitation, as well as overresuscitation, in the perioperative period. This is important as underresuscitaiton can lead to hypotension and impaired tissue oxygenation, as well as impaired perfusion. Overresuscitation may lead to anastomotic compromise and impaired GI function, as well as cardiac and pulmonary complications. Ultimately, fluid replacement should be based on the individual patient, their comorbidities, and surgical considerations.
Conventional Management
This management style consisted of replacing fluid with crystalloid by using the maintenance fluid rate multiplied by the duration of NPO, with the addition of insensible losses (6 mL/(kg hr) for small incisions), as well as replacement of three times the amount of blood loss with crystalloid. The addition of maintenance fluid is also based on the 4–2–1 rule (4 mL kg−1 for the first 10 kg body weight, 2 mL kg−1 for the second 10 kg body weight, and 1 mL kg−1 for the remaining body weight). This method of fluid replacement can lead to a large volume of fluids administered in the perioperative period, and has started to lose favor for more goal-directed fluid management strategies.
Goal-Directed Fluid Therapy
This management style consists of administering intravenous fluid to achieve a specific target. These targets include maintaining stroke volume, cardiac index, urine output, blood pressure, and heart rate. This is achieved by delivering small boluses of colloid (250 mL) in an effort to maintain cardiac output or stroke volume, rather than relying on a maintenance rate of fluid replacement. Use of goal-directed fluid therapy in high-risk patient populations and major surgeries was found to result in improved time to recovery of GI function and decreased length of stay, as well as decreased rates of pneumonia.
Perioperative Fluid Loss
One of the difficult tasks encountered during surgery is estimation of surgical blood loss. This can be complicated by hidden blood loss in the drapes, surgical flush use, and occult bleeding into the surgical wound. Visual estimation is used to determine the amount of blood in the surgical suction canister, as well as assessing the laparotomy pads and surgical sponges. Completely saturated laparotomy pads can contain 100 mL of blood, and surgical sponges can carry as much as 10 mL of blood.
Other fluid losses also occur during surgical procedures. Based on the size of the incision, evaporative losses can be calculated to further track fluid loss. Small incisions, such as hernia repairs, may lead to 0–2 mL kg−1 of volume loss per hour, moderate incisions up to 2–4 mL/(kg hr), and large incisions, such as open abdominal procedures, up to 4–8 mL/(kg hr).
Electrolyte Balance Management
Table 12.2 shows the normal electrolyte composition in body compartments.
Sodium Homeostasis
Sodium homeostasis and plasma sodium concentration are generally reflective of total body osmolality. Table 12.2 above demonstrates the normal electrolyte composition in body compartments. Total body osmolality is the sum of extracellular and intracellular solutes divided by total body water. Plasma osmolality is regulated by thirst and secretion of antidiuretic hormone (ADH) from the posterior pituitary. When an increase in extracellular osmolality is sensed by cells in the hypothalamus, ADH is released and increases water reabsorption in the kidney to restore normal plasma osmolality. Normal plasma osmolality is typically in the range of 280–290 mOsm L−1. On the other hand, when the extracellular fluid has a decreased osmolality, ADH secretion is reduced; the kidneys excrete more water, and plasma osmolality shifts back to normal. Thirst sensors in the hypothalamus also sense increases or decreases in extracellular fluid osmolality and stimulate or suppress thirst. One must evaluate plasma osmolality when assessing a patient with sodium level derangements.
Table 12.2 Normal electrolyte composition in body compartments
Electrolyte | Plasma (mEq L−1) | ICF (mEq L−1) | ECF (mEq L−1) |
---|---|---|---|
Sodium | 142 | 10 | 140 |
Potassium | 4 | 150 | 4.5 |
Magnesium | 2 | 40 | 2 |
Calcium | 5 | 1 | 5 |
Chloride | 103 | 103 | 117 |
Bicarbonate | 25 | 7 | 28 |
ICF, intracellular fluid; ECF, extracellular fluid.
Hyponatremia
Hyponatremia is defined as sodium concentration <135 mEq L−1 and occurs either due to an increase in total body water or through the body’s excess sodium loss relative to water loss. Hyponatremia causes are often determined by evaluation of plasma osmolality. In a hyponatremic patient with a normal plasma osmolality, causes include marked hyperlipidemia or hyperproteinemia which are asymptomatic. It can also be due to glycine absorption during transurethral surgery that presents with symptoms. In those with an elevated plasma osmolality, causes of hyponatremia are attributed to either mannitol administration or hyperglycemia. Hyperglycemia causes what is known as pseudohyponatremia. Calculations can be made to determine the actual sodium level in the presence of severely elevated plasma glucose levels. Patients with both low sodium and low plasma osmolality are categorized as having decreased, normal, or increased total sodium content. In those with a decreased total sodium content, sodium losses can be renal or extrarenal. Renal losses are most commonly attributed to thiazide diuretics but can also be due to salt-losing nephropathies, mineralocorticoid deficiency, or renal tubular acidosis. Extrarenal sodium losses are the result of excessive diarrhea or vomiting, sweating, burns, or “third spacing.” In patients with a normal total sodium content, causes include primary polydipsia, syndrome of inappropriate ADH secretion (SIADH), hypothyroidism, drug-induced, and glucocorticoid deficiency. Finally, in those with an increased total sodium content and hyponatremia, causes typically include congestive heart failure, cirrhosis of the liver, and nephrotic syndromes.
Symptoms and manifestations of hyponatremia are due to an increase in intracellular water. With this effect, symptoms are typically neurological and nonspecific. Some symptoms include malaise, weakness, and anorexia; however, they can progress to life-threatening situations such as seizures or coma and leading up to death. Serious symptoms typically occur when sodium levels are <120 mEq L−1. Chronicity of sodium level derangements also factors into the severity of symptomatology. Those with chronic hyponatremia typically have mild symptoms or none at all.
When correcting for hyponatremia, one must first aim to identify the underlying cause of the metabolic derangement. This is often done through plasma and urine osmolality measurements, along with evaluation of the patient’s volume status. Urine sodium levels are measured to evaluate whether sodium losses are extrarenal or renal in nature. The treatment of choice of hyponatremic patients with a decreased total body sodium content is typically isotonic saline. By contrast, in patients with normal or increased total body sodium content, treatment for hyponatremia is water restriction. Evaluation of a patient’s underlying cause of hyponatremia is particularly important because treatment may be more specific to their disease process such as hormone replacement in a patient with, for example, a glucocorticoid or thyroid hormone deficiency. Since acute hyponatremia presents with the most serious of symptoms, it is generally recommended to treat patients to a sodium level of >120 mEq L−1. Correction of sodium levels must be performed first by calculating the patient’s total sodium deficit by use of the following formula: sodium deficit = total body water × (desired sodium − current sodium). Once this calculation has been determined, the sodium level should not be rapidly corrected due to the possibility of causing central pontine myelinolysis and permanent neurological damage. Acute hyponatremia, typically described as <48 hours, often presents with seizures and/or coma and must be addressed urgently. Several published cases suggest a correction of 4–6 mEq L−1 should be sufficient to suppress seizures secondary to hyponatremia. Fluid boluses of 3% saline are typically used to treat acute hyponatremia. One method for correcting sodium levels is to use an approximation formula of 1 mL kg−1 of 3% sodium chloride, which is expected to raise serum sodium levels by 1 mEq L−1. One must always remember to address the underlying cause to evaluate if it is reversible. It is also recommended to monitor serum sodium levels approximately every 6–8 hours during correction periods. Typically, sodium levels of >130 are acceptable for a patient who will undergo general anesthesia. Those with symptoms should have the sodium levels corrected prior to anesthetic administration, especially those undergoing transurethral procedures and at risk of massive fluid absorption.
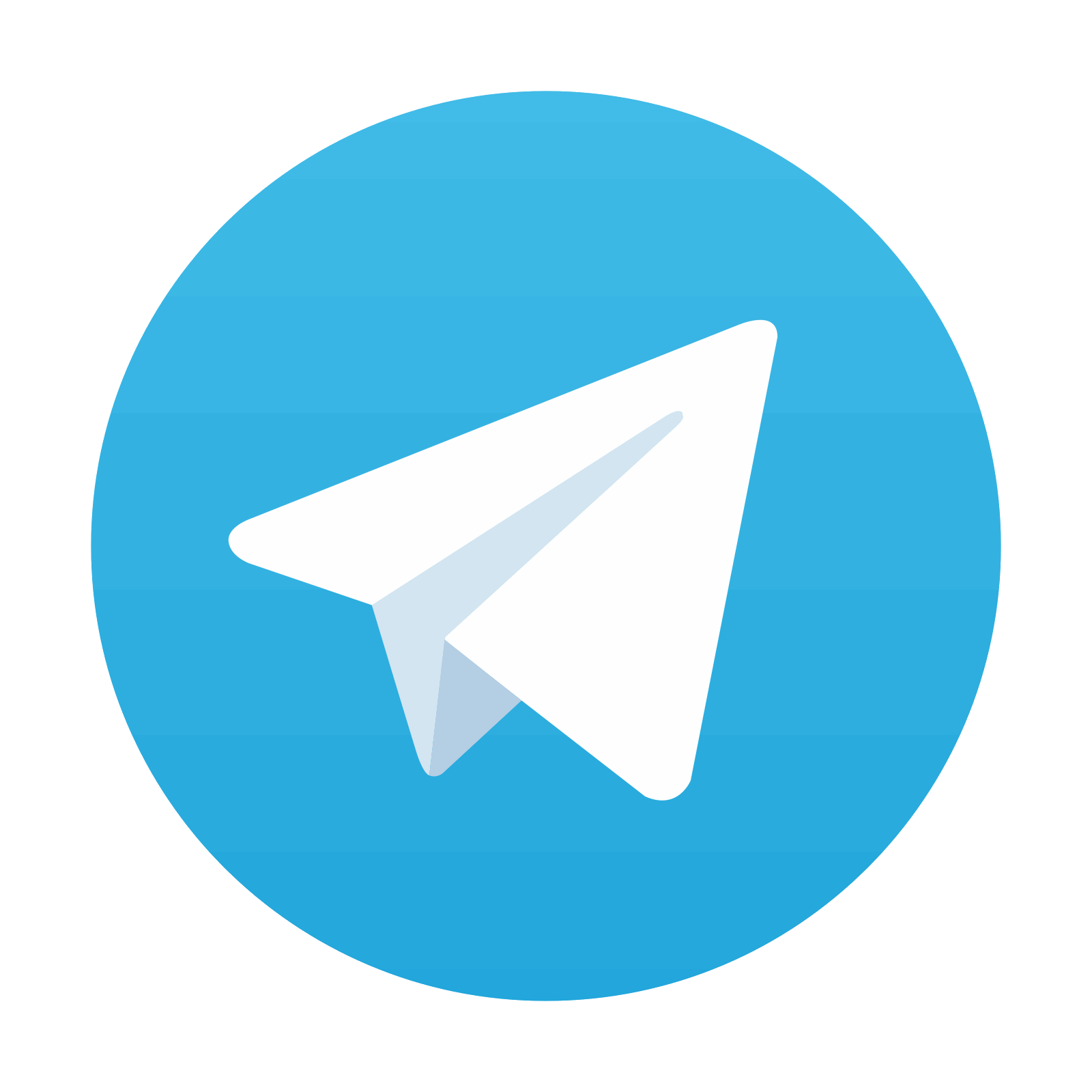
Stay updated, free articles. Join our Telegram channel

Full access? Get Clinical Tree
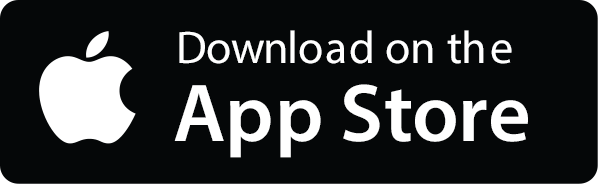
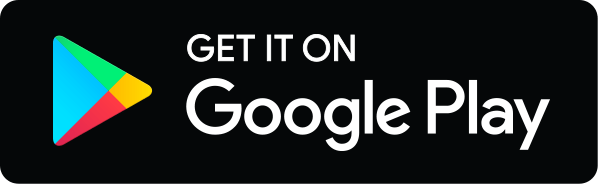
