FIG. 55.1 Normal intracranial pressure waveform. (From McQuillan KA, et al.: Trauma nursing: from resuscitation through rehabilitation, ed 4, St. Louis, MO, 2009, Elsevier.)
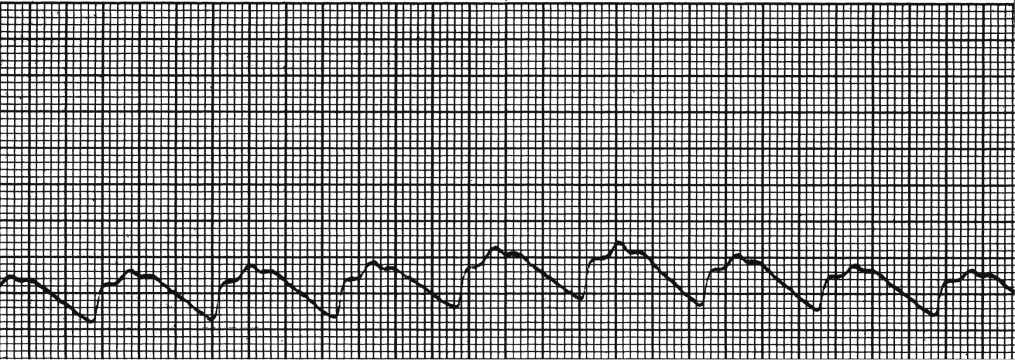
FIG. 55.2 Abnormal intracranial pressure waveform. P2 is higher than P3, which is an indication of poor cerebral compliance. (From McQuillan KA, et al.: Trauma nursing: from resuscitation through rehabilitation, ed 4, St. Louis, MO, 2009, Elsevier.)
CSF is a clear colorless fluid that fills the ventricles of the brain and subarachnoid spaces of the brain and spinal cord. Most CSF is produced by the choroids plexus, which are located in the third and fourth ventricles of the brain. CSF is constantly produced at a rate of approximately 25 mL/h, or 500 mL/day.27 When the pressure within the brain exceeds 15 mm Hg, CSF production may slow or the brain displaces CSF to accommodate increases in brain tissue edema. Additional interventions may be the active removal of CSF through an EVD when the ICP exceeds a certain ICP parameter.
Cerebral blood flow (CBF) is the third component of ICP dynamics. The brain receives approximately 20% of the cardiac output and consumes 20% of the body’s oxygen and 25% of the body’s glucose.25 When pressures within the cranial vault increase beyond normal ranges, the heart has more difficulty delivering nutrients to the brain. Factors that decrease cardiac output and function, increased blood viscosity, and hypotensive states significantly compromise effective cerebral blood flow. Cerebral vasculature is also influenced by changes in carbon dioxide levels in the blood. High levels of carbon dioxide (i.e., PaCO2) cause cerebral vasculature dilation and increase blood flow, and decreased levels of PaCO2 cause vasoconstriction of cerebral vasculature and decrease blood flow. However, reduction of PaCO2 to less than 35 mm Hg reduces blood flow to the brain tissue and may exacerbate cerebral ischemia, further increasing ICP.25,28 During states of elevated ICP, PaCO2 may be artificially lowered through mechanical ventilation settings that cause slight hyperventilation to induce cerebral vasoconstriction. However, caution to avoid hyperventilation that lowers PaCO2 less than 35 mm Hg is warranted to avoid worsening cerebral ischemia causing a secondary cerebral insult.28 End-tidal carbon dioxide (ETCO2) monitoring may be used to trend and more continuously assess PaCO2 during mechanical ventilation.28
Autoregulation refers to the ability of the brain to maintain a constant CBF despite changes in the arterial perfusion pressure (systemic circulation). Autoregulation is a protective homeostatic mechanism of the brain that attempts to keep cerebral perfusion constant and generally continues to maintain CBF until the ICP exceeds 40 mm Hg.25 Autoregulation provides a constant CBF by adjusting the diameter of blood vessels based on changes in the intracerebral pressure. It works synergistically with other protective mechanisms of the brain (e.g., reducing PaCO2 and displacing CSF) to maintain CBF. Autoregulation, however, is limited as a compensatory mechanism. A critical point can be reached because of sustained increases in ICP, global or local diffuse injury, cerebral edema, ischemia, or inflammation.25 If autoregulation is lost, reduced cerebrovascular tone occurs and the CBF becomes dependent on changes in systemic blood pressure. Therefore, a primary goal in management of ICP is effective treatment of the cause of the increase in ICP so autoregulation is maintained as a compensatory mechanism of CBF.
CPP is a parameter calculated from the mean arterial pressure (MAP) minus the ICP and is an indicator of general cerebral perfusion and CBF. CPP becomes increasingly important when the patient loses autoregulatory homeostasis because of sustained increases in ICP. The minimal CPP necessary to maintain adequate perfusion is 50 to 70 mm Hg.22–25 The optimal CPP remains an area of great controversy, and the mechanism of cerebral injury may influence ideal CPP for brain tissue perfusion. Patients with traumatic brain injury may tolerate a CPP of 50 to 70 mm Hg; however, patients with other etiologies (e.g., cerebral hemorrhage) may require a higher CPP threshold.23,25,29 Because CPP is dependent on MAP, interventions to increase MAP may be necessary if ICP cannot be lowered to maintain effective cerebral perfusion during states of increased ICP. Vasoactive agents to support cardiac output and blood pressure are frequently administered to increase CPP to meet cerebral perfusion demands.
Newer technology can be introduced invasively into the brain and cerebral vasculature to measure brain tissue oxygenation (PtbO2). A brain tissue oxygen probe can be inserted through an intracranial bolt or tunneled and provides information that reflects brain tissue oxygenation associated with cerebral oxygen demand and systematic oxygen delivery.30 Neuromonitoring with microdialysis catheters can be used to identify clinical events that precede clinical examination changes.31,32 A cerebral microdialysis catheter can be inserted through an intracranial bolt and allows sampling of cerebral substances within the interstitial fluid to evaluate the brain metabolism markers (e.g., glucose, lactate, pyruvate, glutamate, glycerol). Evaluation of these markers along with clinical assessment can assist with interventions to prevent secondary brain tissue injury from elevated ICP.31,32
Transient increases in ICP are dynamic temporary increases in ICP. They can be caused by coughing, pain, or excessive stimulation and are associated with cerebral hypoxemic and ineffective cerebral perfusion states.25 Signs and symptoms of transient increases in ICP of more than 15 mm Hg include headache, aphasia, changes in respiratory pattern (e.g., Cheyne-Stokes), changes in vital signs, decreases or changes in level of consciousness, motor dysfunction (e.g., hemiparesis), visual disturbances, and nausea and vomiting.25 In addition, sudden diuresis may indicate a dysregulation of antidiuretic hormone related to ICP.
Consequences of increased ICP can be more devastating than the initial neurologic insult.25,29 Nursing interventions need to focus on assessing risk factors for increases in ICP and implementing and monitoring the effects of interventions to reduce sustained increases in ICP.
Postanesthesia Management of the Neurosurgical ICU Patient
Postanesthesia management of the intensive care neurosurgical patient focuses on minimization of increases in ICP and continuous assessment of neurologic status. The goal of nursing care is to minimize secondary insults that are a consequence of ICP. A focused neurologic assessment to include level of arousal, orientation, and motor skills and a cranial nerve examination should be completed hourly for up to 12 hours or longer depending on the nature of the neurosurgical procedure and patient’s ICP challenges. The nurse needs to assess for subtle changes in the neurologic assessment rather than looking for gross deviations from normal. Knowledge of the patient’s neurologic assessment before surgery is important in establishing a baseline from which to base the immediate postoperative neurologic assessment. Another important variable in neurologic assessment is the nurse. Nurses should validate abnormal findings with each other because subtle changes may be hard to assess. Nurses should also perform a neurologic assessment with each change of shift to ensure maximized consistency in the assessment of the neurosurgical patient. Basic assessments of cerebral dressings after surgery with observation for bleeding or presence of CSF fluid leakage are also part of the immediate perianesthesia nursing assessment. If the neurosurgical procedure was related to spinal pathology, the nursing assessment focuses on motor and sensory function of the patient. Management or prevention of edema and maintenance of body alignment remain the nursing priorities in the immediate postanesthesia period.
Monitoring of ICP, documentation and assessment of the ICP waveform at set intervals, and correlation of the neurologic assessment during elevations in ICP are important nursing assessment interventions. Immediate interventions to reduce ICP include elevating the head of the bed 30 degrees and maintaining a neutral neck alignment.21 If noxious stimuli, environmental stimuli, or nursing activities are the cause of increase in ICP, limiting nursing interventions and noxious events such as venipuncture, suctioning, and nursing cares should be considered. When interventions do need to occur, consider clustering interventions, less than six interventions, to minimize stress to the patient while monitoring ETCO2 and ICP.33
Maximization of oxygenation and ventilation is an important intervention in the care of neurosurgical patients. Interventions to maximize oxygenation to achieve a PaO2 greater than 60 mm Hg and normal PaCO2 are important to ensure that cerebral oxygen demands are met and cerebral vasoconstriction from hypocarbia is minimized.
Maintenance of an effective blood pressure and cardiac output to meet cerebral perfusion needs also is a primary nursing intervention. Medications to lower or raise blood pressure to optimize CPP are ordered for the neurosurgical patient. Immediately after surgery, the physician may want the blood pressure lower to minimize bleeding. If the neurologic examination becomes compromised when the blood pressure is lower, the nurse should notify the physician because this may be an indication of ineffective cerebral perfusion. Depending on the neurologic insult, the nurse either administers medications to lower the patient’s blood pressure to prevent further intracerebral bleeding or administers medications to increase the patient’s blood pressure to maximize cerebral perfusion and manage cerebral edema. Knowledge of the patient’s pathology, neurosurgical procedure, and neurologic examination results is a crucial assessment variable to help determine optimal blood pressure parameters.
Management of pain and sedation is an important nursing intervention during the perianesthesia care of the neurosurgical patient. Short-acting analgesics and sedation agents should be used to allow continued assessment of the patient’s neurologic status. Maintaining normothermia or inducing mild hypothermia (core temperature, 33° C to 36° C) and aggressively treating fever have been found to be neuroprotective in patients with cerebral injury.24,34 For every decrease in temperature below normal, brain metabolism decreases by 7% to 10%.25,34 Body temperature can be maintained or lowered with conventional air sources, cooling blankets, or intravascular devices. Regardless of the method used to maintain normothermia or mild hypothermia, interventions should not induce shivering. Shivering can adversely increase metabolic demand and oxygen consumption needs beyond the benefits of lowering the patient’s body temperature.
The research on the negative effects of hyperglycemia in critical illness continues to mount. Whereas hyperglycemia can cause adverse patient outcomes, rigorous insulin regimens to maintain tight control of serum glucose can result in hypoglycemia; even transient hypoglycemia can have detrimental effects on patient outcome.35,36 Current guidelines recommend a slightly higher serum glucose during critical illness (140 to 180 mg/dL) as studies have found attempts for tighter glucose control (e.g., 70 to 110 mg/dL) resulted in transient hypoglycemic events.35,36 Critical illness increases the secretion of counterregulatory hormones such as glucagon, epinephrine, norepinephrine, and growth hormone; it also results in an increase in hepatic glucose production, decrease in peripheral glucose uptake, and induction of a hyperglycemic state. The hyperglycemia of critical illness is initially an adaptive response to stress; however, over time it exacerbates the circulation of abnormal inflammatory mediators and worsens states of tissue ischemia.37 Glucose is a primary substrate for energy in the brain, and states of both hypoglycemia and hyperglycemia have been found to worsen cerebral perfusion.36 Therefore, efforts to maintain normoglycemic states (serum glucose, 110 to 180 mg/dL) by administering intravenous insulin either intermittently or via continuous infusion are indicated in the management of the critically ill neurosurgical patient.36
Care of the critically ill neurosurgical patient after surgical interventions requires that the nurse have a working knowledge of neuroanatomy, the surgical procedure, and monitoring of the patient for subtle changes in neurologic assessment and neurologic hemodynamics. Assessment of subtle changes in the neurologic assessment and correlation of changes to vital sign parameters and neurohemodynamics are essential in the treatment of critically ill neuroscience patients. Simple nursing interventions such as maintaining neutral head alignment, preventing shivering, and maintaining the head of the bed at more than 30 degrees are effective first-line interventions in the treatment of patients with altered ICP. Other interventions focus on maximization of oxygenation, cardiac output, blood pressure, and prevention of infection. Technical knowledge related to ICP monitoring and ventriculostomy management is necessary to effectively monitor and treat changes in ICP.38 Finally, involving the family and ensuring that they understand goals of care and interventions are important so that family-centered care is maximized throughout the patient’s acute illness and recovery phase.
Postoperative Care of the ICU Burn Trauma Patient
Unintentional deaths from fire and burns are estimated at 2855 deaths from residential fires, 300 from vehicle crash fires, and 85 from other sources such as contact with electricity, chemicals, or hot liquids.39 More than 400,000 individuals require medical treatment related to burn injuries with up to 60% requiring acute hospitalization.39 A small percentage of burn-injured patients do not survive (approximately 4%); these patients have associated inhalation injury.40 However, in the face of these sobering facts, the overall mortality and morbidity rates from burn injury have declined over the years because of advances in burn prevention strategies and medical interventions for this patient population. Elements that have been attributed to patient survival include more rapid response by emergency teams, efficiencies in transport to burn treatment facilities, advances in fluid resuscitation, improvements in wound coverage, better support of the hypermetabolic response to injury, advances in infection control practices, and improved treatment of inhalation injuries.40,41
Patients with burn injury have special needs throughout hospitalization. The American Burn Association (ABA) has established guidelines to determine which burn-injured patients should be referred and transferred to a specialized burn center to maximize treatment and decrease patient morbidity and mortality (Box 55.3).42 Patients who meet the criteria outlined by the ABA should be transported to the nearest burn center to maximize patient survival and functional outcome.
Brief Review of Burn Injury Pathophysiology
Burn tissue injury is associated with the coagulation of cellular protein caused by exposure or contact with heat produced by thermal, electrical, chemical, or radiation energy. The depth of coagulative tissue necrosis (depth of burn wound) depends on the intensity of the heat and length of time the tissues are exposed to the heat source. Thermal injury from flame, steam, scald, and contact with hot objects is the most frequent cause of burn injury. Inhalation injury is frequently associated with thermal injury when the victim is trapped in an enclosed space during the fire. Electrical injury occurs when electrical energy is converted into heat and causes tissue destruction as the current flows through the body. Electrical current travels through the body along a path of least resistance such as nerves, blood vessels, and muscles, sparing the skin except at the entry and exit points of the current and resulting in deep internal tissue damage.41,43 Chemical injuries from either acidic or alkaline agents cause tissue destruction related to the type, strength, and duration of contact. Radiation burns are infrequent and usually are the result of medical radiation treatments or industrial accidents.
Regardless of what caused the burn injury, the tissue damage can be conceptualized as having three zones that represent the depth of tissue coagulation. Full-thickness burn is the deepest tissue injury in which full coagulation of the tissue proteins has occurred and causes irreversible tissue necrosis. Immediately surrounding the necrotic tissue area is the region or zone of stasis in which blood flow is impaired.41,43 This region is considered a critical area because it can progress to tissue necrosis if tissue perfusion is inadequate during the burn fluid resuscitation period, which creates a larger burn wound injury. The outer zone of hyperemia has sustained minimal tissue injury and usually heals rapidly. Early goals of burn management focus on stopping the burning process and providing adequate fluid resuscitation to prevent the extension of burn injury from lack of perfusion.
When burn injury occurs, myriad local mediators are released by the body in response to the tissue insult. These mediators—such as histamine, prostaglandins, thromboxane A2, kinins, oxygen radicals, platelet aggregation factor, complement cytokines, interleukins, and catecholamines—cause arteriolar and venule dilation, increased microvascular permeability, and decreased perfusion.41,44 Proteins leak from the intravascular space into the extravascular space and increase tissue oncotic pressure, creating edema.41 Thromboxane A2, a mediator that causes vasoconstriction, is also released by the body and may compromise perfusion to the burn injury area and cause extension of the depth of burn tissue injury.41,44 Concurrently, the coagulation system is activated, which causes platelet aggregation and activation of polymorphonuclear neutrophil leukocytes and macrophages, which are essential to wound healing. The summation of the activation of the body’s intense inflammatory response is vascular stasis and rapid formation of tissue edema. Edema places the patient at risk of developing intraabdominal hypertension (IAH) that can progress to life-threatening abdominal compartment syndrome.41,45 Measurement of intraabdominal pressure via bladder pressure monitoring may be initiated to evaluate the development of IAH.45 Extensive edema also compromises intravascular fluid volume, creating osmotic and hydrostatic pressure changes, often necessitating continued fluid resuscitation to meet tissue perfusion needs that exacerbates edema formation and creates a clinical challenge for optimal fluid volume replacement that minimizes edema formation.
Effective fluid resuscitation is an initial priority to prevent burn shock and progression of tissue injury. Several formulas can be used to guide fluid resuscitation needs based on the patient’s total body surface area (TBSA) injured. One of the more common formulas used to calculate fluid volume resuscitation is the Parkland formula (Box 55.4). Regardless of the formula used, the goal is to support the circulatory system throughout the initial 24 to 48 hours after the burn injury.41 Fluid shifts are significant during early resuscitation, requiring ongoing evaluation of tissue perfusion (e.g., heart rate, blood pressure, urine output, serum lactate levels) and assessment of complications from edema formation (e.g., IAH and compartment syndrome). Optimal fluid for resuscitation remains a topic of significant research. Fluids for resuscitation include crystalloids (typically lactated Ringer solution), and colloids may be added after 12 hours from initiation of resuscitation (e.g., albumin, fresh frozen plasma).41 A critical balance is needed to provide optimal fluid resuscitation to meet tissue and organ perfusion needs without inducing secondary complications associated with overresuscitation and edema.
In addition, the extensive loss of tissue and the exaggerated physiologic inflammatory and stress response associated with the burn injury place the patient at risk for infection, hypothermia, hypercatabolism, and development of acute lung injury (ALI), sepsis, systemic inflammatory response syndrome (SIRS), acute kidney injury (AKI), and multiple organ dysfunction syndrome.41,43 The intensity of the hypermetabolic changes experienced by burn patients is directly related to the extent of injury.46 Metabolic demands increase by an estimated 30% in patients with an injury that covers 20% of the TBSA or more and by 100% in patients with burns that cover 50% of the TBSA or more.46 Meeting nutritional goals is essential because 10% loss of total body mass leads to immune dysfunction, 30% leads to decreased wound healing, and 40% loss leads to death.46 Efforts to attenuate the hypermetabolic response in critically ill burn patients may include beta-adrenergic blockage with propranolol.46 Other pharmacologic anticatabolic therapies include growth hormone, insulin-like growth factor, intensive insulin therapy, and oxandrolone.46
Primary goals in the management of a patient with burn injury focuses on: (1) providing adequate fluid resuscitation to restore circulating volume and minimize the conversion burn tissue injury to deeper full-thickness tissue injury, (2) optimizing tissue oxygenation and management of associated pulmonary insults, (3) preventing hypothermia, (4) preventing infection though topical antibiotics and excision and coverage of burn wounds, (5) maintaining nutritional support the immune system and maximize wound healing, and (6) effectively treating pain and emotional needs of the patient.
Extent and Depth of Tissue Injury
Burn injuries are described according to the extent and depth of tissue injury. Extent is the TBSA that has been injured, and depth is the severity of tissue necrosis. The Rule of Nines is a quick and easy method of estimating TBSA (Fig. 55.3). Using this method, the body is divided into seven areas that represent 9% or a multiple of 9% of the body surface area, with the remaining 1% representing the genitalia. This method is most frequently used in the emergency room or upon initial assessment of the burn-injured patient to estimate TBSA needed in calculated fluid resuscitation needs for the patient.43 When the patient is in the acute care setting, the percentage of TBSA is more precisely calculated with the Berkow and Lund-Browder formulas.47,48 This assessment tool is used to estimate the amount of tissue injured by the thermal agent. The primary goal in estimating the TBSA of burn injury is to predict morbidity or survival, physiologic response in relation to fluid shifts, fluid resuscitation requirement, and metabolic and immunologic responses.
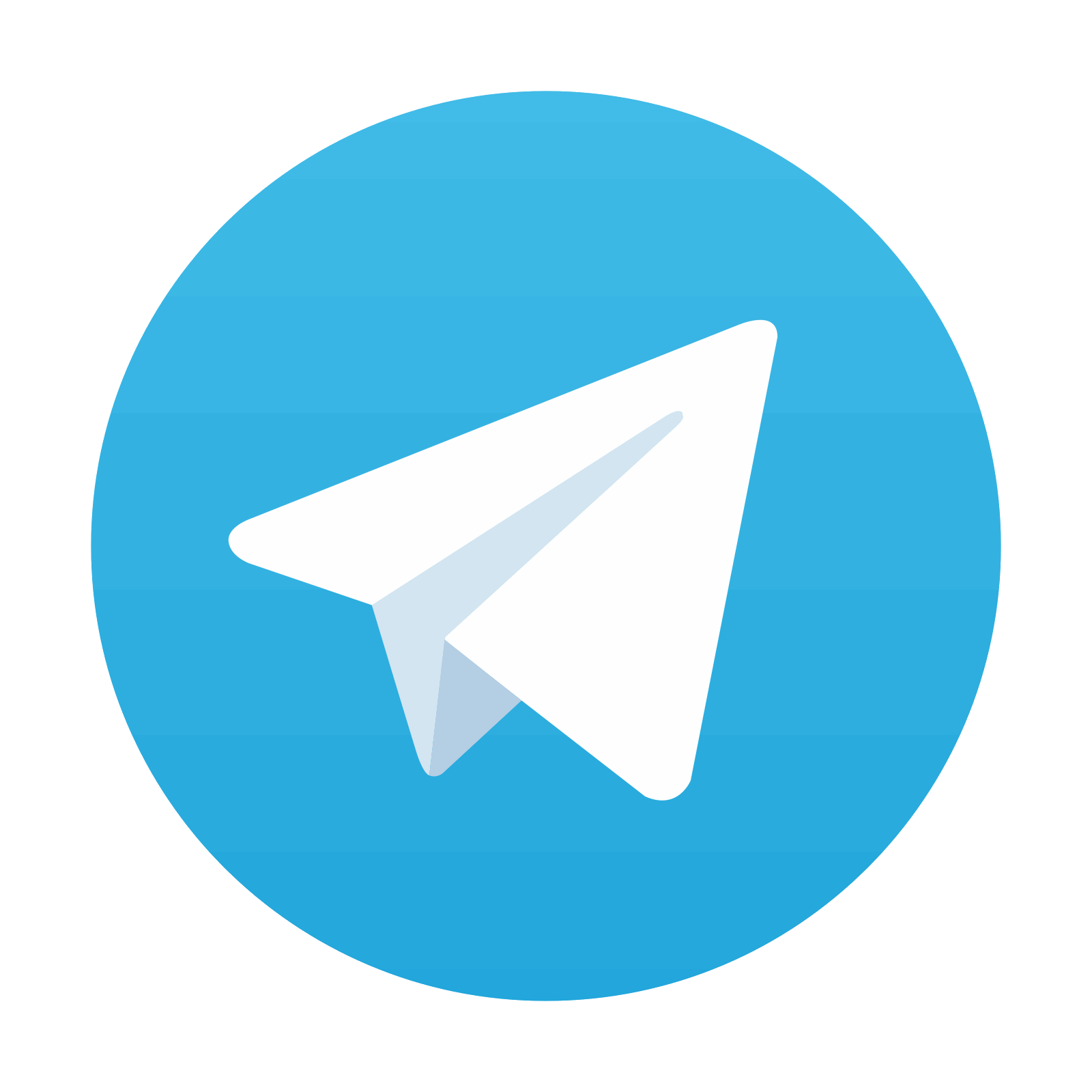
Stay updated, free articles. Join our Telegram channel

Full access? Get Clinical Tree
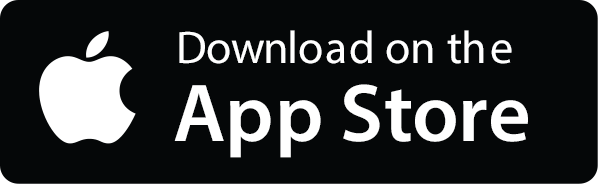
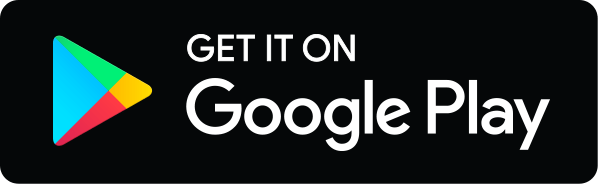