↑ glycogen synthesis
↑ protein synthesis
↑ triacylglycerol synthesis
↑ fatty acid synthesis
↓ glycogenolysis
↓ ketone formation
↓ breakdown of triglycerides
↑ ketone formation
↑ gluconeogenesis
↑ gluconeogenesis
↑ lipolysis
↑ lipolysis
↑ protein catabolism
↓ DNA synthesis
↑ lipolysis
↑ RNA synthesis
↑ protein synthesis
↑ basal metabolic rate
↓ protein synthesis
uncouples oxidative phosphorylation
Organ systems and homeostatic control
Organ systems and their control mechanisms provide the macroscopic means of controlling homeostasis and interfacing the body with its external environment. The traditionally described systems, such as the cardiovascular, respiratory and neurological systems, all exert their control over the body’s physiology by well-recognised mechanisms.
Intracellular control mechanisms
Activation or inhibition of enzymes
Regulation of gene expression
Changes in membrane permeability
Regulation of membrane receptor activity
Changes in membrane potential
Negative feedback systems
The most common homeostatic control mechanism is the negative feedback system. A negative feedback system operates to maintain a constant output parameter or steady state, even if the output parameter is disturbed by an applied stimulus. When the steady state is disturbed the system first detects the change in the output parameter. It then produces an opposite polarity signal (negative feedback signal) proportional to the output deviation, and feeds this signal back to the input of the system. This feedback signal changes the input, and thus acts to correct the output deviation (Figure 10.2).
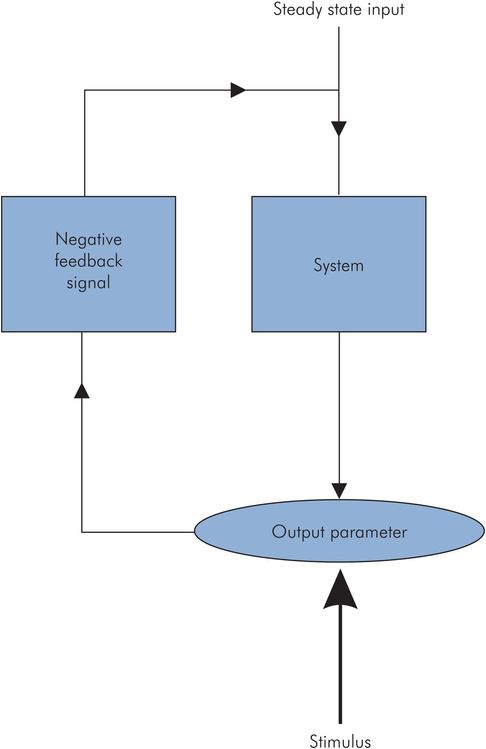
Negative feedback system
The physiological parameter may be a variable such as mean arterial blood pressure, which rests normally at its operating point. When a change occurs in the blood pressure, the change is detected by a baroreceptor that relays a signal to an integrating centre (vasomotor centre of the medulla). The integrating centre then transmits a signal to an effector (vascular smooth muscle), which exerts a response to oppose the original change.
Positive feedback systems
Positive feedback systems are also used for physiological control, although in these cases the system is not used to control a specific physiological parameter, but rather to produce a systemic response directed towards maintaining homeostasis. In such a system there is no polarity change in the feedback signal, and thus, instead of opposing the detected physiological change, the feedback signal acts to increase the deviation. This produces a cascade effect that can be identified in certain physiological responses, such as the coagulation cascade during blood clot formation.
Cell structure
Cellular function is reflected by cell structure. In broad terms, a cell consists of the cell plasma membrane, the cytosol, intracellular organelles and the nucleus.
Basic cell morphology
A basic cell is illustrated in Figure 10.3. The outer cell membrane surrounds various functional structures or organelles, the largest of which is the nucleus. The medium surrounding the nucleus is the cytoplasm.
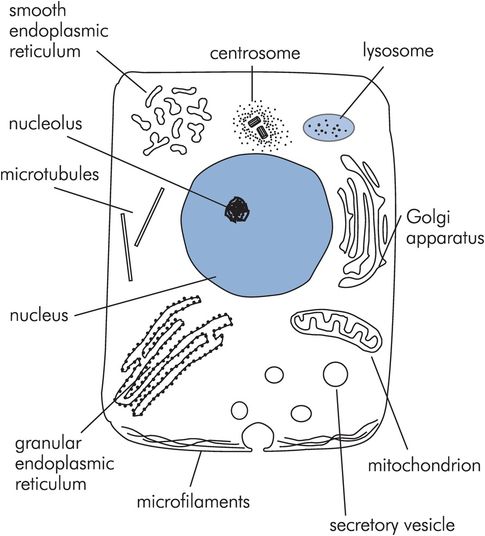
A basic cell
Cytoplasm
‘Cytoplasm’ is used to describe all intracellular contents outside the nucleus. It consists of the cytosol and the organelles.
Cytosol
Cytosol refers to the intracelluar fluid containing proteins and electrolytes. Intracellular fluid forms 40% of body weight and possesses an electrolyte composition, which is discussed in Chapter 11.
Organelles
A summary of the organelles and their functions is shown in Figure 10.4.
Cytoskeleton | Maintains the structure of the cell |
Membrane | Container for cell, nucleus and other organelles Structural support and control of environment on either side |
Mitochondria | Energy source for the cell, generating ATP via oxidative phosphorylation |
Nucleus | Contains the genetic material in the form of chromosomes The central site of cell division or mitosis Nucleolus synthesises ribosomes |
Centrosome | Formation of mitotic spindle in cell division |
Endoplasmic reticulum (ER) | Rough (granular) ER has ribosomes attached and is responsible for protein synthesis Smooth (agranular) ER is the site of steroid synthesis and detoxification |
Ribosome | The actual site of protein synthesis in the ER, or may occur free in cytoplasm |
Golgi apparatus | Processes proteins for secretion from cell |
Lysosomes | Breakdown and elimination of intracellular debris or exogenous substances |
Peroxisomes | Catalyse various anabolic and catabolic reactions, e.g. breakdown of long chain fatty acids |
Cilia | Used by the cell to propel mucus or other substances over exposed mucosal surfaces |
Cytoskeleton
This system of microscopic fibres maintains the cell structure and enables cell movement to occur. Its main components are:
Microtubules – 25 nm diameter structures with 5 nm thick walls. Tubule length is a dynamic balance between assembly (at the positive end) and disassembly (at the negative end) of protein subunits
Muscle thick filaments – composed of myosin 15 nm in diameter
Intermediate filaments – solid fibres about 10 nm in diameter
Microfilaments – solid fibres about 5 nm in diameter made of polymerised actin
Cellular motion, shape changes and ciliary or flagellar movement all involve molecular motor mechanisms based on the action of ATPases. These form moving flexible cross-bridges between cytoskeletal components and membranes or organelles. The actin–myosin mechanism responsible for muscle contraction is a molecular motor mechanism that occurs universally in other cells. Other examples of molecular motors include dynamin and kinesin, which act on microtubules.
Mitochondria
These are sausage-shaped structures with outer and inner membranes. Their main function is to produce chemical energy in the form of ATP by oxidative phosphorylation. The inner membrane is folded to form cristae, which are studded with units containing the oxidative phosphorylating and ATP-synthesising enzymes (Figure 10.5). The matrix contains enzymes required to drive the citric acid cycle, which in turn provides the substrate for oxidative phosphorylation. Mitochondria also contain a small amount of DNA, which is solely of maternal origin.
Endoplasmic reticulum (ER)
This membranous structure is composed of complex folds and tubules. In its granular form, ribosomes are attached to the cytoplasmic surfaces and are the primary site for protein synthesis in the cell. Agranular ER is free from ribosomes and is the site of steroid synthesis and detoxification.
Ribosomes
Ribosomes are about 32 nm in diameter, with large and small subunits. They are composed of 65% RNA and 35% protein, and are the sites of protein synthesis. Free ribosomes exist in the cytoplasm and synthesise haemoglobin, peroxisomal and mitochondrial proteins.
Centrosome
This is composed of two centrioles at right angles to each other. The centrioles are cylindrical structures in which nine triplets of microtubules form the walls. A cylinder of pericentriolar material surrounds these structures. The whole structure is situated near the nucleus and is activated at the start of mitosis. Initially the centrioles replicate, and then the centriole pairs separate to form the mitotic spindle.
Golgi apparatus
This consists of flattened membranous sacs or cisterns that are stacked together to form a polarised structure with cis and trans ends, separated by a middle region. The Golgi apparatus prepares proteins for secretion (via exocytosis) by receiving the proteins from the ER at the cis side, coding them for destination and finally producing secretory granules or vesicles at the trans side (Figure 10.6).
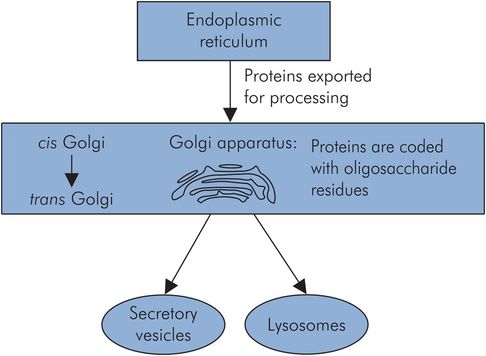
Golgi apparatus and protein processing
Intercellular junctions
The organisation of cells into tissues involves the formation of specialised junctions between the cells and the formation of an extracellular matrix surrounding the cells. Connective tissues such as bone and tendon are predominantly extracellular matrix with the cells anchored to the matrix rather than forming intercellular junctions. Epithelial tissues consist of layers of cells anchored to a basement membrane with little extracellular matrix, but dense specialised intercellular junctions determining the functions of the epithelium.
Some basic types of junction (Figure 10.7) are:
Anchoring junctions – forming cell–cell adhesions and cell matrix adhesions, and providing anchorage for the cytoskeleton. These give the tissue mechanical stability.
Occluding junctions – circumferential junctions that seal the extracellular space between epithelial cells, preventing the passage of molecules between cells.
Channel-forming junctions – small channels (diameter 1.5 nm) that allow transfer of small ions and molecules between cells.
Signal relaying junctions – allowing communication between cells.
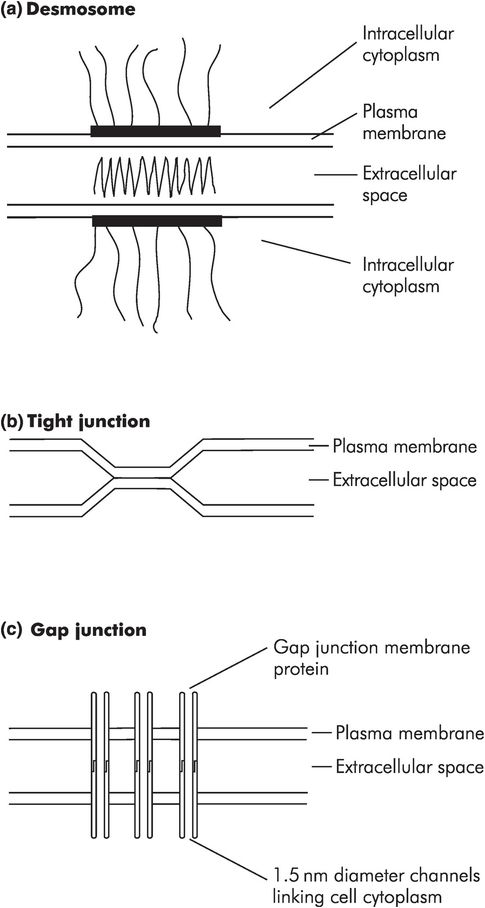
Intercellular junctions
Cilia
These projections on the luminal surface of epithelial cells are motile processes that move secretions and other substances across the surface of the cell. They are composed of nine pairs of microtubules arranged circumferentially around a central pair of microtubules. Each cilium is attached to a basal granule that has a structure similar to that of a centriole. Ciliary movement is produced by molecular motor mechanisms that cause the microtubules to slide relative to each other.
The cell membrane
Membranes surround all cells and the majority of intracellular organelles. The cell membrane has a primary function of controlling the passage of substances across it to maintain the intracellular environment, which is an essential requirement for cellular metabolism. Controlling the movement of ions across the membrane also establishes ion concentration gradients and electrical potential differences (membrane potential) across the membrane, which enable cells to generate action potentials in excitable tissues.
Membrane functions
A summary of cell membrane functions is as follows:
Regulation of the passage of substances across it for intracellular homeostasis
Establishment of ion concentration gradients (K+ and Na+) and membrane potential
Generation of action potentials
Container for cell contents
Anchorage for cytoskeleton
Structural function for tissues acting as a site for intercellular connections
Communication via chemical messengers
Communication via action potentials
Membrane structure
Cell membranes are based on a double phospholipid layer structure. The phospholipid molecules are amphipathic, with one end charged and the other non-polar. The membrane is formed by a double layer of these amphipathic molecules with the polar ends orientated outwards. The double layer is interrupted by integral membrane protein molecules, which often span the membrane completely and are referred to as transmembrane proteins. Membrane proteins and lipids may possess polysaccharide chains attached to their extracellular surface, which appears as a fuzzy coat visible on electron microscopy, known as the glycocalyx (Figure 10.8).
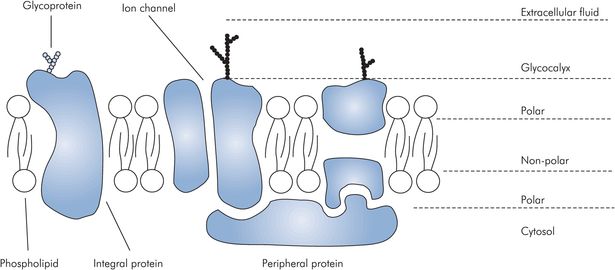
Cell plasma membrane
Integral membrane proteins
Integral membrane proteins have various functions. Some form controllable channels for the passage of ions or water. Another group transmits chemical signals across the cell membrane by acting as active carriers. Cell adhesion molecules determine the cell’s ability to attach to the extracellular matrix, basal membranes and other cells. Types of integral membrane proteins include:
Ion channels
Transport carriers
Cell adhesion molecules
G proteins
Second messenger enzymes
Peripheral membrane proteins are located on the cytoplasmic membrane surface, where they are attached to polar regions of the integral membrane proteins. These peripheral proteins are associated with cell motility and shape.
Cell adhesion molecules (CAMs)
Cell adhesion molecules form a large group of membrane proteins with several subdivisions and a wide range of functions. Some of the processes cell adhesion molecules are involved in include:
Transduction of signals controlling differentiation, gene expression and motility
Programmed assembly of cells to form the complex architecture of individual tissues
Morphogenesis of embryological tissues and organs
Formation of intercellular connections in epithelial tissues
Adhesion of leukocytes to vascular endothelium
Enablement of leukocyte motility
Directing of leukocyte migration in inflammation
Platelet adhesion in blood coagulation
Pathogenesis of airway inflammation in asthma
Epithelial cell adhesion to basement membranes and the pathogenesis of bullous diseases
An outline of the different CAMs is summarised in Figure 10.9.
Integrins | Platelet adhesion Expressed on leukocytes and bind to IgSF (endothelium) Leukocyte motility Cell-matrix adhesion |
Selectins | Expressed on circulating leukocytes Stored in endothelial cells and allow rolling of leukocytes Stored in platelets Leukocyte–endothelial adhesion |
Cadherins | Morphogenesis of tissues Metastasis of tumours Embryological development |
Immunoglobulin superfamily (IgSF) | Expressed on endothelium and bind to integrins (leukocytes) Expressed on gut mucosa and bind integrins and selectins (lymphocytes) |
Cell adhesion molecules (CAMs) form the basis for cells to adhere either to other cells (cadherins) or to the extracellular matrix (integrins). CAM properties also include signal transduction and transmission. The scope of CAM function thus extends well beyond a simple structural role, and involves both normal and pathological processes.
G proteins
This group of membrane proteins has a high affinity for guanine nucleotides. Over 16 G proteins have been identified, composed of α, β and γ subunits, suggesting the existence of many more. Activation of the G protein enables it to bind guanosine triphosphate (GTP) and interact with an effector protein. The activated G protein then deactivates itself by intrinsic GTPase activity (Figure 10.10). This reduces the GTP to GDP, thus deactivating the G protein.
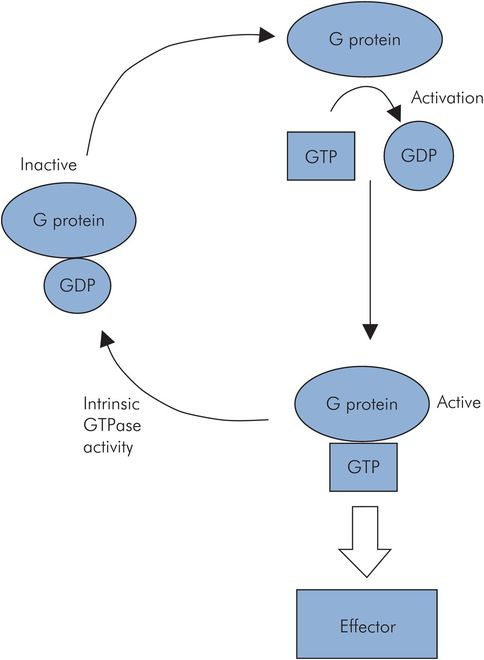
G protein activation and deactivation
Activation of the G protein system can result in various effects. It can control the release of second messengers via Gs- and Gi-type proteins that have stimulatory or inhibitory effects on enzymes such as adenylyl cyclase. Some G proteins are directly coupled to ion channels, and thus control membrane permeability to ions. Others can increase intracellular calcium concentrations and activate intracellular kinases.
The heterogeneous nature of G proteins means that a first messenger common to several tissues can produce a spectrum of different cellular responses according to the tissue targeted. This variability is further increased by the fact that more than one G protein may be activated by a single receptor, and several effector proteins can be coupled to a single G protein.
Second messenger enzymes
The production of second messengers – cyclic adenosine monophosphate (cAMP), cyclic guanosine monophosphate (cGMP), inositol triphosphate and diacylglycerol – takes place at the cell membrane. The enzymes responsible for their production are adenylyl cyclase, guanylyl cyclase and tyrosine kinase. The activities of these enzymes are controlled by various pathways, which involve both activation and inhibition. Second messenger effects are multiple and widespread intracellularly.
Membrane transport of substances
Cell membranes control the movement of a wide range of particles and substances between the intra- and extracellular spaces. These include gases, ions, water, proteins and intracellular granules or debris. Different components of the membrane are associated with different mechanisms of transport. The phospholipid bilayer areas of the membrane allow diffusion of water, small molecules and lipid-soluble substances. Transmembrane proteins provide active mechanisms for transport and allow ion diffusion via channels. Examples of substances transported across membranes are shown in Figure 10.11.
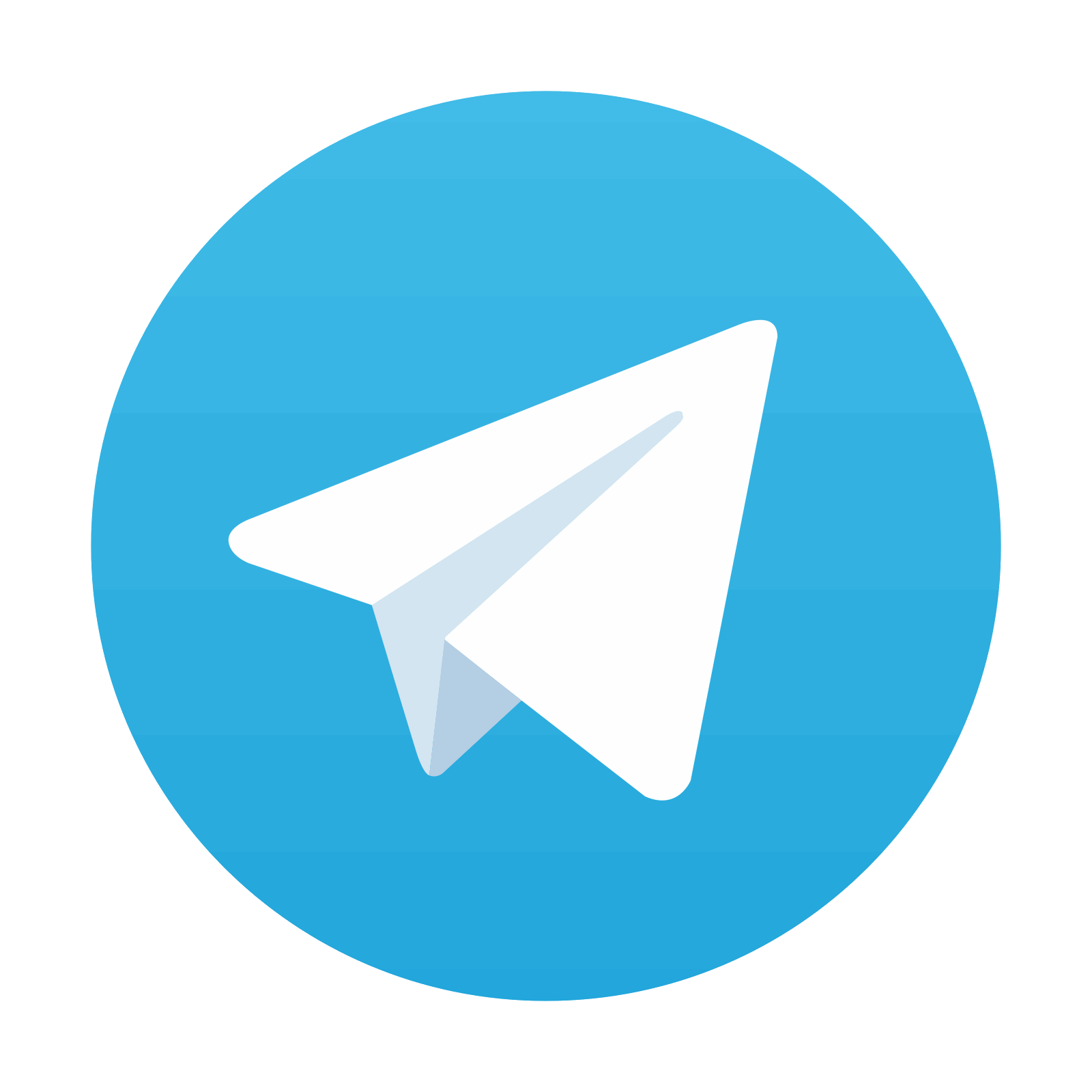
Stay updated, free articles. Join our Telegram channel

Full access? Get Clinical Tree
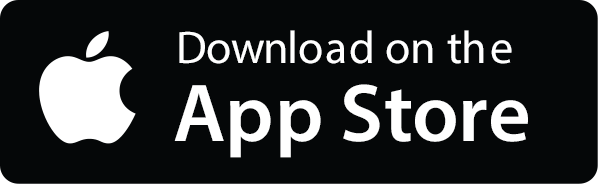
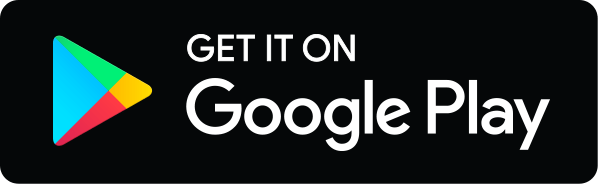