Introduction
Discovery and use of muscle relaxants in anesthesia practice dates back to 1942, when Griffith and Johnson used d-tubocurarine to provide muscle relaxation during surgery. Since then, a number of muscle relaxants have been discovered, and their use has not only revolutionized the practice of anesthesia but also made possible the development of cardiothoracic, neurologic, and organ transplant surgeries. Muscle relaxants can be categorized into different groups, based on their chemical structure, site of action, and their duration of action.
History
Curare (also known as d-tubocurare), prototype of muscle relaxant used in anesthesia practice, was discovered in South America and was used for hunting. Its use in anesthesia was first described in 1940. In 1954, Beecher and Todd reported increased mortality with use of muscle relaxants. This was probably because of inadequate knowledge of pharmacology of muscle relaxants, suboptimal use of artificial ventilation, and unavailability of reversal agents and neuromuscular monitoring. With growing knowledge of pharmacology of muscle relaxants, reversal agents and advancement in neuromuscular monitoring has improved safety profile of these agents in the modern era of anesthesia practice.
1942: Griffith and Johnson described d-tubocurarine.
1952: Thesleff and Foldes introduced succinylcholine (SCh), the first used clinically by Bovet (for which he got Nobel prize).
1967: Baird and Reid used the first synthetic aminosteroid, pancuronium.
Classification
There are three types of neuromuscular blocking agents:
-
Blockers of neuromuscular junction (Table 10.1).
Abbreviation: NM, neuromuscular.Notes: a Neuromuscular blocking agents (intermediate acting) are most commonly used muscle relaxants in anesthesia practice.
Neuromuscular Physiology and Mechanism of Action of Neuromuscular Blocking Agents
It is imperative to have the knowledge of transmission of signal across neuromuscular junction, and the events occurring during transmission, in order to understand the mechanism through which muscle relaxants act.
During neuromuscular transmission, as soon as an action potential reaches presynaptic nerve terminal, it leads to opening of voltage-gated calcium channels. Calcium enters the presynaptic nerve terminal and binds to acetylcholine (ACh) vesicles. Calcium-bound ACh vesicles move near the presynaptic membrane, bind to it and release ACh into synapse. ACh binds to ACh receptors on postsynaptic muscle end plate and causes opening of Na channel. Opening of Na channel results in depolarization of muscle end plate.
SCh (depolarizing muscle relaxant) acts as an agonist at ACh receptor and causes depolarization of muscle membrane, while nondepolarizing muscle relaxants act as antagonists at ACh receptor and compete with ACh for binding sites (Table 10.2).
ACh receptors at neuromuscular junction are of nicotinic type (Table 10.3). It is a ligand-gated ion channel composed of five subunits: Two α and single β, δ, and ε subunits, which are all arranged around a central pore. When a molecule of ACh binds to each of the α subunits, the receptor undergoes conformational change, which results in opening of the central pore. In case of nondepolarizing muscle relaxant, if one molecule binds to α subunit; two agonist molecules cannot bind, and neuromuscular transmission is inhibited. This pore is a nonspecific ion channel, through with Na+, K+ and Ca2+ ions can flow, causing miniature end-plate potentials. When the threshold level of depolarization is reached, voltage-gated Na+ channels open and the action potential is propagated across the muscle.
Note: Both fetal and α7 type ACh receptors appear in burs and during immobilization.
Sequence of Blockade
Central muscles (facial muscles, pharynx, larynx, diaphragm, abdominal muscles) are blocked earlier than peripheral muscles. Recovery follows the an identical pattern. Central muscles recover faster than peripheral muscles.
Factors responsible for it are:
Diaphragm in isolation is most resistant muscle to block and recovers the fastest. Respiratory muscles more resistant than muscles of upper airway. So, if extubated without proper recovery of these muscles, upper airway obstruction may occur.
Monitoring Neuromuscular Blockade
Adductor pollicis muscle is commonly used to monitor neuromuscular blockade. Orbicularis oculi can also be used and is better indicator.
Individual Neuromuscular Blocking Drugs
Succinylcholine
Physicochemical properties: Chemically, it is dicholine ester of succinic acid, that is, 2 ACh molecules linked back-to-back through acetate methyl group. It is stored at 4 degrees to prevent hydrolysis.
Dose: 1 to 2 mg/kg used to provide ideal intubating conditions for rapid sequence intubation and induction.
Onset of action: 30 to 60 seconds; duration of action: 3 to 9 minutes.
Metabolism: Hydrolyzed by butyrylcholinesterase (pseudocholinesterase) in plasma to succinylmonocholine and choline and ultimately to succinic acid and choline. Butyrylcholine esterase is present in plasma and not in neuromuscular junction. Only 10% of administered drug reaches the site of action, due to metabolism by butyrlcholinesterase; so it should be given at a faster rate. The plasma levels of butyrlcholinesterase can alter the duration of action of SCh, with decreased levels prolong the duration of action.
In addition to SCh, mivacurium is also metabolized by butyrylcholinesterase. Therefore, caution should pe paid while using both these agents in conditions listed in Box 10.1.

Full access? Get Clinical Tree
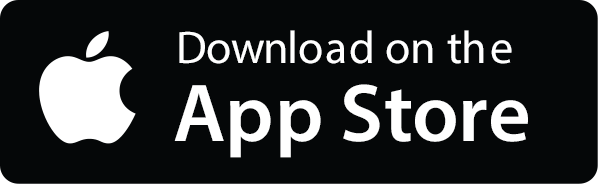
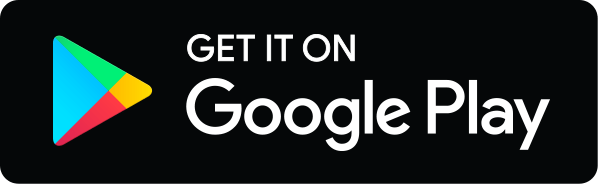