FIG. 38.1 Cerebral angiography allows x-ray visualization of the brain’s vascular system when a contrast dye is injected arterially. A, Insertion of dye through a catheter in the common carotid artery, subsequently outlining vessels of the brain. B, An angiogram using the subtraction technique. 1. Internal carotid artery. 2. Middle cerebral artery. 3. Anterior cerebral artery A1 segment. (From Black JM, Hokanson Hawks JH: Medical-surgical nursing: clinical management for positive outcomes, ed 7, St. Louis, MO, 2005, Elsevier.)
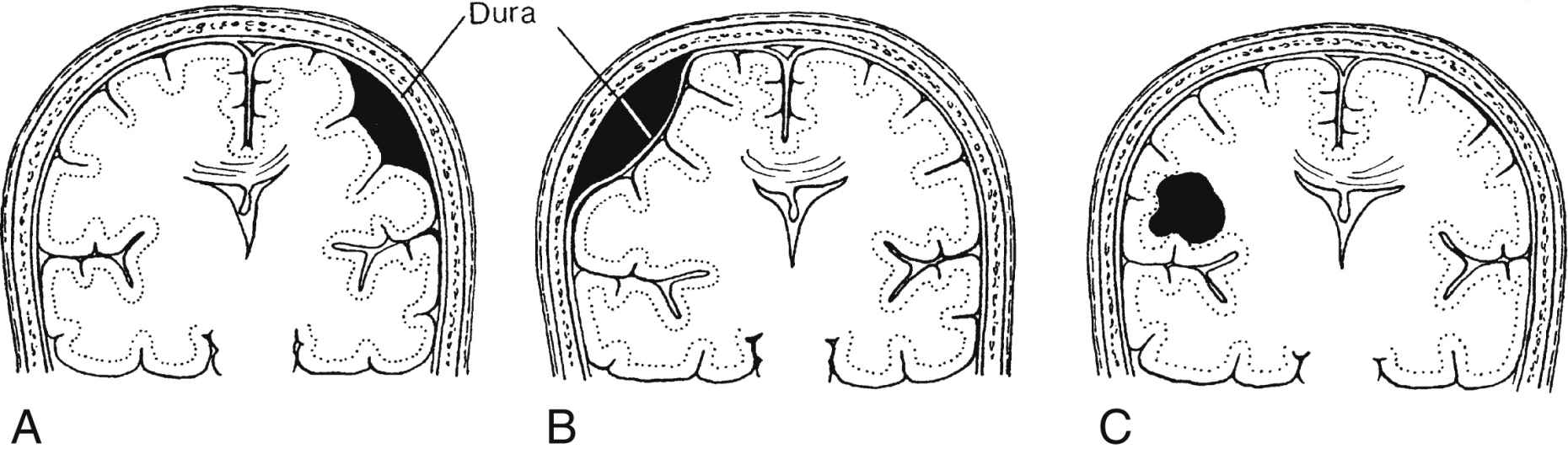
FIG. 38.2 Types of hematomas. A, Subdural hematoma. B, Epidural hematoma. C, Intracerebral hematoma. (From Black JM, Hokanson Hawks JH: Medical-surgical nursing: clinical management for positive outcomes, ed 8, St. Louis, MO, 2009, Elsevier.)
Consequences of Injury
Traumatic head injury can cause hemorrhage beneath a skull fracture or from a shearing of the veins or cortical arteries and results in epidural, subdural, subarachnoid, or intraventricular hemorrhage (Fig. 38.2). The signs and symptoms of brain ischemia and increased intracranial pressure (ICP) vary with the speed at which the functions of vital centers are altered. A small clot that accumulates rapidly may be fatal; however, the patient may survive a slowly developing, much larger hematoma through effective compensatory mechanisms.
An epidural hematoma, or extradural hematoma, accumulates in the epidural space, which is between the skull and the dura mater. The hematoma is most often arterial and caused from a rupture or laceration of the middle meningeal artery, which runs between the dura and the skull in the temporal region. Epidural hematomas may also be seen in the frontal, occipital, and posterior fossa regions. The patient usually loses consciousness and then has a lucid period after which a rapid deterioration occurs. The hemorrhage may be massive, and treatment consists of evacuation of the clot through burr holes made in the skull.
Subdural hematoma may result from trauma and the shearing of the bridging veins. Venous blood usually accumulates beneath the dura and spreads over the surface of the brain. A subdural hematoma may be acute, subacute, or chronic, depending on the size of the vessel involved and the amount of blood present. Patients with acute subdural hematomas have a rapid deterioration in condition and are critically ill.
Subacute subdural hematomas fail to show acute signs and symptoms at onset. Brain swelling is not great, but the hematoma may become large enough to produce symptoms. Progressive hemiparesis, obtundation, and aphasia often appear 2 to 14 days after injury. The degree of ultimate recovery depends on the extent of damage produced at the time of injury.
Chronic subdural hematomas are seen most often in older adults. A history of head injury may be lacking because the causative injury is often minimal and long forgotten or deemed insignificant by the patient. The history is usually one of progressive mental or personality changes with or without focal symptoms as blood slowly accumulates and compresses the brain. The blood itself becomes thicker and darker within 2 to 4 days and within a few weeks resembles motor oil in character and color. Papilledema may be present. Chronic subdural hematomas can mimic any disease that affects the brain or its coverings. Treatment consists of evacuation of the defibrinated blood through multiple burr holes or a craniotomy incision.
Intracerebral hematomas are more commonly found in the elderly, often after a fall, but are also seen as a result of spontaneous rupture of a weakened blood vessel or aneurysm. Hemorrhage may be scattered or isolated and occurs in the brain parenchyma. Surgical evacuation of an isolated or well-defined clot may be attempted, but the mortality rate remains high.
Subarachnoid hemorrhage may occur as the result of traumatic brain injury. Bleeding into the subarachnoid space may result in a vasospasm. A vasospasm is the narrowing of the blood vessel lumen and places the patient at risk for a delayed ischemic event. The risk of development of vasospasm is greatest 3 to 7 days after the bleed. Intraventricular hematoma, which is usually caused by a subarachnoid or intracerebral hemorrhage, is bleeding into the ventricles.6 This can be caused by brain trauma such as penetrating wounds or from an anterior communicating and basilar tip aneurysm.5 An intraventricular hematoma is associated with high mortality, and treatment includes a ventriculostomy with CSF drainage and ICP management.6
Herniation of the brain occurs from untreated increased ICP. Supratentorial herniation is regarded as an emergency more severe than an epidural hematoma. The tentorium is an extension of the dura mater, which forms a transverse partition or shelf that divides the cerebral hemispheres from the cerebellum and brainstem. The superior portion of the brainstem passes upward through an aperture in the tentorium known as the tentorial hiatus. No space-occupying mass or lesion that expands within the cerebral hemispheres can escape upward or outward because of the confinement of the skull. Consequently, expansion within and compression of the hemispheres cause herniation of its contents (usually a portion of the temporal lobe known as the uncus) through the tentorial hiatus.
Uncal herniation is accompanied by compression of the lateral brainstem on the same side, which thus shuts off its blood supply and suppresses certain basic functions. The third cranial nerve (oculomotor) is in close proximity to the herniated uncus, and the pupil on the injured side becomes fixed and dilated. The reticular activating system located in the brainstem responsible for waking and alertness becomes affected, and the patient rapidly becomes less and less responsive. Displacement of the midbrain causes compression of the pyramidal tract and results in contralateral hemiparesis or hemiplegia and plantar extensor responses (Babinski reflex). The respiratory center in the medulla may be affected, which results in changes in the respiratory pattern or cessation of respiration altogether.
In addition to these changes, the cerebellum itself may be so compressed that the cerebellar tonsil herniates inferiorly through the foramen magnum. This condition usually results in immediate death because the centers vital to life are compressed or sheared. The best treatment for supratentorial herniation is prevention through early detection and treatment of increased ICP and its causes. If efforts to minimize edema and increased ICP fail, surgical intervention, if possible, is necessary as a life-saving measure.
Types of Pathologic Conditions
Cerebral aneurysms are round dilations of the arterial wall that develop as a result of weakness of the wall from defects in the media layer of the artery. Most cerebral aneurysms occur at bifurcations close to the circle of Willis and usually involve the anterior portion. Common bifurcations include those with the internal carotid, the middle cerebral, and the basilar arteries and in relation to the anterior and posterior communicating arteries. The exact cause or precipitating factor is not well defined but may be related to congenital abnormality, arteriosclerosis, embolus, or trauma.
An intracranial AVM is a vascular network that appears as a tangled mass of dilated vessels that create an abnormal communication between the arterial and venous systems. The communication may be singular or multiple and resembles an arteriovenous fistula in that no connecting capillary system between the arteries and the veins exists. AVMs most commonly occur in the supratentorial structures and usually involve the vessels of the middle cerebral arteries. AVMs are usually present at birth as the result of congenital abnormalities but may have a delayed age of onset.
Intracranial tumors are space-occupying lesions that can be primary or metastatic. Primary tumors are classified as primary intracerebral (intraaxial) tumors, which originate from glia cells, or primary extracerebral (extraaxial) tumors, which originate from supporting structures of the nervous system. Metastatic tumors most commonly arise from breast malignant disease in women and lung malignant disease in men and women.7
Cerebral ischemia can occur in any area of the brain as a result of arterial occlusions or embolizations.8 The cerebrovascular accident (CVA) or stroke can be ischemic or hemorrhagic in nature and may produce various neurologic deficits, leading to death if not treated appropriately and efficiently. Patients may experience a CVA as a result of an operative procedure. Perianesthesia nurses may also see these patients as they are recovering from a neurologic procedure performed in the operating room. Special precautions should be taken as directed, especially if the patient has received thrombolytics. Neuroassessment should be completed frequently, blood pressure and cerebral perfusion should be monitored and controlled, venous outflow should be maintained, and oxygen should be administered in addition to other precautions.9
Consequences of Pathologic Conditions
Aneurysms are usually asymptomatic and present no clinical problem to the patient unless rupture occurs, which results in neurologic deficits. A ruptured cerebral aneurysm is the major cause of subarachnoid hemorrhage or hemorrhagic stroke. Depending on the severity of the cerebral bleed, the rupture of a cerebral aneurysm can often be fatal.6 If treatable, surgical intervention usually involves clipping or coiling of the aneurysm after identification through angiography. Careful consideration is given to the complications that can occur after aneurysmal rupture or bleeding, which are rebleeding, vasospasm, and hydrocephalus.6
As mentioned previously, AVMs are usually present at birth, and patients may never experience symptoms until the AVM ruptures, which causes an intracranial hemorrhage and increased ICP. If symptoms do occur, they most commonly appear between the ages of 10 and 20 years. These symptoms may include headache, seizures, and altered level of consciousness (LOC). The treatment of choice is complete surgical excision via dissection or obliteration with ligation of feeder vessels. Radiation is used to treat AVMs that are not surgically accessible. Although AVMs are rare, the effect can be enormous and cause serious neurologic problems or even death.
Intracranial tumors can destroy brain tissue and nerve structures through invasion, infiltration, and compression. This typically produces an increased ICP. Clinical manifestations can be both localized and generalized in nature. Local pathophysiologic changes, such as focal neurologic deficits, seizures, visual disturbances, cranial nerve dysfunction, and hormonal changes, result from the tumor itself destroying tissue at a particular site in the brain. Generalized pathophysiologic changes result from the effects of increased ICP. The treatment for cerebral tumors is surgical excision or surgical decompression if total excision is not possible. Surgery is often performed before, during, or after radiation treatment and chemotherapy.
Hydrocephalus is not a disease entity but is rather a clinical syndrome characterized by excess fluid within the cerebral ventricular system, the subarachnoid space, or both. Hydrocephalus occurs because of abnormalities in overproduction, circulation, or reabsorption of CSF. Hydrocephalus can be classified into two categories: noncommunicating (obstructive) or communicating (nonobstructive). Noncommunicating hydrocephalus is the result of an obstruction in the ventricular system or the subarachnoid space that prevents the flow of CSF to the location of the arachnoid villi where reabsorption occurs. The obstruction can be caused by congenital abnormalities or space-occupying lesions. Communicating hydrocephalus occurs when the flow of CSF is normal, but absorption of the fluid at the arachnoid villi is impaired. Common causes of communicating hydrocephalus include inflammation of the meninges, subarachnoid hemorrhage, congenital malformation, and space-occupying lesions.
Intracranial Pressure Dynamics
The contents of the skull (brain tissue, CSF, and blood) are essentially not compressible. A volume change in any compartment requires a reciprocal change to occur in one or both of the other compartments if the ICP is to remain constant (Monro-Kellie hypothesis). The contents of the skull allow for partial compensation when increased ICP occurs. These compensation capabilities are limited because of the small amount of CSF that the spinal subarachnoid space can hold, and total displacement of cerebral blood results in cerebral ischemia. Normal ICP is 0 to 15 mm Hg. Intracranial hypertension occurs when a sustained increased ICP at the level of the head occurs and exceeds 15 mm Hg.
Volume may be added to any of the cerebral compartments and results in increased ICP when the compensatory capacity is exceeded. Brain volume can be increased by a tumor, a hematoma, or edema. Blood volume can be increased through dilation of the vascular bed. CSF volume can be increased through obstruction in the ventricles, resistance to reabsorption, or, in rare instances, increased production of the CSF. Large brain tumors can increase pressure by mass alone, by blocking CSF reabsorption, or by a combination of both. If the tumor is near the surface of the brain, it can cause inflamed meninges that may exude large quantities of fluid and protein into the CSF, thus increasing ICP. Hemorrhage or infection also causes increases in ICP. Large numbers of cells suddenly appear in the CSF and can almost totally block CSF absorption through the arachnoid villi. Regardless of the mechanism, when the volume added exceeds the volume that can be displaced, intracranial compliance is greatly reduced and ICP begins to increase.
Fig. 38.3 illustrates the relationship between intracranial volume and pressure. Phase I shows the success of compensatory mechanisms in maintenance of a constant ICP despite early increases in volume. In Phase II, the limited capability of compensatory mechanisms has been exceeded and ICP begins to rise. In Phase III, even a slight increase in volume causes a dramatic rise in ICP and thus results in complete decompensation and death. The shape of the curve may be altered by the rate at which the volume increases. Slowly developing increases in volume broaden the curve, whereas rapid increases narrow it.
Perianesthesia care for the patients with the potential for increased ICP requires an understanding of CBF and the factors that affect it; these factors become defective during increased ICP and are manipulated to reduce ICP. CBF is directly proportional to cerebral perfusion pressure (CPP) and inversely proportional to cerebrovascular resistance. CPP is described as the pressure required to perfuse the brain.10 CPP is typically expressed as the difference between the mean arterial pressure (MAP) and the ICP:
CPP=MAP− ICP
Consequently, any increase in ICP or reduction in MAP reduces CPP and resultant CBF. Average CBF is 50 mL per 100 g/min.3 The CBF below which cerebral ischemia occurs has been termed the critical CBF, which is a flow rate of 16 or 17 mL per 100 g/min. Average CPP is 80 mm Hg.3 CBF begins to fail at a CPP of 30 to 40 mm Hg.6 Irreversible hypoxia occurs at a CPP less than 30 mm Hg. When ICP equals MAP, CPP equals zero and CBF ceases.
Factors that influence CBF regulation are partial pressure of oxygen in arterial blood (PaO2) and partial pressure of carbon dioxide in arterial blood (PaCO2; metabolic regulation), arterial blood pressure and autoregulation, and venous blood pressure. Metabolic regulation works in two ways. The first is regulation of blood flow based on the tissue needs for metabolic substrates: oxygen and glucose. As the activity of neuronal and glial cells in the brain increases, the demand for oxygen and glucose increases. The increased demand causes vasodilatation of arterioles, which increases CBF. Likewise, if the metabolic demand decreases, vasoconstriction occurs and CBF decreases.
The second and most significant way metabolic regulation affects CBF is the presence of metabolic byproducts, specifically carbon dioxide. Carbon dioxide is the most potent vasodilator of cerebral blood vessels. Normal cerebral vessels respond to changes in carbon dioxide by dilating when carbon dioxide increases and constricting when carbon dioxide decreases. The relationship between CBF and carbon dioxide is linear, and changes in CBF are in direct proportion to changes in carbon dioxide. A decrease of 1 mL per 100 g/min in CBF occurs for every 1 mm Hg decrease in carbon dioxide. In the treatment of elevated ICP, carbon dioxide levels of 30 to 35 mm Hg are used to lower CBF.
Autoregulation is the ability of the cerebral vasculature in normal brain tissue to alter its resistance so that CBF remains relatively constant over a wide range of CPP. This mechanism causes vasoconstriction when perfusion pressure increases and vasodilation when perfusion pressure decreases. The limits of autoregulation are at a CPP of approximately 60 mm Hg at the lower end and 160 mm Hg at the upper end. Beyond the limits of autoregulation, CBF becomes passively dependent on CPP. When CPP increases to more than the upper limit of autoregulation, it exceeds the ability of the vasculature to constrict; CBF becomes directly related to and possibly dependent on CPP.
The lower limit of CBF autoregulation is the blood pressure below which vasodilatation becomes inadequate and CBF decreases. When CPP decreases to less than 60 mm Hg because of increases in ICP, autoregulation ceases to be beneficial or effective in regulation of CBF. Defective autoregulation aggravates pressure increases and creates critical or irreversible levels of ICP by increasing the blood volume within the cranium in an effort to maintain CBF. Defective autoregulation generally occurs when ICP exceeds 30 to 35 mm Hg. Eventually, autoregulation ceases altogether, and blood flow fluctuates passively with changes in arterial pressure regardless of metabolic activity or regulation.
When ICP is increased, CPP and CBF are reduced, which renders the tissues ischemic. Ischemic cerebral tissue releases acid metabolites that cause a relatively fixed reduction in cerebrovascular tone. Autoregulation ceases, and any increase in MAP causes a further increase in cerebral blood volume and elicits a further increase in ICP. CPP is reduced and thus causes ischemic areas to enlarge such as those that surround an expanding intracranial mass. As can be seen in Fig. 38.4, a pathologic cycle ensues in which ICP and MAP eventually equilibrate, the CPP drops to zero, CBF stops, and death occurs.
Neurosurgical Procedures
With further developments in technology, neurosurgeons have more options in the treatment of patients. Although nonsurgical treatment may be a possibility for some patients, surgical procedures that use instrumentation, lasers, and radiation therapy have increased the surgeon’s ability to treat neurologic disorders. Additionally, microneurosurgery, neuroendoscopy, and endovascular procedures are paving the way in treatment for specific neurologic conditions. These minimally invasive procedures offer treatment to patients who may not be candidates for open neurosurgery procedures.8 However, open cranium surgical procedures remain valuable to many patients as well.
Minimally Invasive Surgical Procedures
Depending on the injury or pathologic condition, patients may benefit from a minimally invasive procedure that is often performed in the operating room. Stereotaxis enables precise localization of a specified point. A stereotactic frame is applied to the patient’s head, and the target tissue is located with the stereotactic frame’s coordinates and CT scanning. Hemorrhage evacuation, catheter, shunt, or electrode placement and implantation of radioactive seeds are all procedures that may benefit from the use of stereotaxis. Additional uses include destruction of intracranial sensory pathways and the treatment of intractable chronic pain.9
Stereotactic radiosurgery (SR) can destroy deep and surgically inaccessible areas. The most common approaches to SR are gamma knife and medical linear accelerator units. The goal of SR treatment is the delivery of high-dose radiation to a specific target area without delivery of the radiation to surrounding tissue. Regardless of the type of SR, a stereotactic frame is secured to the patient’s head for accurate determination of target location. The placement of the stereotactic frame necessitates local anesthesia. Complications after SR may not appear for months to years later. Potential complications include permanent neurologic deficit, rebleeding, and worsening clinical symptoms.
The benefit of laser surgery is that it enables the neurosurgeon to access areas that were surgically inaccessible with conventional surgery. With laser surgery, the surgeon can dissect a structure without trauma to the surrounding tissue, shrink tumors, and coagulate blood vessels. A complete discussion on laser surgery is in Chapter 47.
New procedures are now available through techniques such as microneurosurgery where specialized microsurgical instruments can reach areas previously unreachable.8 Microsurgery has also enabled treatment of aneurysms through clipping and coiling as well as excisions of AVMs in addition to other cerebrovascular procedures. Endoscopes may also be used in neuroendoscopy procedures to control hydrocephalus or removal of tumors. The transsphenoidal approach is often used with an endoscope for access to pituitary tumors.8 Endovascular surgery is often used for diseases of the vasculature including cerebral ischemia and stroke.
Open Surgical Procedures
Craniotomies, craniectomies, and other open surgical procedures may be warranted as the patient’s condition and treatment are determined by the physician. If the skull must be accessed for brain tissue exposure, some form of cranial surgery is performed. This could be for tumor or lesion removal, hematoma evacuation, or other fluid/space-managing procedures. As mentioned previously, epidural hematomas may be evacuated through a burr hole made in the skull. Burr holes may also be made for instillation of medication, aspiration or drainage of CSF, or other subdural fluid removal.9 During a craniotomy, a bone flap is created for access to brain tissue. This may be necessary for access to lesions or tumors, evacuation of hematomas, aspiration of abscesses, or decompression of cranial nerves.8 Cranioplasties are performed if skull defects need to be repaired, and craniectomies are done if a portion of the skull needs to be permanently removed.9
Anesthetic Agents and Intracranial Pressure
Although the brain represents only 2% of the body’s total weight, it receives 12% to 15% of the total cardiac output because of the brain’s high metabolic rate.11 Anesthetic agents alter ICP by increasing or decreasing CBF and cerebral metabolic rate (CMR). In addition to the effects on ICP, some of these agents may also reduce systemic blood pressure and cause cerebral ischemia as a result of inadequate CPP.
Inhalation Anesthetics
The inhalation anesthetic agents generally decrease blood pressure and may increase ICP in the patient for cranial surgery. They produce a clinically significant degree of cerebrovascular vasodilation and metabolic depression and can modify autoregulation. In fact, high doses of volatile anesthetic agents can cause a total loss of autoregulation. The resulting increase in CBF ultimately leads to increased ICP. In patients with decreased intracranial compliance from neurologic disease, anesthetic agents that increase CBF may produce marked changes in ICP.
Isoflurane (Forane) at normocarbia has been shown to increase ICP. The initiation of hyperventilation simultaneously with the introduction of isoflurane prevents the increase in ICP that occurs at normocarbia. Isoflurane does not alter production of CSF and actually decreases resistance to absorption. It does not cause excitation of the central nervous system. Isoflurane produces reductions in CMR, and autoregulation is not impaired to any degree. The greater decrease in CMR may explain why CBF increases are minimal at low concentrations.
Desflurane and sevoflurane have essentially the same characteristics as isoflurane. During normocarbia, desflurane and sevoflurane cause cerebral vasodilation with resultant increases in CBF and ICP. The cerebrovascular response to carbon dioxide is maintained; therefore, the increase in CBF can be attenuated with hyperventilation. The CMR is reduced in a dose-dependent manner similar to that of isoflurane, and no neuronal excitation is seen. Both agents cause cardiovascular depression and can be used with caution for controlled hypotension to reduce CBF. Desflurane, unlike sevoflurane, can result in an increase in MAP and heart rate when used for induction or at initially high concentrations. This effect may be reduced with the use of opioids or beta blockers. Both desflurane and sevoflurane have relatively low blood–gas partition coefficients that allow for rapid elimination of the agent, which makes them desirable for use because of the more rapid recovery.
Nitrous oxide has become controversial for use during intracranial surgical procedures. When used alone, nitrous oxide can cause an increase in CBF, ICP, and CMR at normocarbia. These effects, however, are attenuated with the use of barbiturates, benzodiazepines, opioids, and hyperventilation. To a small extent, nitrous oxide is a cerebral vasodilator and does not interfere with autoregulation of the CBF. Nitrous oxide may be an acceptable choice when combined with other agents to benefit from its rapid onset and elimination for neurosurgical patients in whom increased ICP is not a problem; however, it should be used cautiously in patients with increased ICP.
Intravenous Anesthetics
A combination of inhalation and intravenous anesthetic agents may be used, but intravenous anesthetic agents (with the exception of ketamine) are usually the anesthetics of choice in cranial surgery. When neurologic assessment is necessary during the procedure, minimal sedation may be used if any at all. Anesthesia, the surgeon, and the entire health care team should work together to guarantee the appropriate plan for anesthesia is maintained.
Barbiturates, such as thiopental (Pentothal), are potent cerebral vasoconstrictors that can reduce CBF with subsequent reduction in elevated ICP. Cerebral vasoconstriction produced by barbiturates and the effect on CBF and ICP are dose related. The reduction in CBF produced by barbiturates is even greater if hypocarbia is also present and is maintained at a constant level. Deep thiopental anesthesia during normocarbia results in an approximately 50% reduction in both CMR and CBF.
Benzodiazepines, such as diazepam (Valium) and midazolam (Versed), produce sedation and amnesia by stimulating specific receptors in the brain. The benzodiazepines produce dose-related reductions in CMR and CBF. When central benzodiazepine receptors are pharmacologically saturated, these drugs can decrease CMR by as much as 40%.
Opioids, such as fentanyl, morphine, and meperidine, are typically classified as cerebral vasoconstrictors, with resultant reductions in CBF. This effect is readily abolished by vasodilation that can accompany opioid-induced ventilatory depression and the resultant increase in PaCO2. Inconsistencies do exist throughout the literature related to the actual effect CBF and CMR have on the unstimulated nervous system.11 During normocarbia, the combination of nitrous oxide and morphine does not significantly alter CBF or autoregulation. Fentanyl causes a reduction in CBF and ICP in patients with normal CSF pathways. Alfentanil and sufentanil may in fact cause increased ICP in patients with compromised cerebral compliance.
Etomidate (Amidate) produces a maximum 45% decrease in CMR and CBF; like the barbiturates, it can produce complete electroencephalographic suppression and appears to be comparable with lowering of ICP. Unlike the barbiturates, etomidate has less effect on MAP and offers greater stability in patients with hemodynamic compromise.
Propofol (Diprivan) reduces CMR, CBF, and ICP and increases cerebrovascular resistance in a dose-dependent manner. The use of propofol in patients with elevated ICP might not be appropriate because of the substantial decrease in MAP and resultant decrease in CPP. Although often used in rapid sequence intubation and continuous infusion during surgery, propofol has a short half-life and can therefore be used for sedation purposes in the neurosurgical patient; this facilitates the neurologic evaluation when needed.12
Dexmedetomidine (Precedex) is a sedative that allows for the patient to be easily aroused and often calm and cooperative while sedated; this enables the completion of the neurologic assessment. Although some precautions remain with this drug, respiratory and hemodynamic stability is typically maintained with dexmedetomidine.6,13
Ketamine (Ketalar, Ketaject) can rapidly increase ICP and often reduce CPP despite mild increases in blood pressure. Ketamine is generally contraindicated for use in neurosurgical patients unless the fontanels are open, CSF aspiration is instituted, or ventilatory control is maintained.
The use of controlled ventilation to produce PCO2 levels in the range of 30 to 38 mm Hg and administration of nitrous oxide and oxygen and possibly low concentrations of isoflurane, together with opioids and muscle relaxants, is a generally accepted anesthetic technique for the neurosurgical patient.14
Adjunctive Drugs and Interventions Used to Reduce Intracranial Pressure
Diuretics
Mannitol and furosemide (Lasix) are diuretics often used to control increased ICP. Mannitol, an osmotic diuretic, is the agent of choice for intracranial hypertension because it decreases intracellular edema.6 Appropriate infusion of crystalloid and colloid solutions is often necessary to prevent adverse changes in plasma concentrations of electrolytes and intravascular fluid volume because of the rapidity of diuresis. Potential complications of the use of mannitol include hyperosmolarity, electrolyte loss, changes in blood viscosity and coagulation, transient intravascular hypervolemia, and rebound or secondary elevation of ICP.
Whereas mannitol reverses edema, furosemide is a loop diuretic and is more effective in decreasing circulating blood volume and thus reducing edema.5 Furosemide is more effective than mannitol in reducing ICP and is the drug of choice in patients with congestive heart failure.
Furosemide, when combined with mannitol, has been shown to potentiate the ICP-reducing effects of mannitol at the cost of rapid loss of intravascular volume and electrolytes. The ICP effects of these drugs are lost after 1 or 2 hours. Patient monitoring should consist of the diuretic effects and need for additional medication.
Corticosteroids
The drugs most commonly used are dexamethasone and methylprednisolone. Steroids are effective in lowering increased ICP because of localized vasogenic cerebral edema associated with mass-type lesions such as neoplasm, abscess, and intracerebral hematoma. This is best accomplished when the steroid can be administered 48 hours before surgery.11 Steroid administration is then continued intraoperatively and postoperatively for the patient to continue to have the effects of decreased ICP.11
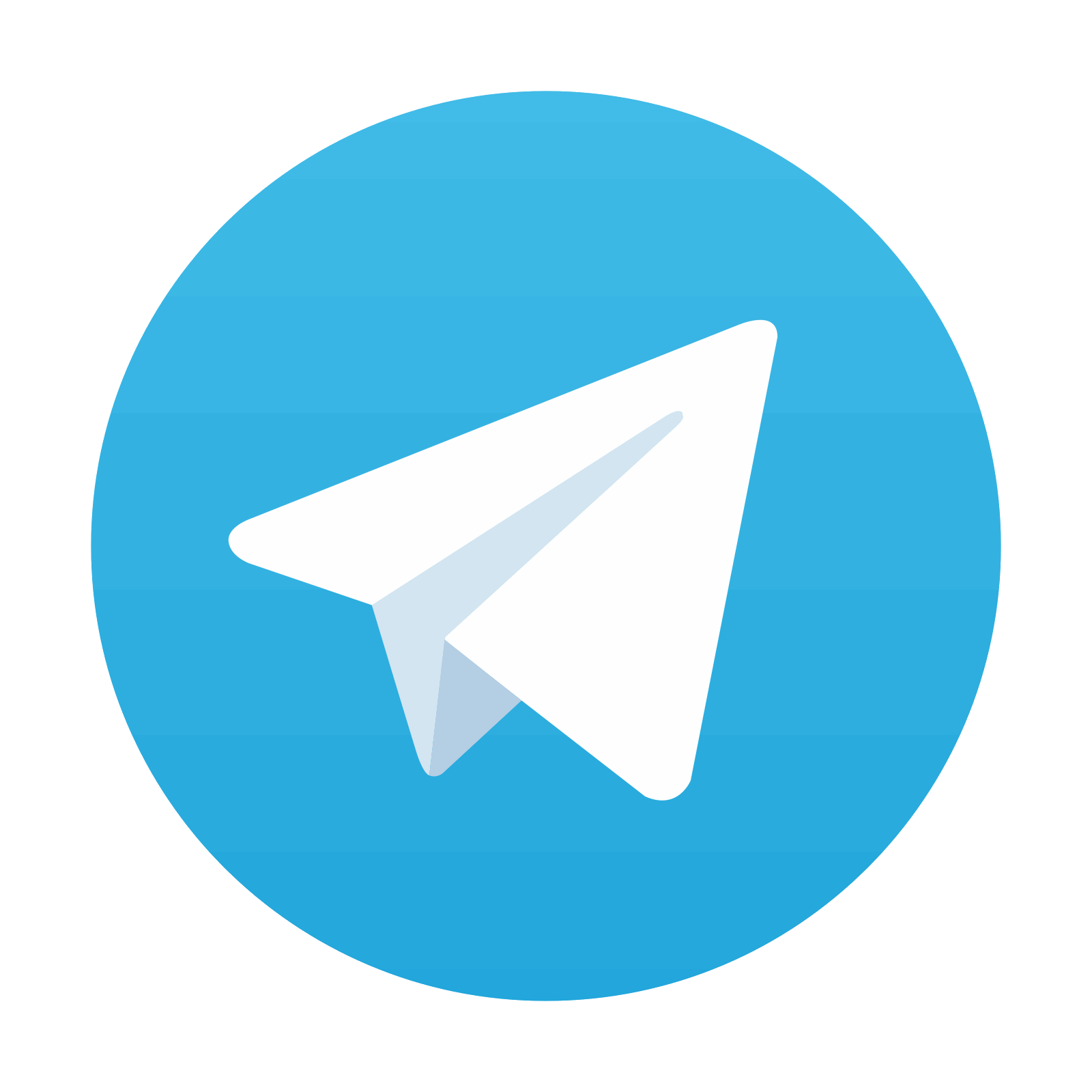
Stay updated, free articles. Join our Telegram channel

Full access? Get Clinical Tree
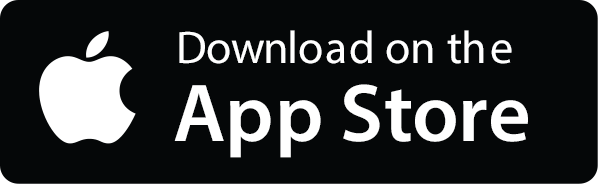
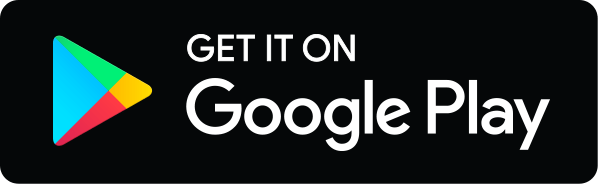