The ST changes are new when compared with a prior ECG. You place a pulmonary artery (PA) catheter through an existing introducer in order to assess her cardiac function. Her PA pressures are elevated at 53/37 mm Hg with a cardiac index (CI) of 2.1 L/minute/m2, mixed venous oxygen tension (SvO2) of 60%, pulmonary artery occlusion pressure (PAOP) of 32 mm Hg, and mean arterial pressures (MAPs) in the 50 to 55 mm Hg range. Intraoperative transesophageal echocardiography (TEE) shows an area of marked left ventricular (LV) apical akinesis with severely depressed LV systolic function (ejection fraction [EF] 20% to 30%) and restrictive LV filling.
What is her differential diagnosis? What are the proposed mechanisms of neurogenic stress cardiomyopathy and Takutsubo cardiomyopathy? How can you differentiate between them? Which medications can you use to improve her cardiac function?
The neurosurgeon successfully coils the aneurysm, and you have started a dobutamine infusion. Her CI improves to 3.1 L/minute/m2; SvO2 is now 68%; and her MAPs have been stable, giving her an adequate CPP. The neurosurgeon asks about the evolution of neurogenic stress cardiomyopathy and whether it will affect their management if she were to develop vasospasm in the next few days.
What types of therapies will she receive in the prevention of vasospasm? How will this affect her cardiac function? Which medications can be used to increase her systemic vascular resistance (SVR) in this setting? Is a suspected neurogenic stress cardiomyopathy reversible?
Chapter Highlights
Oxygen delivery to the brain is of utmost importance in the perioperative management of neurosurgical patients. Optimization of cerebral perfusion pressure (CPP = mean arterial pressure [MAP] − intracranial pressure [ICP]), cerebral blood flow (normal CBF is 50 mL/100 g/minute), and the various components affecting cardiac output should be the focus of therapy.
Hemodynamic goals for optimizing therapy include: MAP ≥ 80 mm Hg, CPP > 65 to 70 mm Hg, mixed venous saturation (SvO2) ≥ 65%, CI > 2.5 L/minute/m2, and urine output (UOP) > 0.5 mL/kg/hour.
Vigilant maintenance of an appropriate MAP is key. In the absence of significant pre-existing HTN or decreased intracranial compliance, a 20% decline from baseline is usually well tolerated.
Vasoactive drugs are commonly used. Most do not affect cerebral vascular tone directly but can have varied indirect effects on CPP, CBF, and cerebral metabolic rate for oxygen consumption (CMRO2).
Although sodium nitroprusside (SNP) and nitroglycerin (NTG) can provide rapid and reliable vasodilatation, the risk of increasing CBF, especially in the setting of elevated ICP, may preclude their use. Calcium-channel blocking drugs such as nicardipine and clevidipine have proven to be safe, effective, and comparably fast acting in such scenarios.
Subarachnoid hemorrhage (SAH) and its complications can have varied effects on the cardiovascular system. These include neurogenic stress cardiomyopathy and cerebral vasospasm, along with their hemodynamic sequelae and respective therapies.
The management of the cardiovascular complications of perioperative neurologic disorders, such as autonomic dysfunction and reflexive blood pressure (BP) fluctuations, focuses on tight BP control and maintenance of the hemodynamic goals as mentioned previously. Knowledge of the potential complications specific to each disorder and its treatment is warranted.
I. BASIC CONSIDERATIONS
The primary goal of cardiovascular therapy in perioperative neurosurgical patients is to provide sufficient metabolic substrate to the threatened central nervous system (CNS). Because glucose is usually not a problem related to cardiovascular therapy, our attention is directed to oxygen delivery. Cardiovascular therapy can affect intracranial volumes and pressures both globally and locally and result in irreversible injury. Therefore, while maintaining oxygen delivery is important, care must be exercised not to compromise intracerebral hemodynamics. The following sections will illustrate the heart-brain paradox, which is an ever-present challenge that deserves much consideration in the realm of neuroanesthesia.
A. Autoregulation refers to the interdependence of CBF and mean arterial (blood) pressure (MAP). The range of MAP over which non-hypertensive patients exhibit autoregulation is 50 to 150 mm Hg. Chronically hypertensive patients have altered autoregulation and neither maintain CBF at a MAP in the low end of the normal range nor vasoconstrict adequately when the MAP exceeds 150 mm Hg. A similar alteration of autoregulation occurs with sympathetic activation induced by shock or surgery.
1. When autoregulation is impaired, CBF will become more dependent on the BP; minor swings in MAP might have significant impact on intracranial hemodynamics.
2. Many conditions such as head injury and tumors are associated with either generalized or regional disruption of autoregulation.
B. Intracranial pressure (ICP). The intracranial compartment is essentially a closed space, so ICP is determined by the intracranial volume, which is comprised of cerebrospinal fluid (CSF), cerebral parenchyma, and cerebral blood volume (CBV). Increases in any of these components can contribute to intracranial HTN and result in decreased CPP, the difference between MAP and ICP. Daily CSF production, for example, is fairly constant, as is its volume. Any obstruction to CSF flow or resorption (e.g., tumor, intraventricular hemorrhage, etc.) will result in elevated CSF volumes, and in turn, elevated ICP.
C. CPP is the difference between MAP and ICP. In most organs, venous pressure generates the back pressure that determines the pressure drop across an organ. Because venous pressure tends to be negative in the brain, parenchymal pressure (ICP) generates the back pressure for determining CPP. CPP determines CBF both globally and regionally in the CNS. Normal CBF is 50 mL/100 g brain/minute and decreases only if CPP falls from a normal value of approximately 80 mm Hg to below 50 mm Hg unless autoregulation is altered.
II. CEREBRAL OXYGEN DELIVERY is a function of CBF and the quantity of oxygen carried in arterial blood (CaO2). CBF is related to CPP, and, because BP is a function of cardiac output (CO) and SVR, CO becomes an important parameter to manipulate to preserve CBF and cerebral oxygen delivery. The normal awake brain consumes 3.3 mL O2/100 g/minute, a value reduced by approximately one-third during the administration of anesthesia.
A. Cardiac output
1. Determinants of CO. The CO is a result of five basic factors: preload; contractility; heart rate (HR); rhythm; and afterload—assuming normal valvular function and the absence of obstructive cardiac pathology.
a. Preload is difficult to monitor but can be best determined by measuring ventricular end-diastolic volume (EDV) by either angiography or echocardiography. Surrogates for preload volume are CVP as a measure of right atrial preload and PAOP as an estimate of left atrial preload. In addition to PAOP, the volumetric PA catheter monitoring measures right ventricular EDV for preload assessment. Stroke volume variation and pulse pressure variation can be used to assess preload and volume responsiveness when pulse contour cardiac monitoring technology is employed.
b. Contractility. The ejection fraction measured by either angiography or echocardiography is frequently used to assess contractility, which can also be assessed by evaluating the magnitude of the CO for a given preload condition. Right ventricular EF is available when monitoring is performed with a volumetric PA catheter. CO equals the product of HR and stroke volume. When preload, contractility, or both limit stroke volume, an increase in HR often acts to maintain CO.
c. Rhythm. Rhythm disturbances can result in stroke volume variability, and should be treated promptly and appropriately. An adequate and effective stroke volume depends on coordinated contraction of both the atria and ventricles. This variability can negatively affect the accuracy of most monitoring systems, including thermodilution CO and pulse contour analysis techniques.
d. Afterload. Afterload is difficult to measure by non-invasive means. Surrogates employed are MAP, which is the afterload against which the left ventricle ejects a stroke volume, and SVR index (SVRI), which can be calculated while using a PA catheter or pulse contour monitoring.
B. Cardiac failure occurs when CO is insufficient to deliver adequate oxygen. Typical causes include cardiomyopathies from infarction, ischemia, and chronic HTN; dysrhythmias; increased afterload from arterial HTN; and outflow obstruction from pulmonary embolus. Neurosurgical patients who are critically ill have additional considerations.
1. Hypovolemia resulting in insufficient preload can be caused by
a. Blood loss from surgical and traumatic causes including scalp lacerations
b. Osmotic diuretic administration
c. Fluid restriction to minimize cerebral swelling
d. Diabetes insipidus associated with severe traumatic brain injury (TBI) and pituitary surgery
2. CNS causes
a. Spinal cord injury and injury to the brain stem vasomotor center can result in hypotension from decreased sympathetic tone.
b. Neurogenic stress cardiomyopathy has been described in patients after they have suffered SAH and acute TBI. The leading theory as to its etiology postulates the massive release of catecholamines at the time of injury. The resulting myocardial wall stress secondary to increasing BP and HR can lead to cardiac injury, and the resultant coronary vasospasm has been suggested as a cause of a reversibly “stunned” myocardium. Takutsubo cardiomyopathy can also present in this fashion, but usually has a more distinct apical distribution to the wall motion abnormalities. Its etiology is similarly linked to a high catecholamine state.
Patients can have disturbances in cardiac biomarkers, as well as echocardiographic evidence of wall motion abnormalities. The ensuing ECG changes are consistent with ischemia and myocardial dysfunction. The most common changes described include ST-segment abnormalities, deeply inverted T waves, prominent U waves, and prolongation of the QTc interval. Dysrhythmias, especially within the first 48 hours of injury, can include atrial fibrillation, sinus tachycardia, ventricular tachycardia, ventricular fibrillation, and sinus bradycardia. The frequency and severity of both rhythm disturbances and morphologic ECG changes have been shown to correlate with the severity of neurologic injury. Awareness of these abnormalities, which can commonly occur within the first 72 hours of injury, and recognition of their neurogenic origin and reversibility are integral to the determination of appropriate cardiac management.
3. Drug induced. Occasionally, aggressive therapy with calcium-entry blocking drugs or beta-blocking drugs can result in suboptimal myocardial performance.
C. Blood pressure
1. MAP = CO × SVR. CO is discussed in the preceding text.
2. Hypotension in the presence of normal intravascular volume and cardiac function occurs because of decreases in SVR.
a. Hypotension can be secondary to high cervical cord injury.
b. Brain stem damage involving the vasomotor center results in hypotension. Such an insult is usually lethal.
c. Fever and sepsis can be associated with hypotension.
III. PHARMACOLOGY OF CARDIOVASCULAR THERAPY
A. Guidelines for administration of vasoactive drugs
1. Use a calibrated pump for all infusions.
2. Inject into the intravenous line as close to vein insertion site as possible.
3. Avoid using systolic blood pressure (SBP) as the primary goal of therapy because this value is prone to fluctuate from a number of causes, not all of which are related to the patient’s cardiovascular condition. MAP is a more reliable variable. SBP goals may be used, however, in such settings as an unsecured cerebral aneurysm or hemorrhagic stroke where the concern is more for the sharp systolic upstroke than the mean pressure.
4. Potent vasoactive drugs should be administered in a graded fashion starting with a dose below that thought to be therapeutic. This can prevent large swings in hemodynamics that can be detrimental to surgical hemostasis as well as end-organ perfusion.
5. Consider adrenal insufficiency in patients who are hypotensive and unresponsive to adrenergic therapies.
B. Adrenergic agonists. See Table 23.1 for action of adrenergic receptors; see Tables 23.2 and 23.3 for relative adrenergic action and dosing recommendations. Sympathomimetic drugs appear to have little influence on the vascular tone of cerebral vessels. However, raising MAP above the upper threshold of autoregulation increases CBF. Some evidence suggests that beta agonists can increase the CMRO2 and secondarily raise CBF.
TABLE 23.1 Action of Adrenergic Receptors
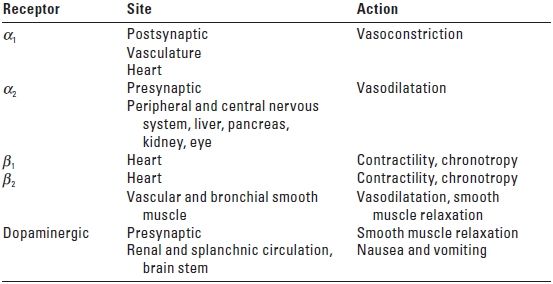
α, alpha; β, beta.
TABLE 23.2 Adrenergic Agonist and Phosphodiesterase Inhibitor Drugs
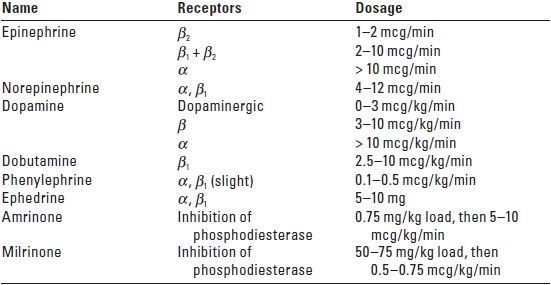
α, alpha; β, beta.
TABLE 23.3 Adrenergic Antagonist Drugs
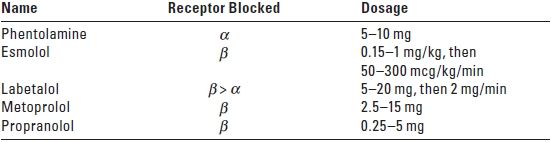
α, alpha; β, beta.
1. Norepinephrine
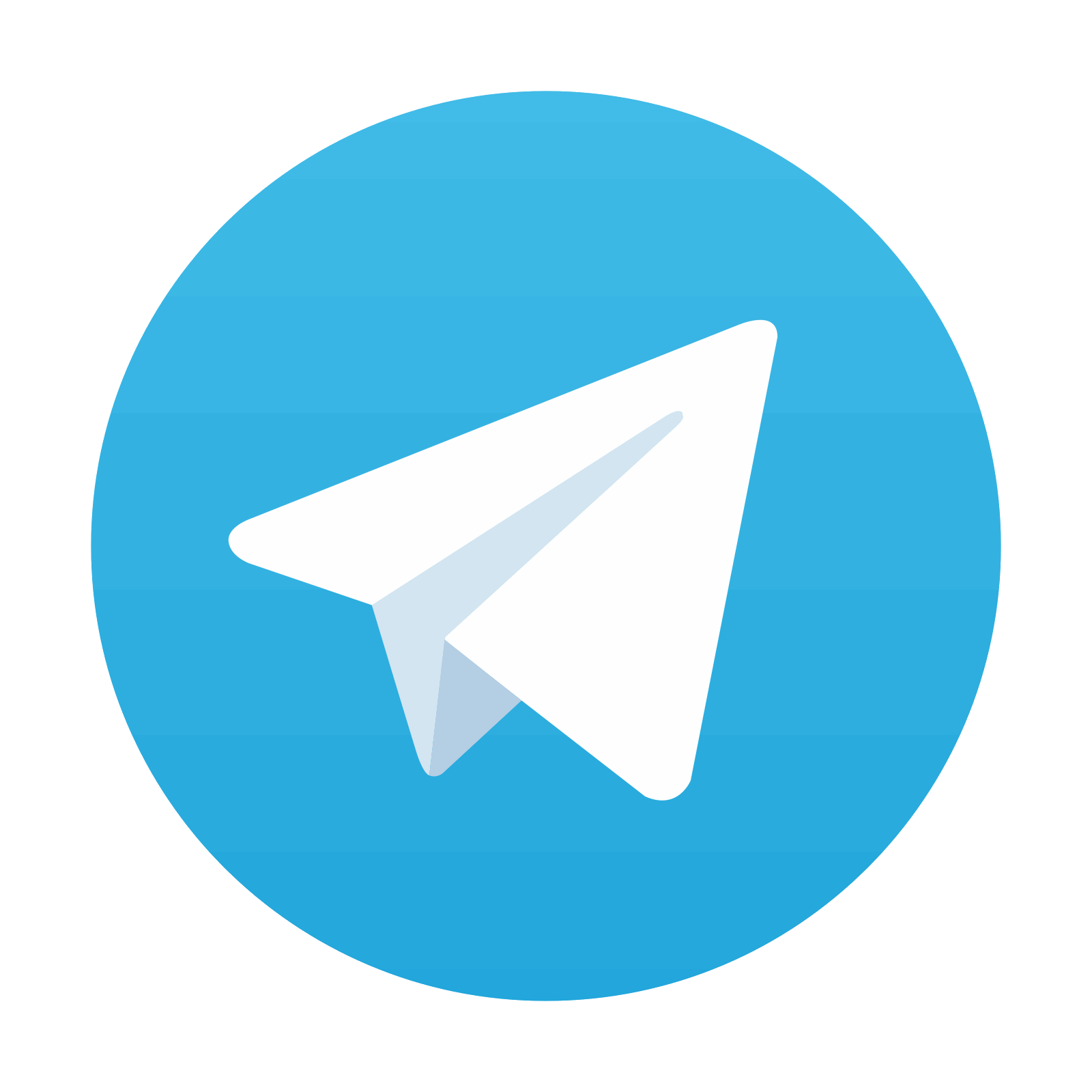
Stay updated, free articles. Join our Telegram channel

Full access? Get Clinical Tree
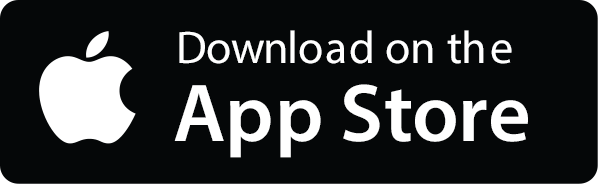
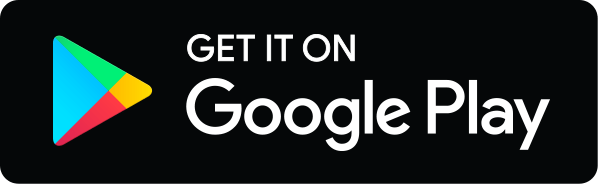