The perfect racing car is the one which breaks down a moment after the finish line.
Introduction
While aging starts with being born and is part of life, the duration of life has progressively expanded over time from prehistory to present times. In the last century, lifespan has taken a huge jump and today humans are living longer than ever. Now the post-World War II generation is entering its senior years, and the geriatric population will increase tremendously in the next few decades.
Surgery dates from the Neolithic period, but major advancements have occurred in recent decades that have allowed for previously unimaginable procedures. Aging is associated with both a reduced capability to cope with external stressors – such as surgery – and an increased need for surgical care to treat different conditions. So, aging and the need for surgery have been proceeding in parallel and this has allowed octogenarians and over to become the fastest growing segment of the surgical population in most advanced countries.
This chapter will examine the definition of aging and aging processes and will provide some keys to understanding how age-related functional changes may interfere with perioperative phenomenological events.
Definition of Aging
An age of 65 years is widely accepted as the threshold for being considered “older,” but – as this definition is not universally applicable – there is no standard numerical criterion presently in use. Persons aged 65–74 years are generally designated as “young old”, those aged 75–84 years “middle old” and older than 85 years “oldest old.”
Individuals age at different rates, and defining aging could be difficult, mostly because it is not the sum of all the diseases that marks the limit, but principally diminished physiological reserves. Most evolutionary biologists define aging as “an age-dependent or age-progressive decline in intrinsic physiological functions, leading to an increase in age-specific mortality rate and a decrease in age-specific reproductive rate.” Another definition considers aging “a decline or loss of adaptation occurring with increasing age, caused by a time-progressive decline in forces that regulate natural selection.”
While aging appears to be an almost universal feature of life, aging rates and lifespan show great diversity, even within humans. At the same time, people are often interested in learning what they can do to live longer and to remain healthier during their later years, and views about aging are changing rapidly.
Chronological and Biological Age
Older people are as heterogeneous and varied a group as the rest of the population; this is essentially due to differences between chronological and biological age. Whereas chronological age coincides with the amount of time a person has lived, biological age results from the sum of aging processes, associated conditions, lifestyle and genetics.
Various descriptors of biological age, including “successful aging,” “frailty” or “telomere measurement,” have been proposed (Kim and Jazwinsky 2015). However, no quantitative evidence-based measure exists for clinical or research purposes. Comprehensive Geriatric Assessment (see Chapter 2) is a set of measures that explores health and functional status and offers a reliable appreciation of biological age.
From a physiological standpoint, aging is characterized by progressive, interindividual variation and not standardized constriction of each organ system’s capacity to maintain homeostasis in the face of challenge. Individuals become more dissimilar as they age, belying any stereotype of age. Thus, whereas biological age outlines a personalized, multifaceted profile that may help in predicting outcome after a stressful event, chronological age should be essentially regarded simply as a demographic variable.
Theories on Aging
Aging is a large and complex topic, and presently there are more questions than answers. Historically, from a biological point of view, evolution was not on our side regarding longevity, because it took place in an era of scarcity, whereas today many of us live in an era of abundance. However, our DNA really hasn’t changed very much in the past 100,000 years.
More than 300 theories have been postulated to explain aging (Figure 1.1). Most refer to the “programmed aging” and “wear and tear” paradigms. The first group includes the neuroendocrine theory of aging (biological clocks to regulate the aging process), the immune theory (the immune system as a pacemaker of the aging process) and the finite cell division theory (human fibroblasts in culture can only divide a limited number of times). In the second group, free radicals, metabolic rate, error catastrophe, DNA damage, glycosylation and decreased protein function are postulated to play a role in the aging process itself. Cellular waste accumulation-based theories have also been postulated.
However, the biological process differs from diseases of aging, and most of the theories suffer from the fact that they take one well-documented aspect of aging and try to explain from it the whole phenomenology of aging (Armbrecht and Coe 2000).
The term “senemorphism” has been recently proposed (Siqueira Trinidada et al. 2012) in regard to a plastic aging phenotype that can be generated through genetic pathways in response to environmental conditions. The authors stressed the importance of caste, reproduction and diet, taking into account effects of caloric restriction on oxidative damage, expression of several genes, stem cell loss, hormone homeostasis, fitness and cause of death. The conclusion was that aging cannot be regarded as a single process, rather the aging phenotype depends on how environmental conditions influence the expression of genes.
A new view on some old evolutionary theories is currently under discussion (Globerson and Reznick 2011). The theory of mutation accumulation proposes that genes beneficial in early life are favored by natural selection over genes beneficial late in life and young organisms are favored over old by their contribution to creating a new generation. This means that the power of natural selection fades with age, making it possible for hazardous late-acting genes to accumulate with old age. Another theory (antagonistic pleiotrophy) claims that the same genes that are beneficial at younger ages become harmful at older ages, and can have an effect on several traits of the organism.
But, in spite of many theories explaining negative effects of aging processes, having a biological age that is younger than the chronological one today seems a feasible perspective, with the maintenance of physical and cognitive independence until old age. Contrary to the stereotype of later life as a time of loneliness, depression and decline, a growing body of research shows that, in many ways, life gets better as we get older. Evidence indicates that our moods and overall sense of wellbeing improve with age.
Effects of Aging on Organs and Apparatuses
The Concept of Homeostenosis
Homeostasis is the process through which the body maintains internal equilibrium. Age inevitably induces a progressive reduction in the global functional reserve and the physiologic reserves of every organ and system, which become less resilient. So, homeostatic mechanisms deteriorate with aging, although there is wide variability in this dysfunction. In addition, with aging, more physiologic reserves are required to maintain homeostasis when the body is not at rest, and organisms become less resistant.
Homeostenosis is the characteristic, progressive constriction of homeostatic reserve that occurs with aging in every organ and system (Khan 2017). Stenosis refers to the narrowing of a structure. In the aging process, it means an encroachment on function, that in Figure 1.2 corresponds to the progressively smaller area laying under the physiologic limit curve. With aging, homeostenosis increases the vulnerability of organs to disease, although a homeostenotic system is not necessarily diseased. When the body undergoes stress states such as exercise, trauma, infection or surgical aggression, physiologic reserves are used to maintain homeostasis. The greater the stress, the more physiologic reserves are needed.
As a result, a stress easily overcome by the young organ (represented in the left side of the figure) may put the older organ’s ability to maintain homeostasis beyond the physiologic limit, leading to an acute injury or disease state (see the right side of the figure, where a sharp decline in physiologic reserve is represented). This trend illustrates the reduction in reserves that occurs at the most advanced ages and explains why the oldest individuals frequently become frail, susceptible to more disease and injury, and less able to recover from stressful situations. Homeostenosis highlights the reduced ability in older subjects to recover after an aggressive or stressful state (Troncale 1966).
Functional Decline
All organs and apparatuses lose their functional reserve with lifespan. The rate of decline varies depending on the organ and system, and on individual characteristics mainly associated with healthy habits, quality of life and probably genetics. It is noteworthy that in the global drop-off of functional reserve, 80 years old marks a sharp limit for all organs and systems. Functional changes occur in all organs and apparatuses; in the following sections, changes in organs that are most relevant to the perioperative course are described.
Respiratory System
Aging is marked by significant changes in the respiratory system. Lung function and capacity reaches its maximum around the age of 20 years and is maintained for about 10–20 more years (Smith 1986). Deterioration then sets in, as some 1% of capacity is lost with each year of life (Wahba 1983).
The most important changes consist of reduced elastic recoil of lungs, decreased compliance of the chest wall and diminished respiratory muscle force. Elastic recoil begins to decrease at the rate of 0.2 cmH2O each year after the age of 20, leading to a loss of 50% at around the age of 50 years (Janssens et al. 1999). Senile emphysema, increased residual volume and functional residual capacity are the main consequences. Changes in chest structure (kyphosis, vertebral collapse, calcification) reduce rib mobility and chest compliance, leading to increased breathing work and reduced functional reserve in acute conditions. Decreased respiratory muscle strength can decrease maximal inspiratory and expiratory force by as much as 50%.
All these changes lead to a 20% reduction in the surface area available for gas exchange and modify the ventilation–perfusion ratio. Gas exchange surface reduces from approximately 75 m2 at 30 years to 60 m2 at 75 years, and – by the age of 90 years – 30% of the surface available at 30 years may have been lost.
Declines in reflexes governing coughing and swallowing, and diminished sensitivity to hypoxia and – to a lesser degree – to hypercapnia, also occur.
The changes described above are important causal and concausal factors for postoperative atelectasis, respiratory failure, aspiration and infection.
Cardiovascular System
The main cardiovascular changes induced by aging are related to replacement of flexible, functional cardiac and vascular tissue by stiff material. As a result, compliance of cardiovascular structures progressively reduces. The large arteries of the body lose their elasticity, with a stiffer aorta resulting in increased peripheral resistances, that are further increased by the increased sympathetic tone that establishes with aging.
The aortic valve is the cardiac valve most frequently affected by age-related pathological changes. Blood pressure normally increases and may remain slightly high, even if treated. A variable degree of left ventricular hypertrophy develops as an adaptive mechanism to the increased peripheral resistance. Hypertrophy is accompanied by inadequate tissue perfusion, leading to myocardium–coronary-artery disproportion and risk of ischemic disorders.
Clinically important diastolic dysfunction likely involves poor ventricular relaxation in early diastole, as well as the natural ventricular tissue stiffening from aging and hypertrophy. This predisposes to heart failure, pulmonary congestion and cardiac decompensation.
Stiffening in veins reduces the ability to buffer changes in volemia and the capability to compensate vasodilation. At the autonomic nervous system level, both reduced β-adrenergic and baroreflex responsiveness further reduce the compensatory tachycardia in response to hypovolemia.
To eject blood into a more rigid aorta, the left ventricle has to put in an increased amount of work. In the older heart, the reduction in functional reserves limits the extent to which cardiac output and peripheral oxygen delivery can be augmented to compensate increased metabolic demands induced by exertion, sepsis or shivering. In the presence of atherosclerotic lesions, this reduced capability to defend general and regional flow against the usual perioperative challenges can cause even moderate hypotension to turn in intolerable reduction in cerebral, cardiac or renal flow, with consequent increased risk of ischemic complications (see chapter 36).
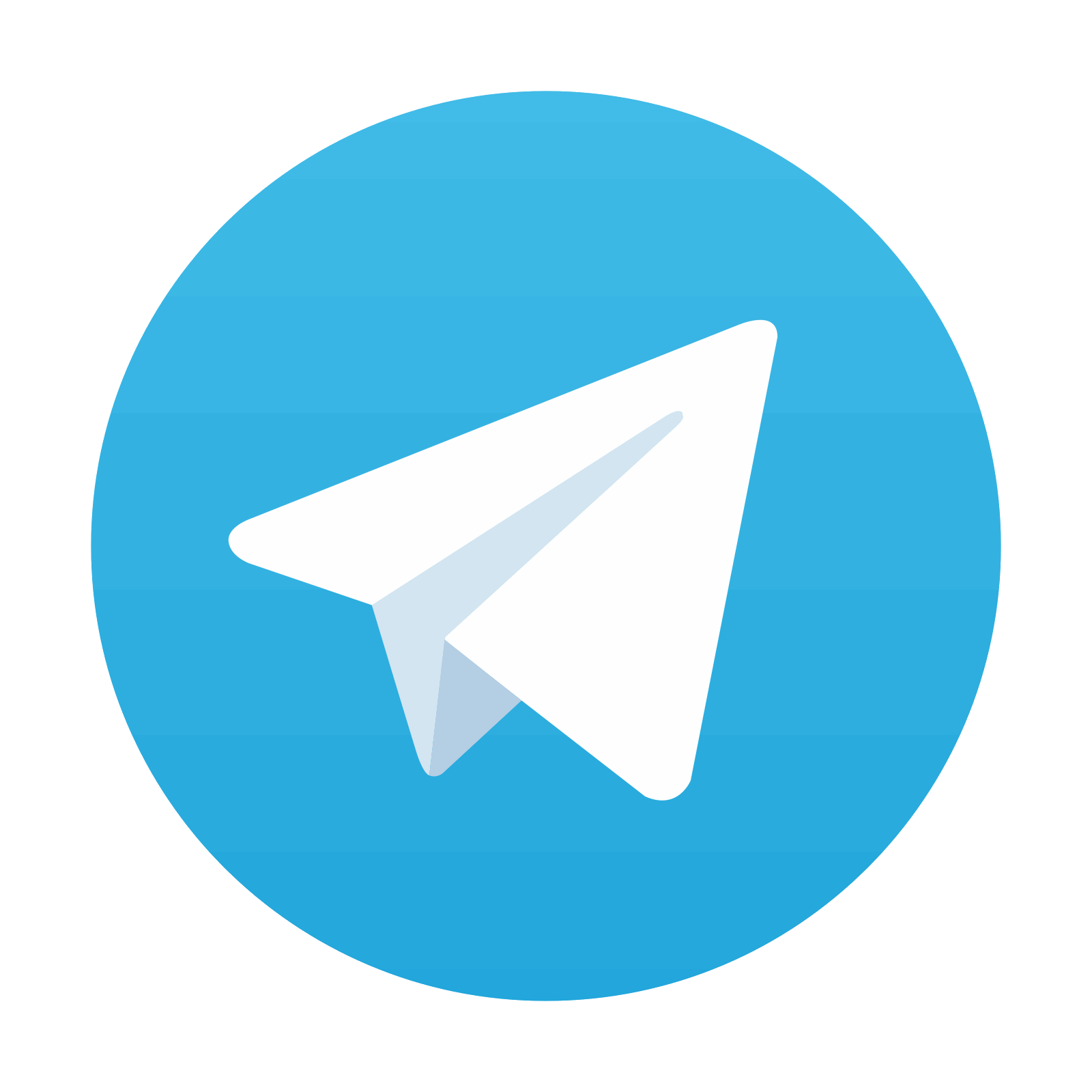
Stay updated, free articles. Join our Telegram channel

Full access? Get Clinical Tree
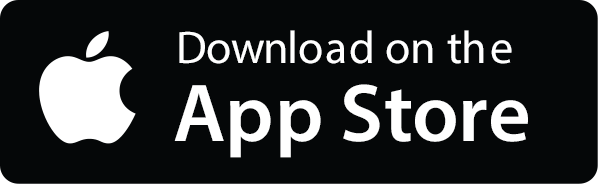
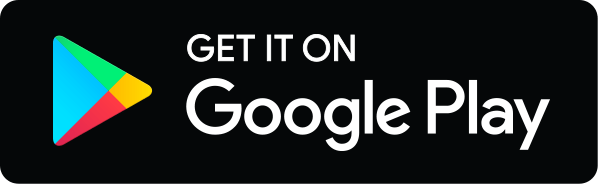
