In the general adult population, the causes of disruptions of arterial flow can be separated into three major categories based on cause: large vessel atherothrombotic disease (small vessel (lacunar) infarction, and cardiogenic embolism. This categorization will focus the diagnostic evaluation and therapy and has prognostic implications. Determining the cause will also guide the clinician in administering the appropriate level of care based on the possibility of progression and anticipated complications. For example, subtle speech changes and right-hand weakness could be the presenting symptoms of any of the three stroke subtypes outlined above. If a lacunar infarction is the cause, symptoms will most likely be maximal at onset and rarely will the patient experience complications compromising respiration or circulation. The volume of brain tissue involved is by definition small, and the risk for progression or recurrence in the acute period is quite small. A cardiogenic embolus would also be expected to cause symptoms maximal at onset, but the infarct volume could be large enough to cause mass effect and, depending on the underlying cardiac pathology, the risk for complications could be substantial.
Large Vessel Atherothrombosis
Large vessel atherothrombosis encompasses approximately 15% of all strokes; of these, two-thirds are of extracranial internal carotid artery (ICA) origin, and one-third are due to intracranial atheromatous disease (2). Atheromatous disease of large vessels is a slowly degenerative process, but as the disease progresses, the chance for lesion instability increases. Vascular plaques may fragment, exposing an ulcerated surface that is highly thrombogenic and leading to local occlusion or creation of emboli material. Thus, in large vessel disease, thrombosis or artery-to-artery embolism are often parts of the same underlying pathologic process. Atherosclerotic lesions tend to occur at bifurcations or sharp turns in the vessel, both of which are associated with increased blood flow turbulence. The prototypic example of this is carotid stenosis at the origin of the ICA. Other common extracranial sites include the origin of the vertebral artery and the other great vessels. Intracranially, common sites include the distal vertebral artery, the midbasilar artery, the siphon of the ICA, and the proximal middle cerebral artery (MCA).
TABLE 124.2 Conditions Most Frequently Mistaken for Stroke |
![]() |
Lacunar Strokes
Lacunar strokes represent approximately one-quarter of all such events. They are caused by occlusion of a single perforating arteriole, such as those that supply deep brain structures like the thalamus, pons, or basal ganglia (Fig. 124.1). The result is a small infarction—by most definitions, less than 1 cm3—that undergoes liquefaction necrosis with time, leaving a tiny fluid-filled space for which they are named. The primary risk factor for lacunar infarction is hypertension, which results in lipohyalinosis of the vessel wall with progressive concentric stenosis and eventual thrombosis (3).
Cardiogenic Embolism
Approximately 60% of all ischemic strokes are caused by cerebral embolism, of which only one-third have a definitively known clinical source (4). Cerebral emboli may be composed of atherosclerotic plaque material, clotted blood, or, in rare cases, air or fat. Once free in the arterial circulation, the emboli will tend to follow the straightest path formed by the most blood. Therefore, most emboli will affect distal branches of the MCA, although other locations are possible; the larger the embolus, the more proximal it will lodge.

FIGURE 124.1 Intracerebral hemorrhage of the left basal ganglia. Hyperdense appearance of blood on the CT scan easily defines the extent of hemorrhage.
Cerebral emboli may originate from atheromatous disease of more proximal large vessels, such as the aortic arch, as outlined above, or the heart (5). Atrial fibrillation is the most common cardiac cause, but others include valvular heart disease, intracardiac thrombus, atrial myxoma, dilated cardiomyopathy, patent foramen ovale (PFO), especially when accompanied by an atrial septal defect, and endocarditis. Air emboli are usually iatrogenic and result when a large amount of air enters the venous circulation (e.g., through a central venous catheter) and bypasses the lungs through a PFO, thereby entering the arterial circulation. Fat emboli are generally the result of long-bone fractures in severe trauma. It is important to seek out the definitive source whenever possible, as it may have a profound impact on the management of secondary stroke prevention. For example, although most sources of cardiogenic emboli are treated with oral anticoagulation, several conditions may contraindicate it, such as bacterial endocarditis (6) or atrial myxoma (7).
Arterial Dissections
Although arterial dissections may occur at any age, they are probably the most common cause of stroke in young patients (<50 years old) who are unlikely to have typical risk factors. Arterial dissections may arise spontaneously or following a traumatic head or neck injury (8). These lesions typically arise at the petrous portion of the ICA or at the C1–2 level in the vertebral artery. Thrombus may form at the site of intimal tear, extending into the media, with subsequent artery-to-artery embolism. If a large intimal flap or intramural hematoma forms, occlusion of the affected vessel may occur. Dissection may also lead to subarachnoid hemorrhage (SAH) when a pseudoaneurysm forms after the artery passes intradurally (9).
Clinical Evaluation
History
The history, when available, is the key instrument in diagnosing neurologic disease. If the patient is unable to provide a reliable history, which is often the case in acute brain dysfunction, then historic details should be sought from witnesses, family, EMS records, or whatever sources are available; no other diagnostic tool will so quickly narrow the differential diagnosis.
The key historic element in ischemic cerebrovascular disease is sudden onset of symptoms, which are typically maximal at onset. Under certain circumstances, the symptoms may follow a stuttering or stepwise progression, but in each instance there is a sudden change. Contrast this with the waxing and waning character of delirium or the symptoms that may develop over days to weeks from a brain tumor. There is an unfortunate tendency to dismiss symptoms of short duration, but this is a serious mistake, as the duration of the symptoms speaks little to the underlying pathogenesis. For example, a patient with occult atrial fibrillation may suffer transient neurologic deficits from an embolus that happens to spontaneously lyse before infarction has completed. Without addressing the underlying cause, the patient is unlikely to continue to be so fortunate, and an opportunity to prevent a devastating neurologic injury will have been lost.
If the ictal event is consistent with a stroke, the remainder of the initial history should be focused on determining if the patient is a candidate for thrombolytic therapy and identifying possible risk factors. Whereas other details not elucidated in the initial history can be revisited at a later time, it is of the utmost importance to obtain the exact time of symptom onset, as current acute stroke treatment protocols depend on this for inclusion. If the onset was not witnessed, then the time when last known to be normal is used. For example, if the patient awakens with symptoms, then the time when the patient retired is used (assuming he or she was asymptomatic then). The reason time of onset is so important is that it is used as a surrogate marker for the likelihood that salvageable tissue remains. Additionally, the risk of intracerebral hemorrhage (ICH) associated with thrombolytics increases with time after onset of symptoms. Current research is focused on using multimodal imaging to generate physiologic data that may be used in lieu of time to identify patients with a salvageable penumbra, but these techniques have yet to mature.
TABLE 124.3 Inclusion Criteria for Tissue Plasminogen Activator Use within 3 Hours from Symptom Onset |
![]() |
Aside from time of onset, factors that may place the patient at increased risk for bleeding must be sought. Tables 124.3 to 124.6 list common inclusion and exclusion criteria for intravenous (IV) tissue plasminogen activator (tPA), which are based on those used in the pivotal trials. Most institutions have their own protocols, which may vary somewhat from those used in the trials.
TABLE 124.4 Absolute Contraindications to Tissue Plasminogen Activator Use |
![]() |
TABLE 124.5 Relative Contraindications to Tissue Plasminogen Activator Use |
![]() |
Once a decision regarding acute treatment has been made, attention can be directed to identifying conditions that may have caused the patient’s stroke. Cerebrovascular and cardiovascular diseases share many of the same risk factors, including hypertension, hyperlipidemia, diabetes mellitus, cigarette smoking, and obesity. Other risk factors that may be important include obstructive sleep apnea, migraine headaches with an aura, and drug abuse. Family history may provide insight into heritable causes of stroke such as cerebral autosomal dominant arteriopathy with subcortical infarcts and leukoencephalopathy (CADASIL) or Fabry disease.
Neurologic Examination
The neurologic examination will allow the clinician to quickly determine which brain areas are dysfunctional and further narrow the differential diagnosis. The neurologic deficits caused by ischemic cerebrovascular disease are expected to be lateralizing and confined to a vascular distribution. For example, the triad of language disturbance and right face and arm motor deficits is typical for occlusion of the left MCA. However, sudden-onset motor and sensory deficit of both arms is not lateralizing, nor readily explained by a cerebrovascular occlusion, and thus is more likely due to spinal cord pathology.
The first step in localization of vascular lesions is determining, based on the signs and symptoms, whether they arise from the anterior circulation (carotid artery and its main branches, the anterior and middle cerebral arteries) or the posterior circulation (vertebral, basilar, and posterior cerebral arteries). This finding will guide the remainder of the diagnostic evaluation, therapy, and prognosis. Ideally, these two separate circulations would be robustly connected such that a failure in one could be compensated by the other, but this is rarely the case.
TABLE 124.6 Additional Inclusion and Exclusion Criteria for the Use of IV tPA within 3 and 4.5 Hours from Symptom Onset |
![]() |
The two symptoms that most accurately reflect carotid circulation disease are aphasia and monocular blindness. Aphasia is a deficit in either the expression or comprehension of language and may involve both in the acute period. Aphasia must be distinguished from dysarthria, which is the inability to correctly produce words due to motor impairment of facial, lingual, or pharyngeal muscles; dysarthria may result from either anterior or posterior circulation infarcts. The areas responsible for language reside in the dominant (nearly always left) hemispheric cortex, within the territory of the MCA; a stroke causing aphasia must, therefore, involve this circulation. Similarly, the blood supply of the eye arises largely from the ophthalmic artery, a direct branch from the carotid artery, and monocular ischemia, therefore, implicates the carotid circulation. The prototypic example of this process is amaurosis fugax, or transient monocular blindness, in which vision is lost in one eye for minutes. This must be contrasted with a visual field deficit, which affects one field of both eyes, as this is more likely the result of posterior circulation ischemia. Pain is rarely a significant complaint, but when present, especially if following the course of a major blood vessel, arterial dissection should be considered. Involvement of the carotid artery may cause Horner syndrome.
Because of the density of discrete populations of neurons supplied by the posterior circulation, the clinical syndromes that result from strokes in this area are usually more complex than those in the cerebral hemispheres. The medulla, pons, midbrain, cerebellum, parts of the thalami, and the visual cortices are the major structures involved. Strokes involving the brainstem often manifest with cranial nerve dysfunction (dysarthria, dysphagia, diplopia). Crossed signs, with motor or sensory deficits affecting one side of the face and the opposite side of the body, may occur as major decussations in these pathways and occur in the pons and medulla. The unique vascular anatomy of the basilar artery, with a single midline vessel supplying both sides of the pons and the posterior cerebral arteries, may lead to bilateral neurologic deficits.
Lacunar infarctions also have a set of clinical features that may be used to differentiate them from other stroke subtypes. Classic lacunar syndromes include pure motor hemiparesis (caused by infarction in the internal capsule or basis pontis), pure hemisensory symptoms (caused by infarction in the ventral posterolateral [VPL] thalamic nucleus), dysarthria–clumsy hand syndrome (with pontine or internal capsule infarcts), and ataxia–hemiparesis (pontine infarct).
Vascular System Examination
Physical examination of the vascular system itself is usually surprisingly unrewarding. Atherosclerosis may present few outward signs. Although carotid bruits were classically emphasized, modern ultrasound techniques have proven them to be of low sensitivity and specificity for predicting vascular disease. No characteristic feature, including the volume, pitch, or duration of the bruit, reliably indicates the degree or the nature of constriction of the vascular lumen. Many bruits reflect benign conditions. The clinical significance of carotid bruits is minimized because they are audible in many asymptomatic persons without atherosclerosis who never suffer from cerebrovascular disease, but may be absent in severely diseased vessels. Therefore, even if a carotid bruit is detected, it may be difficult to decide whether it is relevant to the patient’s symptoms, and it should not be given undue emphasis in the overall evaluation. Clinical decisions should be based on definitive assessment of blood vessels using ultrasound or angiography.
Examination of the heart should focus on detecting thrombogenic diseases, including myocardial infarction (MI), congestive heart failure, arrhythmias, prosthetic valves, and bacterial endocarditis. Heart disease is a key risk factor for stroke and may complicate the acute period. Patients with stroke may also present concurrently with a MI and, without careful examination, the less dramatic of the two may go undetected. Elevation of serum troponin levels is a very sensitive and specific indicator of an MI. Isolated creatine kinase (CK)-MB elevations should be interpreted with caution, however, as the MB fraction is expressed in brain tissue, and small elevations are not uncommon in stroke (10).
Laboratory Studies
Laboratory studies will also help narrow the differential diagnosis and may reveal relevant comorbid conditions. Some studies need to be obtained immediately to determine a patient’s candidacy for thrombolytic therapy, including an electrolyte battery, glucose, platelet count, cardiac enzymes, beta-human chorionic gonadotropin (b-HCG), and coagulation parameters. Severe electrolyte (specifically hyponatremia or hypernatremia) or glucose disturbances can cause neurologic dysfunction that may mimic stroke. Cardiac enzymes will determine if cardiac ischemia is part of the current presentation, and a b-HCG will reveal occult pregnancy, both of which may be contraindications to systemic thrombolytics. Coagulation parameters and a platelet count will identify patients who may be at greater risk for bleeding.
Other laboratory studies will help determine the cause and identify risk factors but do not need to be obtained immediately. A fasting lipid profile should be obtained for potential vascular risk factor modification. In patients more than 50 years of age, an erythrocyte sedimentation rate and C-reactive protein are essential if giant cell arteritis is suspected. In patients for whom an unusual cause of stroke is suspected (young patients or minimal vascular risk factors), laboratory investigation of prothrombotic states could be considered. The interpretation of these tests is very complex and should be performed in consultation with a hematologist (11). Toxicology screening should be performed on hospital admission, with attention directed to amphetamines, phencyclidine, ephedrine, and cocaine.
Imaging Studies
CT Scan
Several imaging studies are used in the evaluation of acute stroke. A noncontrasted CT scan of the brain is the standard initial evaluation for stroke. CT is very sensitive to the presence of hemorrhage, which is the primary reason for its use in the acute setting, and is the only radiologic test necessary to determine eligibility for IV-tPA. CT is not sensitive for the detection of an acute cerebral infarction (Fig. 124.2), and the lack of abnormality within the first 24 hours should be expected. The view of the posterior fossa is also quite limited, and any changes (with the exception of hemorrhage) seen within the brainstem or cerebellum should be confirmed with MRI. When available, CT angiography (CTA) and perfusion (CTP) studies can be obtained with very little additional time and provide valuable additional information regarding the patency of blood vessels and blood flow to individual large vessel territories. Pathologic changes on these studies are visible immediately, but they require iodinated contrast, which may be a limiting factor for some patients. The angiographic results from CTA are closest to the traditional gold standard examination: digital subtraction catheter angiography (DSA). When examining the carotid arteries, the results from CTA are often sufficient to differentiate a high-grade stenosis (60% to 99%) that is treatable from complete occlusion that is not. Use of CTP in conjunction with noncontrasted CT brain and CTA can help determine tissue that is potentially salvageable with reperfusion strategies.
MRI
MRI is far more sensitive for acute infarction than CT. Diffusion MR sequences can detect ischemia within, perhaps, minutes of onset. MRI is of special value in brainstem and posterior circulation strokes, since the images it produces are not obscured by bony artifacts as with CT (Fig. 124.3). The combination of multiple MRI sequences allows for much more specific differentiation between ischemic brain and other structural abnormalities. In addition, MRI can display flow-related enhancement of the vasculature, resulting in a magnetic resonance angiogram (MRA). The resulting image can be manipulated in three dimensions, allowing for more accurate interpretation of small abnormalities. MRA uses gadolinium as a contrast agent instead of an iodinated material, making the study available to more patients. Perfusion studies, very similar to those performed with CT, may also be obtained if the right equipment is available. Disadvantages of MRI and MRA include reduced availability and substantially longer scanning times, which place some limitations on their use.

FIGURE 124.2 Evolving radiographic evidence of cerebral infarction. A: CT scan at 3 hours shows little evidence of acute ischemia. B: At 3 days, damaged brain is indicated by hypodensity in the left subcortical region. C: At 8 days, frank infarction is now clearly demonstrated by CT.

FIGURE 124.3 MRI demonstrates enhancing left lateral medullary infarction in a patient with Wallenberg syndrome.
DSA
DSA is an invasive imaging technique in which the artery of interest is selectively catheterized under fluoroscopy and dye injection enables a high-resolution view of the vessel that can be obtained in multiple planes. As the quality of noninvasive imaging techniques has improved and the associated morbidity is approximately 1% (12), DSA is no longer used as a routine screening test. Indications now include precisely delimiting critical vascular stenoses and examination of arterial dissections, arteriovenous malformations (AVMs), and aneurysms.
Carotid Ultrasound
Carotid ultrasound provides a rapid noninvasive assessment of carotid artery disease, based on abnormalities of either flow (Doppler) or morphology (B-mode). As with any ultrasound technique, sensitivity is to some degree operator-dependent, but with experienced technicians and interpreters, duplex scanning provides a reproducible, accurate screening examination for carotid disease. However, this technique suffers from the same limitation as MRA in differentiating high-grade stenosis and occlusion. Lesions of the more distal ICA may also be difficult to visualize in some patients.
Transcranial Doppler
Transcranial Doppler (TCD) allows rapid bedside assessment of abnormal flow within the distal ICA and major intracranial arteries. It is primarily a functional study that provides information about blood flow velocity and vascular resistance rather than structural features. The 2-MHz ultrasonic signal can penetrate various bony “windows” in most patients, and its gated character allows identification of arteries by “depth” of the reflected signal. TCD can examine proximal portions of all major branches of the circle of Willis, but is insensitive for pathology beyond the A1, M1, or P1 segments. Newer applications include detection of microemboli and online monitoring of arterial flow during invasive procedures, such as carotid endarterectomy (Fig. 124.4). TCD may also be useful as an adjunctive therapy to tPA, as continuous insonation may enhance thrombolysis (13). Disadvantages include major dependency on operator skill and the prevalence of acoustically inadequate bony windows.
Transthoracic Echocardiography
Transthoracic echocardiography (TTE) is essential to evaluate cardiac function. Physicians must attend not only to a visualized thrombus, but also to other pathologic states associated with systemic embolization, including left ventricular wall motion abnormalities, chamber dilatation, valvular disease, ejection fraction, and septal defects. TTE can be routinely performed and is a superior study for the detection of ventricular apex pathology, left ventricle thrombus, and views of prosthetic valves. Transesophageal echocardiography (TEE) provides much greater resolution and is more sensitive for pathology of the left atrial appendage, interatrial septum, atrial aspect of mitral–tricuspid valves, and the ascending aorta. TEE is an invasive procedure that requires sedation but can be performed safely on most patients (14).
MANAGEMENT
Acute Therapy
As with any acutely ill patient, attention should initially be focused on the evaluation of airway, breathing, and circulation. A secure airway should be established for patients with depressed levels of consciousness. Supplemental oxygen or mechanical ventilation should be used as needed to treat any degree of hypoxia. Circulation assessment includes evaluation of blood pressure and cardiac electrical activity with an ECG, as coexistent MI is not uncommon. Patients with acute stroke are often markedly hypertensive, and one should be cautious in aggressively treating elevated blood pressure before a more complete assessment of the patient has been completed. Blood pressure goals are determined by type of stroke, cause, and the presence of comorbid conditions, such as coronary artery disease; this will be discussed in the following sections.
The immediate goal will be to determine if the patient is a candidate for thrombolytic therapy; thus attention should be focused on obtaining the relevant history and performing a neurologic examination. The care of patients who will ultimately not receive thrombolytic therapy will be discussed below (see Supportive Care). Crucial for therapy is the proper determination of the exact time of onset of the stroke. The patient must be witnessed to have had an abrupt change in neurologic status by a reliable observer; otherwise the time of onset, by default, must be the last time the patient was seen at his or her baseline level of neurologic function. All patients should be evaluated with the National Institutes of Health Stroke Scale (NIHSS), which can help to exclude a patient from potentially harmful therapy on the basis of the stroke being too small or too severe.

FIGURE 124.4 Transcranial Doppler. A: The normal flow-velocity profile through the middle cerebral artery (velocity plotted over time during three cardiac cycles) is demonstrated. B: Elevated flow velocities as a result of the arterial spasm associated with subarachnoid hemorrhage is demonstrated. C: Two microemboli are detected through the middle cerebral artery as transient high-intensity signals.
Thrombolytic Therapy
Currently, thrombolytic treatment with IV-tPA in eligible stroke patients is the standard of care based on the results of several large randomized controlled trials (15–22). The collective results indicate that patients treated with tPA within 4.5 hours of onset had significantly higher odds of a good stroke outcome (23). The average disability across all groups was, additionally, decreased in the treatment group. The benefit seen by the tPA-treated group existed regardless of patient age or stroke subtype. It is important to acknowledge, however, that while the American Stroke Association recommends IV-tPA within 4.5 hours of onset in eligible patients, the Food and Drug Association has only approved the use of tPA within 3 hours of onset (24,25). Table 124.6 provides additional inclusion and relative exclusion criteria for use of tPA in the 3- to 4.5-hour window.
To maximize the possible benefit and minimize the risk for hemorrhage, the National Institute of Neurological Disorders and Stroke (NINDS) study helped to establish strict inclusion criteria for the administration of thrombolytic therapy in acute stroke patients, which are outlined in Table 124.3 (15). Absolute exclusion criteria have been established as well (see Table 124.4). In addition, there is a list of relative contraindications to thrombolytic therapy (see Table 124.5), which often vary slightly in institutional protocols. These can be summarized as risks for bleeding from a noncompressible site, the presence of potential stroke mimics, or uncontrollable hypertension. The rationale for excluding patients with improving symptoms is to avoid giving a potentially harmful treatment to patients with epileptic postictal presentations or spontaneous recovery.
When the protocol is followed, the administration of tPA is safe relative to other commonly accepted treatments. Several studies have attempted to examine the risk, but perhaps the most clinically relevant is the number needed to harm. For every 100 patients treated with tPA who match the NINDS trials populations across all levels of final global disability, approximately 32 will receive benefit and 3 will be harmed (26). The risk–benefit ratio, thus, strongly favors treatment. Many of the hemorrhages that occur are asymptomatic, and of those that are symptomatic, not all symptoms persist to discharge. Most patients who experience ICH after tPA therapy have severe baseline insults and were already destined for a poor outcome; thus the hemorrhage does little to alter the final outcome (27). One should keep in mind that, although excluding a patient from treatment will mitigate the risk for hemorrhage, it also denies the patient a significantly better chance of recovery.
The dose of tPA is 0.9 mg/kg, with a maximum dose of 90 mg; 10% is given as a bolus over 1 minute, and the remaining 90% is infused over 60 minutes. Following treatment, patients should be monitored in an intensive care unit (ICU) for at least 24 hours. While many post-tPA patients will not need ventilatory or vasopressor support, as do many other patients in the ICU, blood pressure monitoring and frequent neurologic examinations are critical for a favorable outcome, as hypertension dramatically increases the risk for hemorrhage. Blood pressure must initially be monitored noninvasively as arterial puncture is contraindicated for 24 hours after the administration of tPA. All other invasive procedures, such as placement of nasogastric tubes, urinary bladder catheters, central venous lines, intramuscular injections, and rectal temperature, should be avoided for 24 hours as well. Also, any drug that impairs hemostasis such as heparin, or antiplatelet or nonsteroidal anti-inflammatory agents is contraindicated during this 24-hour period.
TABLE 124.7 Management of Blood Pressure During and After Treatment with Tissue Plasminogen Activator or Other Acute Reperfusion Intervention |
![]() |
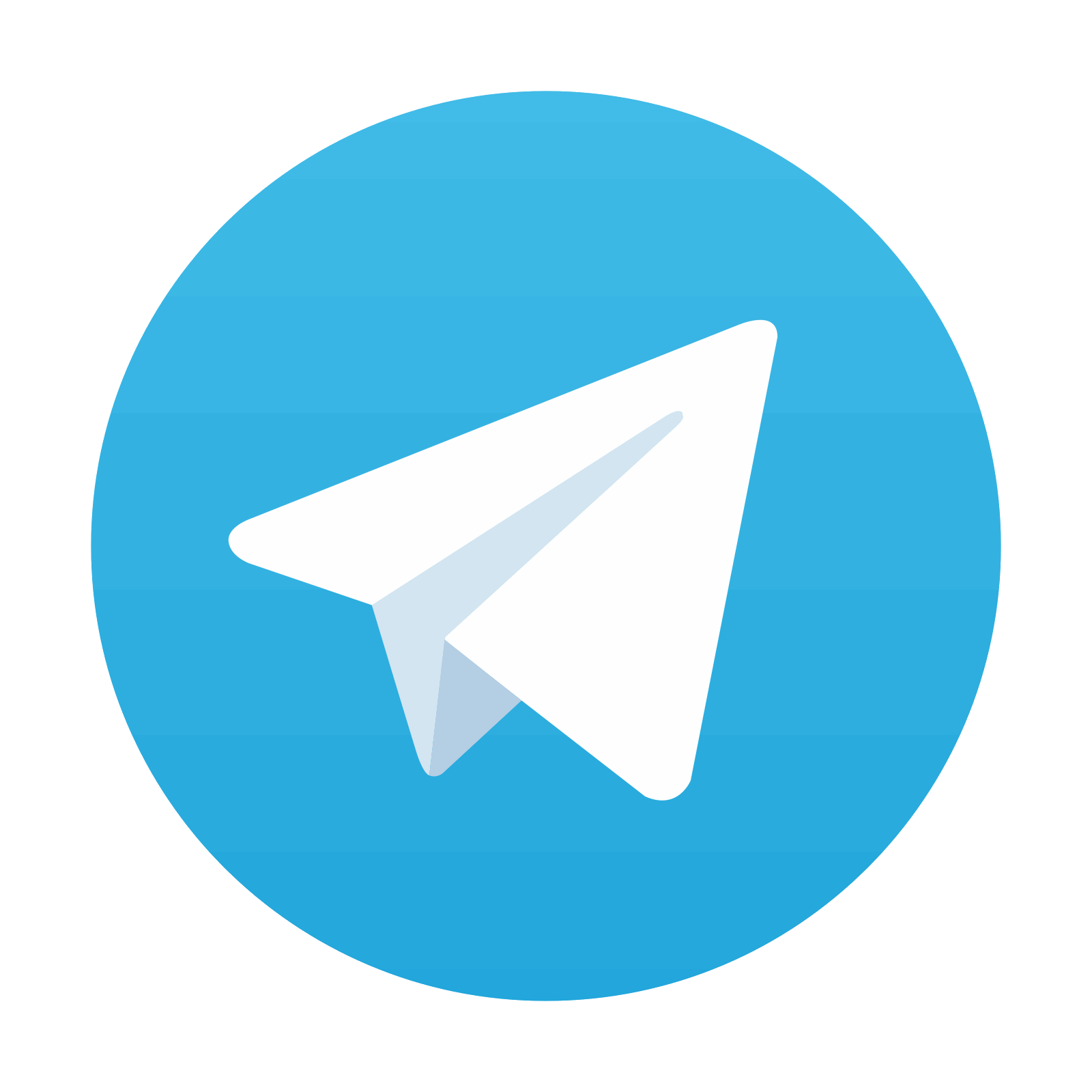
Stay updated, free articles. Join our Telegram channel

Full access? Get Clinical Tree
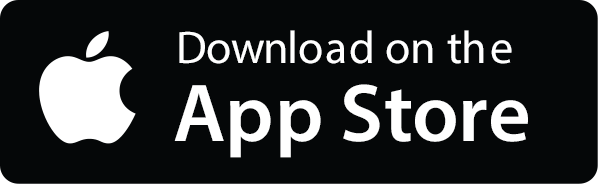
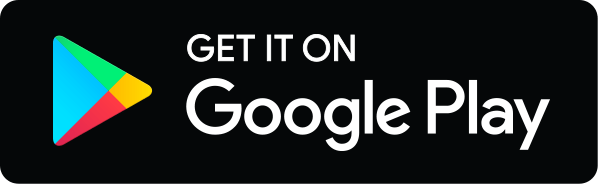