Abstract
Head trauma is the most common cause of death and permanent disability following trauma. Blunt head injury is usually the result of motor vehicle collisions, auto versus pedestrian collisions, or falls from significant heights. Gunshot wounds cause the vast majority of penetrating head injuries, although stab wounds and impalement injuries may also be seen.
Introduction
Head trauma is the most common cause of death and permanent disability following trauma. Blunt head injury is usually the result of motor vehicle collisions, auto versus pedestrian collisions, or falls from significant heights. Gunshot wounds cause the vast majority of penetrating head injuries, although stab wounds and impalement injuries may also be seen.
Clinical Examination
Head injury is classified into mild, moderate, and severe categories, depending on the patient’s Glasgow Coma Scale (GCS) at the time of presentation.
Patients with mild head injury have a GCS of 14–15, those with moderate head injury have a GCS of 9–13, and those with severe head injury have a GCS of <9. The GCS is a physiologic score and does not provide information about the anatomic nature of the injury. It can be affected by hypotension, hypoxia, hypoglycemia, medications, and alcohol and illicit drug use. The anatomic severity of head trauma is described by the head Abbreviated Injury Score (AIS), which is based on the computed tomography (CT) scan, magnetic resonance imaging (MRI), and operative findings. A head AIS of 1 or 2 indicates minor brain trauma, an AIS of 3 indicates moderate trauma, an AIS of 4 or 5 denotes severe trauma, and an AIS of 6 describes a nonsurvivable injury.
Following the primary and secondary survey according to Advanced Trauma Life Support (ATLS) protocols, a more specific head injury evaluation should be performed. The head is inspected for signs of trauma, including lacerations, hematomas, areas of skull depression, raccoon eyes, Battle’s sign, hemotympanum, rhinorrhea, and otorrhea. The pupils are examined for symmetry and reaction to light, the extraocular movements and other cranial nerve functions are assessed, and motor and sensory function is assessed for symmetry or deficiencies.
Patients with brain stem herniation present with depressed levels of consciousness; dilatation of the ipsilateral pupil, due to compression of the third cranial nerve (in the early stages, there may be transient pupil constriction due to stimulation of the nerve); contralateral motor posturing (decorticate, followed by decerebrate and finally flaccid paralysis); bradycardia; elevated blood pressure; and irregular respiration.
Investigations
All patients with a GCS of <14, or patients with a GCS of 15 with a history of loss of consciousness, amnesia, headache, posttraumatic seizure, scalp hematoma, signs suspicious of fracture of the base of the skull (ecchymoses around the eyes or behind the ears, ear or nose bleeding, or cerebrospinal fluid leakage), significant mechanism, advanced age, or history of anticoagulation, should undergo noncontrast CT scan evaluation. Selected patients may require further investigation by CT angiography (CTA), MRI, or rarely cerebral angiography. The ability to perform 3-D or multiplanar reconstructions may be particularly helpful in the diagnosis of injuries like basilar skull fractures.
Multitrauma patients may have injuries to the chest or abdomen that require immediate operative intervention for bleeding control, which takes priority over head-injury evaluation. In these cases, the CT scan of the head may be deferred until after the hemorrhage control.
CTA should be performed in suspected vascular injuries, such as in the presence of fractures of the vertebral canal or the carotid foramen, or in neck hematomas following blunt trauma, such as seat-belt marks.
Skull X-rays are seldom indicated because CT scan is more sensitive for identifying skull fractures and provides more information regarding underlying brain injury. If CT scan is not available, skull X-rays may be useful for identifying foreign bodies (e.g., bullet fragments) or intracranial air.
General Management
The main goal of cerebral resuscitation in the emergency department is to prevent secondary brain damage, by preventing or correcting any hypoxia, hypotension, seizures, hyperthermia, and hypercarbia. Cerebral resuscitation is performed in the context of overall resuscitation and is guided by ATLS principles. Securing an airway and ensuring adequate oxygenation and ventilation are the highest priorities. A brief neurologic examination should be performed prior to using paralytic drugs for rapid sequence intubation (RSI). Patients with a GCS <9 should be evaluated for intubation, and selected patients with a higher GCS may also need intubation for airway protection.
The cerebral perfusion pressure (CPP) is defined as the mean arterial pressure (MAP) minus the intracranial pressure (ICP) and is a reflection of the blood flow and oxygen delivery to the brain. Maintaining adequate CPP (CPP >70 mmHg in adults, CPP >50 mmHg in young children) is important in the face of increased ICP to prevent secondary ischemic injury.
Consequently, measures to decrease ICP while maintaining adequate systemic blood pressure with resuscitation and hemorrhage control are essential.
For patients with evidence of increased ICP or clinical signs of actual or impending herniation, immediate measures to decrease ICP are indicated. The patient should be adequately sedated and, if necessary, pharmacologically paralyzed. Hyperventilation to reduce pCO2 to a level of 32–35 mmHg may temporarily decrease intracranial pressure. Mannitol administration (0.5–1.0 g/kg over 20 minutes) may be considered in hemodynamically stable patients with no suspicion of significant extracranial bleeding. In cases where potential hypotension and hemorrhage are of concern, mannitol should be avoided and consideration should be given to the use of hypertonic saline (3% NaCl, 250 ml). The neck should be cleared of any binding or restricting devices or ties to improve venous drainage from the head. Positioning the head at 30 degrees elevation can decrease ICP once the spine has been cleared. If the patient has spinal precautions, head-of-bed elevation can be achieved with reverse Trendelenburg positioning. The placement of a ventriculostomy to drain cerebrospinal fluid (CSF) is often effective for treating elevated ICP. In refractory cases, barbiturate coma may be used to decrease cerebral metabolic demand. In extreme cases, a decompressive craniectomy or even lobectomy may be considered.
Hyperthermia should be avoided, as this significantly increases cerebral metabolism and oxygen demand. Seizure prophylaxis (phenytoin loading dose 10–15 mg/kg over 30–60 minutes, followed by 5 mg/kg per day or levetiracetam 500 mg twice a day for 7 days) in all patients with intracranial hemorrhage is recommended.
Definitive treatment for head injury depends on the nature of the lesion. Closed skull fractures require no specific treatment, but open fractures should be irrigated, debrided, and closed. Depressed skull fractures require elevation of the fragment if the displacement is greater than one bone width, along with debridement if the wound is grossly contaminated. Basilar skull fractures usually heal uneventfully, but patients with rhinorrhea or otorrhea require precautions and careful follow-up to ensure that the fistula closes. Most CSF leaks stop within two weeks, but persistent leaks may require a formal dural closure.
Most intracranial injuries can be managed nonoperatively, with supportive measures. Smaller lesions are usually managed with observation and repeat CT scan, usually after about six hours or earlier if the patient deteriorates.
As a general guideline, adults with epidural hematomas (EDH) diameter >15 mm, subdural hematomas (SDH) >10 mmHg, or a midline shift >5 mm require a craniectomy. Subarachnoid hemorrhage (SAH) is treated nonoperatively and in severe cases with nimodipine (calcium channel blocker) to decrease surrounding vasospasm. Intraventricular hemorrhage (IVH) may require ventriculostomy to remove blood and CSF, but the prognosis usually remains poor.
ICP monitoring should be considered in patients with a GCS <9 plus an intracranial pathology on CT scan or in patients with a GCS <9 and normal CT scan, if two or more of the following are present: (a) age >40, (b) BP <90, and (c) posturing. In addition to the diagnostic value, an ICP catheter may be used for therapeutic purposes, to drain CSF and lower the intracranial pressure.
Tips and Pitfalls
Some patients are at a higher risk for intracranial hemorrhage after relatively minor mechanisms of injury. These include the elderly, chronic alcoholics, infants, patients with cerebral atrophy, and patients on antiplatelet or anticoagulant medications. A low threshold for obtaining a head CT scan should be maintained in these patients.
Pediatric patients with a GCS of 15 and scalp hematoma should be evaluated by CT scan or observed for at least six hours because of the risk of EDH.
Altered mental status, seizures, and focal neurologic deficits should not be ascribed to intoxication, dementia, or other chronic conditions if there is a history or evidence of head trauma present.
Excessive hyperventilation (pCO2 <30 mmHg) should be avoided because it results in cerebral vasospasm and decreased brain perfusion.
Subacute SDH may appear isodense with the surrounding brain 5–10 days after the injury. Altering the density values of the CT or using contrast will demonstrate the lesion.
Coagulopathy is common with serious head injury and may result in more severe bleeding, hemorrhage from other noncerebral sites, and dysregulation of the coagulation cascade. A baseline coagulation profile should be obtained in all patients with serious head injury and repeated periodically during admission. Appropriate blood products and reversal agents should be administered early.
Child abuse should be suspected in cases of intracerebral injury or skull fracture in infants and children.
Scalp Injuries
The scalp is composed of five layers: skin, dense connective tissue, loose connective tissue, aponeurosis, and epicranium. The vessels and nerves are located in the dense connective tissue. Scalp lacerations can bleed profusely because of the rich vascularity and the position of the vessels in the dense connective tissue, which limits retraction and constriction when bleeding. It is important to suture or staple any scalp laceration and apply a compression dressing as soon as possible following the primary survey. Injection of local anesthetic should be done in the dense connective tissue and should be met with significant resistance. If there is no resistance during injection, most likely the anesthetic is infused into the loose connective tissue layer, which does not contain any nerves, and it will not be effective. Scalp lacerations usually heal well and rarely get infected because of the rich blood supply (Figure 1.1).
Skull Fractures
Skull fractures are often associated with intracranial injury, and specific locations, such as those across the middle meningeal arterial groove or the dural sinuses, can be of particularly high risk for EDH. CT scan has largely replaced plain radiographs of the skull for diagnosis due to the high sensitivity of CT scan for detection of fractures. The incidence of brain injury in the setting of a skull fracture is high and occurs in about one-third of the patients. Skull fractures in infants are commonly due to child abuse, and the circumstances surrounding the injury need to be carefully investigated.
As with fractures of other boney structures, skull fractures are classified as simple or comminuted, displaced or nondisplaced, and open or closed. Linear fractures are the most common, accounting for approximately 80% of all skull fractures, and generally result from lower-energy blunt trauma over a large surface area. In contrast, comminuted fractures contain multiple fracture lines and generally require a larger amount of force to create compared to linear fractures. Displaced fractures are the result of a very-high-energy direct blow to a small surface area. Patients with depressed skull fractures have an increased incidence of posttraumatic seizures because the impact of the fragments on the cortex of the brain results in areas of trauma that may ultimately become seizure foci. Consequently, seizure prophylaxis is begun on admission and continued for one week. The management of closed depressed skull fractures depends on the degree of depression. Conservative treatment is usually recommended for closed fractures with minor depression; however, surgical elevation of the fragments is usually recommended if the depression is greater than one bone width (from inner to outer table) (Figure 1.2 AC, Figure 1.3 A,B, Figure 1.4, Figure 1.5 AD).
Figure 1.5 A–D Depressed skull fractures. Lateral radiograph of the skull showing hyperdensity due to overlapping bone fragments (arrow) at the apical–parietal skull (A). CT scan showing right temporoparietal comminuted depressed fracture (arrow) and associated soft tissue swelling. This patient suffered a concomitant EDH (B). CT scan showing depressed fracture of the left parietal bone (large arrow) with underlying EDH (small arrow; C). CT scan showing 3-D reconstruction of a skull with left parietal depressed comminuted skull fracture (arrow; D).
Open fractures that communicate with the skin or mucus membranes may result in the direct introduction of contaminants such as hair, skin, or foreign debris into the cranial vault. Pneumocephalus (air within the confines of the skull) rarely results in mass effect and displacement of intracranial contents. More commonly, its significance is the presence of a direct communication between the brain and the extracranial environment, which may lead to the development of meningitis, osteomyelitis, or, more commonly, brain abscess. Consequently, open skull fractures must be carefully debrided, irrigated, and closed to prevent such complications. Prophylactic antibiotics are indicated for the treatment of open skull fractures (Figure 1.6).
Isolated skull fractures may result in headache and local tenderness. Physical examination of the skull may reveal a depression in the skull, but more commonly, the fracture is not palpable because of overlying soft-tissue swelling.
Basilar Skull Fracture
The skull base is divided into three compartments (anterior, middle and posterior fossa), and the clinical presentation of a basilar fracture varies depending on location. Anterobasilar fractures may present with raccoon eyes (hematomas around the eyes), rhinorrhea, and anosmia; middle fossa fractures may present with hemotympanum, otorrhea, vertigo, or Battle’s sign (ecchymosis of the skin behind the ear); posterior fossa fractures may present with ataxia and nystagmus. Raccoon eyes and Battle’s sign may develop over time, despite being absent on initial presentation (Figure 1.7 A,B, Figure 1.8 AC).
Figure 1.7 A,B Physical examination findings indicative of basilar skull fracture of the anterior cranial fossa. Patient with bilateral periorbital ecchymosis (raccoon eyes), due to fracture of the base of the anterior skull fossa (A). Bilateral raccoon eyes and massive epistaxis in a patient with severe basilar skull fracture (B).
Figure 1.8 A–C Physical exam findings indicative of basilar skull fracture of the middle cranial fossa. Battle’s sign – ecchymosis over the mastoid process, indicating basilar fracture involving the middle cranial fossa (A). Bloody otorrhea following basilar skull fracture of the middle cranial fossa with rupture of the tympanic membrane (B). Bloody otorrhea, reveals the “halo effect” or “double ring sign” (arrow) of CSF separating from blood when applied to the sheet (C). This sign can also be seen when the bloody drainage is applied to a paper towel or filter paper.
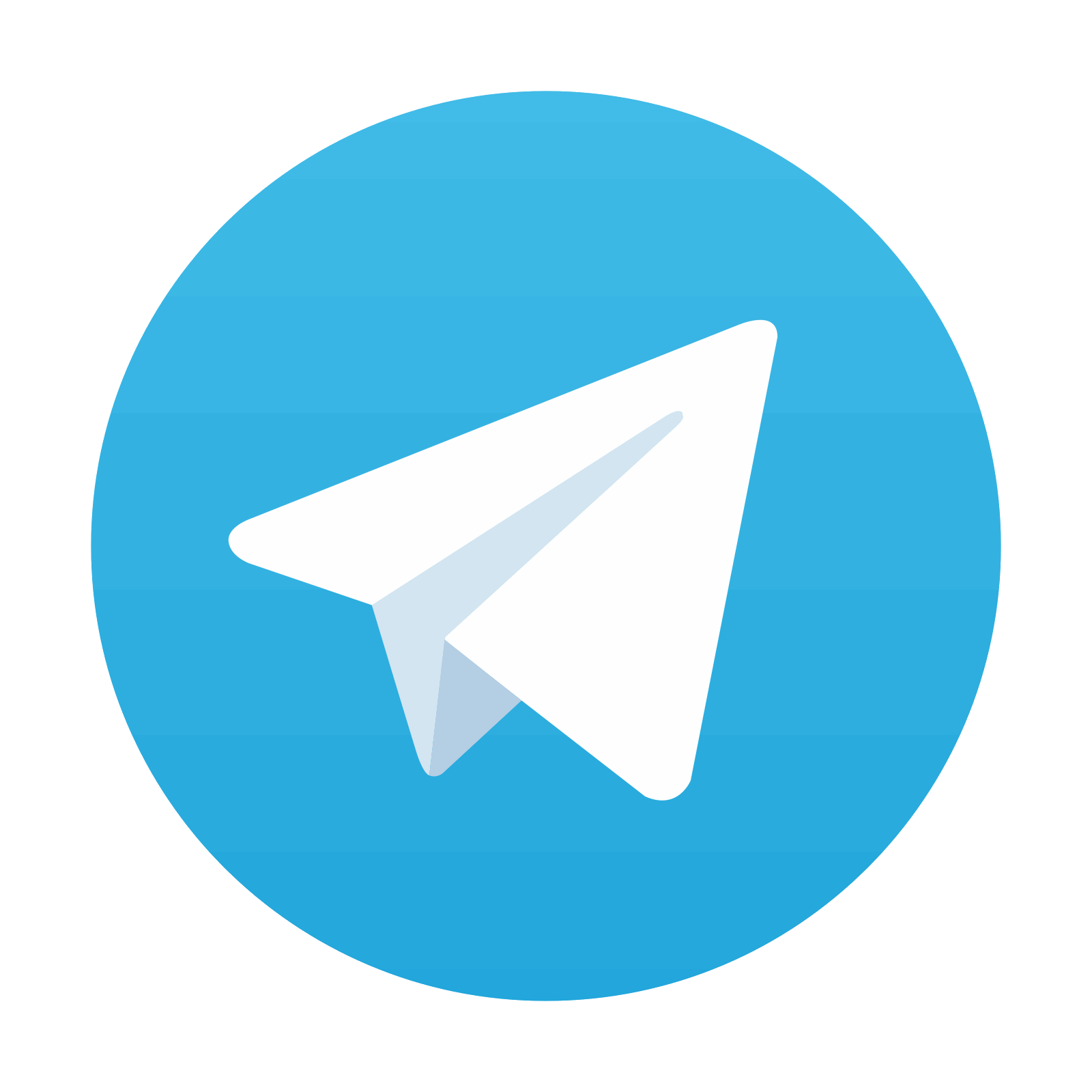
Stay updated, free articles. Join our Telegram channel

Full access? Get Clinical Tree
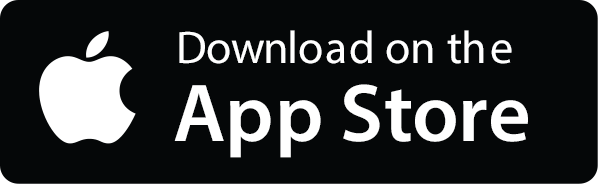
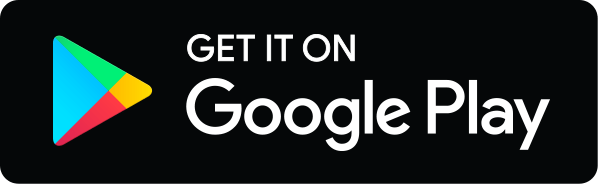
