Central Nervous System
Josh Finkle
Jeffrey Kirsch
▪ INTRODUCTION
The majority of anesthetic procedures involve the central nervous system (CNS), from inducing unconsciousness to performing regional anesthesia. In order to understand anesthesia, the anesthesia technician will need a basic understanding of the anatomy and physiology of the CNS. This chapter introduces the anatomy of the brain and spinal cord, which make up the CNS. The chapter also covers the physiology of the CNS beginning with the main cells of the CNS, neurons.
▪ ANATOMY OF THE BRAIN
The central and peripheral nervous systems together make up a complex network of cells and fibers that extends throughout the body. This network uses electrical and chemical signals to gather, interpret, and react to information from the external environment and from within the body. The CNS processes and integrates signals received by the peripheral nervous system and sends instructions to the rest of the body. Signals going into and out of the CNS are carried by peripheral nerves, which interact directly with the environment.
The CNS can be divided into two distinct anatomic structures: the brain and the spinal cord. The brain is a complex organ with many functions, including the processing of sensory input, the generation of movement, and higher functions such as cognition, language, and emotions. The brain is functionally divided, with specialized regions that are primarily responsible for unique sets of functions. The structures that collectively make up the brain are the cerebral hemispheres, the central cerebral structures, the brainstem, and the cerebellum (Fig. 13.1).
The cerebral hemispheres are the most prominent of the brain’s structures and consist primarily of the cerebral cortex, and some underlying white matter, which are axons that serve to connect cells between brain regions. The cortical tissue of the cerebral hemispheres contains many folds, called gyri, and grooves, called sulci. The cerebral hemispheres can be divided into four major areas, or lobes: frontal, temporal, parietal, and occipital lobes (Fig. 13.2). Each lobe of the cortex contains specialized areas that are responsible for unique functions and responses, leading to a predictable distribution of the functional areas of the cortex. The occipital lobe is primarily associated with the processing of visual information and the formation of a coherent interpretation of the visual world. The temporal lobe is the primary site of auditory perception and contains a structure called the hippocampus, which is responsible for storage and retrieval of memories. The parietal lobe is a locus for integration of multiple senses and is responsible for the understanding of symbolic language and spatial relationships. The frontal lobe is responsible for executive functions, such as attention, conscious motor movement, and behavioral control.
Knowledge of the link between structure and function allows the clinician to predict the location of pathology based on clinical presentation. It also allows the surgeon to warn patients preoperatively regarding expected postoperative neurologic deficits following brain tissue resection during tumor and seizure surgery. During any brain surgery, surgeons and patients are challenged with balancing the opportunity for cure (e.g., complete resection of a tumor with surrounding tissue) with the devastation that resection may cause to an individual’s postoperative cognitive and functional outcomes.
Internal to the cerebral cortex, there are a number of structures that serve to connect and modulate the signals of the rest of the CNS. The basal ganglia, a set of nuclei below the cortex, are responsible for modulation of complex
motor signals sent from the frontal cortex. The thalamus is a large central structure within the cerebral hemispheres, and acts as a sensory integration point, with regions corresponding to inputs from various sensory modalities (vision, audition, touch, pain) on their way to the cortex. The hypothalamus is a region just below the thalamus with many important functions, the most notable of which is to connect the CNS to the endocrine system. The hypothalamus has direct input to the principal endocrine organ, called the pituitary gland, which sits just below the hypothalamus and controls chemically mediated signaling functions such as growth, metabolism, blood pressure, and sexual development. The cerebellum is in the posterior aspect of the skull, below the occipital lobe of the cortex. The cerebellum has connections to other CNS structures, including the cerebral cortex, the basal ganglia, and the spinal cord. The cerebellum’s major function is to integrate tactile and proprioceptive sensory inputs with motor signals for the
production of smooth motion and the maintenance of balance and posture.
motor signals sent from the frontal cortex. The thalamus is a large central structure within the cerebral hemispheres, and acts as a sensory integration point, with regions corresponding to inputs from various sensory modalities (vision, audition, touch, pain) on their way to the cortex. The hypothalamus is a region just below the thalamus with many important functions, the most notable of which is to connect the CNS to the endocrine system. The hypothalamus has direct input to the principal endocrine organ, called the pituitary gland, which sits just below the hypothalamus and controls chemically mediated signaling functions such as growth, metabolism, blood pressure, and sexual development. The cerebellum is in the posterior aspect of the skull, below the occipital lobe of the cortex. The cerebellum has connections to other CNS structures, including the cerebral cortex, the basal ganglia, and the spinal cord. The cerebellum’s major function is to integrate tactile and proprioceptive sensory inputs with motor signals for the
production of smooth motion and the maintenance of balance and posture.
The brainstem is the most caudal structure in the brain, and it connects the higher areas of the brain to the spinal cord. The sensory and motor pathways from the body (carried by the spinal cord) and the sensory pathways from the head (the trigeminal system) pass through the brainstem and make important functional connections. The brainstem is divided both structurally and functionally into three areas—the midbrain, pons, and medulla (from rostral to caudal). The medulla controls vital and unconscious functions, including breathing, heart rate, blood-vessel tone, and vomiting. Throughout the brainstem are nuclei (collections of cell bodies) for the cranial nerves, a set of nerves that control various functions within the head and neck, such as taste, audition, and sensation, as well as eye movements, vocalization, and facial expression.
Within the cranial cavity there are structures that offer support to the tissues of the brain, namely, the meninges and the vasculature. The meninges are a set of three layers of protective tissue that surround the structures of the CNS (Fig. 13.3). The outermost of the meninges, the dura mater, is a thick protective layer that directly contacts the skull. Just below the dura mater is the arachnoid mater, which surrounds a fluid-filled cavity called the subarachnoid space. The subarachnoid space contains cerebrospinal fluid (CSF) that acts as a cushion for the brain against traumatic insults. The pia mater is a thin layer that runs along the surface of the brain, following the sulci and gyri of the cerebral cortex. The blood supply to the brain comes from the vertebral arteries and the internal carotid arteries, which meet on the ventral surface of the brain to form an arterial network, referred to as the circle of Willis. Branching off the circle of Willis are the arteries that supply the regions of the brain,
including the cerebral arteries, cerebellar arteries, and their branches. Blood returning from the brain enters a network of cavities within the dura mater, called venous sinuses, which collectively drain into the internal jugular vein.
including the cerebral arteries, cerebellar arteries, and their branches. Blood returning from the brain enters a network of cavities within the dura mater, called venous sinuses, which collectively drain into the internal jugular vein.
▪ CLINICAL IMPLICATIONS OF ANATOMY
The rigid structure of the skull and meninges in combination with an increase in the intracranial volume of any component within the intracranial vault (e.g., brain, CSF, blood, or foreign bodies) will result in an increase in intracranial pressure and secondary brain injury. The brain component can increase because of abnormal growth (e.g., tumor) or swelling (e.g., from trauma or surgery). The blood component can increase because of ruptured blood vessels (i.e., ruptured aneurysm, hemorrhagic stroke, or trauma). Unfortunately, all of the inhaled (gas) anesthetics cause dilation of the cerebral blood vessels and an increase in intracranial pressure. Therefore, a typical neuroanesthetic will include an intravenous anesthetic (e.g., propofol, opiates) at concentrations that minimize the doses of inhaled anesthetic required to maintain a reasonable plane of anesthesia. The CSF component can increase from overproduction or poor reabsorption. Although each of these pathologies results in increased intracranial pressure, the definitive treatment is usually left to the discretion of the surgeon.
Pressure within the brain can be measured via a catheter placed in the central CSF-containing spaces (e.g., lateral ventricles), or a “bolt” can be placed through the skull and a fluid connection with the CSF space is established through the dura and arachnoid tissues. In both cases, the device is attached to a transducer (as one would use for measurement of invasive blood pressure or central venous pressure) that is zeroed and balanced at the level of the external auditory canal. Alternatively, a fiber-optic Camino device can be placed by the surgeons into the brain parenchyma. Although all techniques provide an accurate assessment of intracranial pressure, only the catheter in the lateral ventricle can be used therapeutically to lower intracranial pressure by withdrawing CSF.
Other means of decreasing intracranial pressure that are under the control of the anesthesiologist include hyperventilation (low pCO2 causes cerebral vasoconstriction and decreases blood volume in the brain) and intravenous administration of osmotic diuretics (e.g., mannitol and hypertonic saline), which reduce the amount of edema fluid in the brain. Because of the use of hyperventilation by anesthesiologists to lower intracranial pressure in patients having intracranial surgery, the anesthesia technician is likely to observe low arterial pCO2 values and high pH values on blood gases taken during the procedure. In addition, the administration of osmotic diuretics to neurosurgery patients will often result in an increase in the osmolarity measurement by the anesthesia technician.
▪ ANATOMY OF THE SPINAL CORD
The spinal cord begins just below the base of the brainstem, and continues down, through the vertebral column. The vertebral column is divided into four segments based on anatomic location (Fig 13.4). The cervical region of the vertebral column spans the length of the neck and has seven segments. The thoracic region spans the upper part of the back and is divided into 12 segments. The lumbar region spans the lower back and is divided into five segments. The sacral region of the vertebral column extends into the pelvis and is composed of five bones fused to form a single structure. A single coccygeal bone (referred to as the tailbone) is found at the end of the spinal column. Found within the vertebral column is the spinal cord itself. Although in the adult the spinal cord ends in the thoracic region of the vertebral column, the spinal cord levels are divided into segments based on the spinal column level for exit of their paired sensory and motor spinal nerves. Each of the four regions of the spinal cord controls motor and sensory functions for a specific part of the body. For example, the cervical spinal cord controls motion and sensation from the neck and upper extremities. The thoracic spinal cord controls motion and sensation from the trunk. The lumbar and sacral regions of the spinal cord control motion and sensation in the lower extremity and the pelvic/genital region.
Within the spinal cord, there is gray matter and white matter. The gray matter is composed primarily of neuronal cell bodies, and the white matter is composed of long bundles of nerve cell tissue for signal conduction. The meningeal layers continue inferiorly and surround the spinal cord throughout its length. At the level of the spinal cord, the three layers of the meninges lie
within the vertebral column and external to the spinal cord itself. As with the intracranial CNS, the meninges and CSF serve an important role to protect the spinal cord from trauma.
within the vertebral column and external to the spinal cord itself. As with the intracranial CNS, the meninges and CSF serve an important role to protect the spinal cord from trauma.
![]() ▪ FIGURE 13.4 Segmental organization of the spinal cord. The spinal cord is divided into cervical, thoracic, lumbar, and sacral divisions (left). The right side shows the spinal cord within the vertebral column. Spinal nerves are named for the level of the spinal cord from which they exit and are numbered in order from rostral to caudal. (From Bear MF, Connors BW, Parasido MA. Neuroscience—Exploring the Brain. 2nd ed. Philadelphia, PA: Lippincott Williams & Wilkins; 2001, with permission.) |
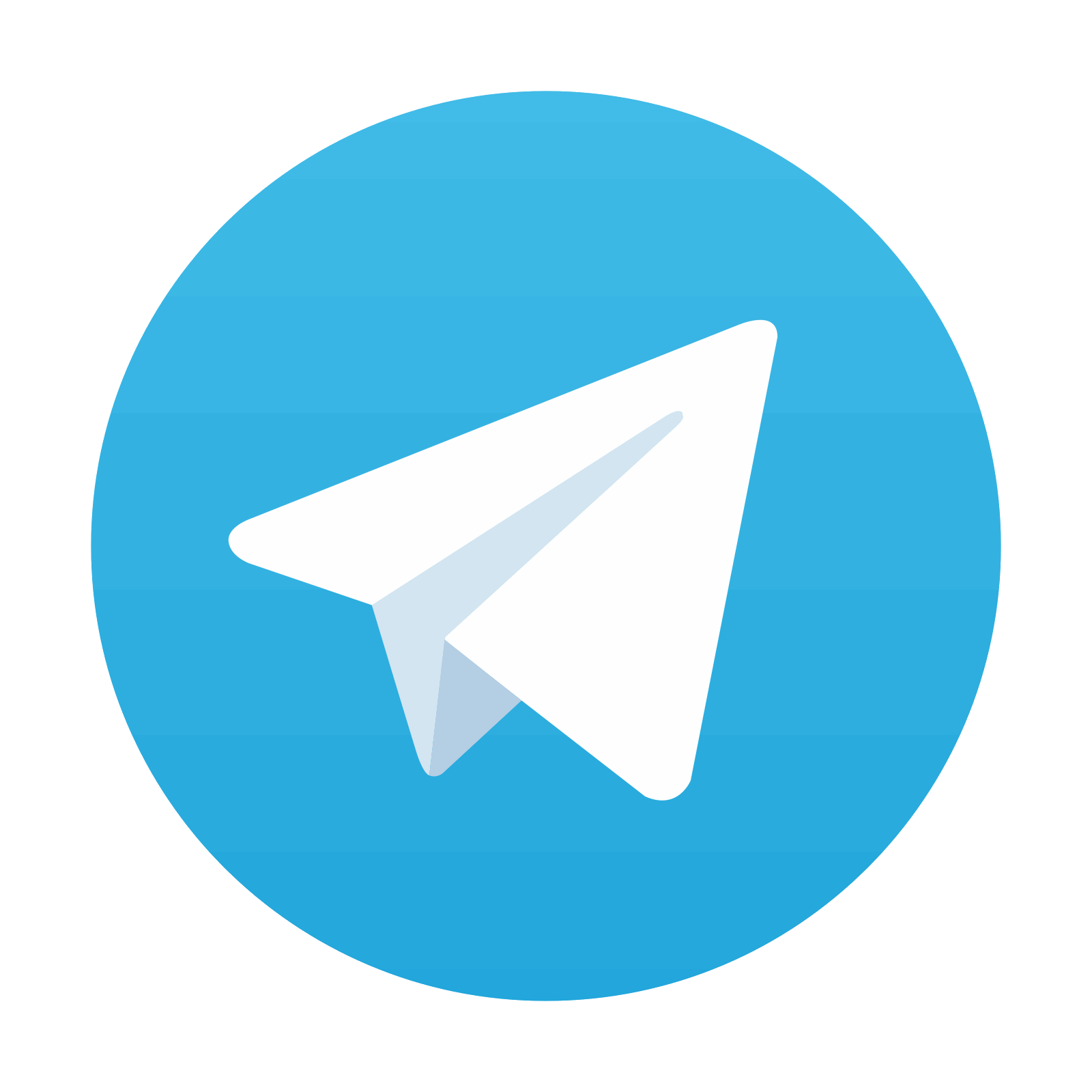
Stay updated, free articles. Join our Telegram channel

Full access? Get Clinical Tree
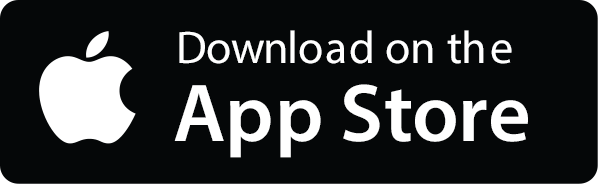
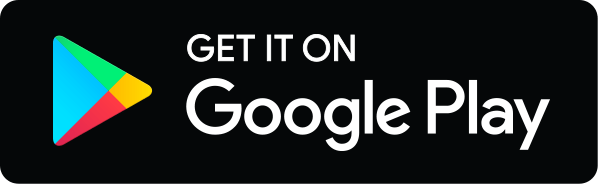