39 Cardiopulmonary Resuscitation
Epidemiology and Outcome of In-hospital Cardiopulmonary Arrest
Mechanics of Cardiopulmonary Resuscitation
Defibrillation and Cardioversion
Vascular Access and Monitoring during Cardiopulmonary Resuscitation
Medications Used during Cardiopulmonary Resuscitation
Special Cardiac Arrest Situations
Adjunctive Cardiopulmonary Resuscitation Techniques
Historical Background
In 1814, a description in poetical form of the Rules of the Humane Society for recovering drowned persons included the following description of mouth-to-mouth resuscitation1:
Let one the mouth, and either nostril close
While through the other the bellows gently blows.
Thus the pure air with steady force convey,
To put the flaccid lungs again in play.
Should bellows not be found, or found too late,
Let some kind soul with willing mouth inflate;
Then downward, though but lightly, press the chest.
External cardiac massage was successfully conducted more than 100 years ago in two children (ages 8 and 13 years) after circulatory arrest precipitated by chloroform anesthesia during a surgical procedure.2 In 1904, Crile described the effectiveness of external cardiac compressions in maintaining the circulation of dogs.3
After multiple reports that attested to the effectiveness of mouth-to-mouth resuscitation,4–6 in 1958 the National Academy of Sciences National Research Council recommended mouth-to-mouth resuscitation with maximum backward tilt of the head as the preferred technique for all individuals requiring emergency artificial ventilation. In 1960, external cardiac compression was revived as a resuscitation technique when Kouwenhoven, Jude, and Knickerbocker7 demonstrated its effectiveness when combined with artificial respirations. Many of their patients, including the first, were in cardiac arrest as a result of anesthesia. Before this study, internal cardiac compression was the accepted technique, with its effectiveness demonstrated by experience in cardiac bypass surgery. In 1947, Beck and associates8 successfully internally defibrillated the human heart; and in 1956, Zoll and colleagues9 performed the first successful external defibrillation of a human heart.
Epidemiology and Outcome of In-Hospital Cardiopulmonary Arrest
A 2009 review of cardiac arrest events submitted to the National Registry of Cardiopulmonary Circulation included 3342 pediatric events, excluding events in a delivery room or neonatal intensive care unit (NICU).10 Seventy-three percent of the inpatient cardiac arrests reported occurred in an ICU, 7% in a general inpatient area, 11% in an emergency department, and 3% in an operating room or postanesthesia care unit. Return of spontaneous circulation (ROSC) was achieved in 65%, 24-hour survival occurred in 47%, and 30% of children survived until hospital discharge. Other large series of in-hospital pediatric cardiac arrest report survival until hospital discharge ranging from 14% to 44%,11–14 with the 44% survival representing cardiac arrests that occurred in a pediatric cardiac ICU. In another multicenter cohort study of in-hospital pediatric cardiac arrest,15 48.7% of the 353 children survived until hospital discharge. Survivors had greater body temperatures, greater pH values, and reduced serum lactate concentrations compared with nonsurvivors. Nonsurvivors were more likely to have a tracheal tube before the arrest, and to receive sodium bicarbonate, calcium, and vasopressin during the arrest. In this study, postoperative cardiopulmonary resuscitation (CPR) was associated with decreased mortality.
Mechanics of Cardiopulmonary Resuscitation
Airway
In the child without an artificial airway, the use of BVM devices may result in a significant risk of gastric inflation, followed by pulmonary aspiration of gastric contents. Abdominal distention (gastric and bowel) can significantly compromise oxygenation; therefore the stomach should be vented when excessive gastric inflation occurs. One study found a 28% incidence of pulmonary aspiration in a series of failed resuscitations.16 For this reason as well as for the risk of barotrauma and volutrauma, excessive inflation pressures should be avoided. However, effective bilateral ventilation is best judged by visualizing bilateral chest excursions and listening to the quality of the breath sounds rather than setting a preset maximal inflation pressure.
Breathing
Overventilation is common during CPR, resulting in greater mean intrathoracic pressures than required, which decreases venous return and reduces cardiac output.17 In cardiopulmonary arrest, a less than normal minute ventilation may be appropriate, because cardiac output and delivery of CO2 to the lungs are diminished. If an artificial airway is not in place for single person rescue, two breaths should be given for each 30 chest compressions. If an artificial airway is not in place for two person rescue, two breaths should be given after each 15 chest compressions. Once an artificial airway is in place, a ventilator rate of 8 to 10 per minute without pausing during rapid chest compressions should be used (Table 39-1).
Circulation
During cardiac arrest, chest compressions provide the sole perfusion to a child’s vital organs; therefore optimal performance of CPR is critical. Key elements to providing quality chest compressions include (1) ensuring an adequate rate (100 compressions per minute), (2) ensuring adequate chest wall depression (one third to half of the anteroposterior chest diameter), (3) releasing completely between compressions to allow full chest wall recoil, (4) minimizing interruptions in chest compressions, and (5) ensuring that the child is on a sufficiently hard surface to allow effective chest compressions.18 In short, push hard and push fast, release completely, and do not interrupt compressions unnecessarily. Incomplete recoil during CPR is associated with higher intrathoracic pressures and significantly decreased venous return, and coronary and cerebral perfusion.19
If a child is small enough (e.g., younger than 6 months) that the person providing chest compressions can comfortably encircle the chest with his or her hands, chest compressions should be performed using the circumferential technique, with thumbs depressing the sternum and the fingers supporting the infant’s back and circumferentially squeezing the thorax (Fig. 39-1). In larger infants, the sternum can be compressed using two fingers; and in the child, either one or two hands can be used, depending on the size of the child and of the rescuer.19 Whichever method is used, focused attention must remain on delivering effective compressions with minimal interruptions.20 In all cases other than circumferential CPR, a backboard must be used. Properly delivered chest compressions are tiring to the provider, and providers should rotate approximately every 2 minutes to prevent compressor fatigue and deterioration in the quality and rate of chest compressions.19
Mechanisms of Blood Flow
External chest compressions provide cardiac output through two mechanisms: the cardiac pump mechanism and the thoracic pump mechanism. By the cardiac pump mechanism of blood flow, blood is squeezed from the heart by compression of the heart between the sternum and the vertebral column, exiting the heart only anterograde because of closure of the atrioventricular valves. Between compressions, ventricular pressure decreases below atrial pressure, allowing the atrioventricular valves to open and the ventricles to fill. This sequence of events resembles the normal cardiac cycle. Although the cardiac pump is likely not the dominant blood flow mechanism during most closed-chest CPR, specific clinical situations have been identified in which the cardiac pump mechanism is more prominent. For example, a smaller, more compliant chest may allow for more direct cardiac compression (Fig. 39-2). Increasing, the applied force during chest compressions also increases the likelihood of direct cardiac compression.
Several observations do not support the cardiac pump as the primary mechanism of blood flow during CPR. Angiographic studies show that blood passes from the vena cava through the right heart into the pulmonary artery and from the pulmonary veins through the left heart into the aorta during a single chest compression.21,22 Echocardiographic studies show that the atrioventricular valves are open during blood ejection.21,23,24 Without closure of atrioventricular valves during chest compression, the cardiac pump mechanisms cannot account for forward movement of blood during CPR.
In 1976, Criley and colleagues25 made the dramatic observation that several patients who developed ventricular fibrillation during cardiac catheterization produced enough blood flow to maintain consciousness by repetitive coughing.25 The production of blood flow by increasing thoracic pressure without direct cardiac compression describes the thoracic pump mechanism, in which the heart is a passive conduit for blood flow. The intrathoracic pressure is greater than the extrathoracic pressure during the compression phase of CPR, at which time blood flows out of the thorax, with venous valves preventing excessive retrograde blood flow (Fig. 39-3). Experimental and clinical data support both mechanisms of blood flow during CPR in human infants.
Rate and Duty Cycle
The recommended rate of chest compressions for all patients is 100 per minute, with great care taken to minimize interruptions in chest compressions and to ensure adequate compression depth.20 This rate represents a compromise that attempts to maximize contributions from both the thoracic pump and cardiac pump mechanism of blood flow.
Duty cycle is defined as the percent of the compression–relaxation cycle that is devoted to compression. If blood flow is generated by direct cardiac compression, then primarily the force of compression determines the stroke volume. Prolonging the compression (increasing the duty cycle) beyond the time necessary for full ventricular ejection should have no additional effect on stroke volume. Increasing the rate of compressions should increase cardiac output, because a fixed volume of blood is ejected with each cardiac compression. In contrast, if blood flow is produced by the thoracic pump mechanism, the volume of blood that is ejected comes from a large reservoir of blood contained within the capacitance vessels in the chest. With the thoracic pump mechanism, flow is enhanced by increasing either the force of compression or the duty cycle but is not affected by changes in compression rate over a wide range of rates, given a set duty cycle.26
Different animal models yield conflicting results as to the optimal compression rate and duty cycle. However, a rate of compression during conventional CPR of 100 per minute satisfies both those who prefer the faster rates and those who support a longer duty cycle. This is true because it is easier to produce a longer duty cycle when compressions are administered at a faster rate.27,28
Defibrillation and Cardioversion
Electric Countershock
Electric countershock, or defibrillation, is the treatment of choice for ventricular fibrillation and pulseless ventricular tachycardia. Defibrillation should not be delayed to secure an airway, because the likelihood of restoring an organized rhythm decreases with increased duration of fibrillation. Ventricular fibrillation is terminated by simultaneous depolarization and sustained contraction of a critical mass of myocardium,29 allowing return of spontaneous, coordinated cardiac contractions, assuming the myocardium is well oxygenated and the acid-base status is relatively normal. Drug treatment may be required as an adjunct to defibrillation, but by itself cannot be relied on to terminate ventricular fibrillation.
An older generation of defibrillators that is still present in many hospitals delivers energy in a monophasic damped sinusoidal waveform (Fig. 39-4, A). This type of instrument delivers a single, unidirectional current with a gradual decrease to zero current. By contrast, the newer generation of biphasic defibrillators delivers a current in a positive direction for a set period, followed by a reversal in current (see Fig. 39-4, B). Biphasic defibrillators are more effective than monophasic defibrillators in terminating ventricular fibrillation in adults; therefore their use is recommended where possible.

FIGURE 39-4 Energy delivery during conventional monophasic (A) and biphasic truncated exponential (B) defibrillation.
In the majority of adult cases, energy levels of 100 to 200 joules are successful when shocks are delivered with minimal delay.30,31 The goal of defibrillation is to deliver a minimum of electrical energy to a critical mass of ventricular muscle while avoiding excessive current that could further damage the heart. The most reliable predictor of success of defibrillation is the duration of fibrillation before the first countershock.32 Acidosis and hypoxemia also decrease the success of defibrillation.32
Practical Aspects of Defibrillation in Children
For children with in-hospital ventricular fibrillation or pulseless ventricular tachycardia, defibrillation should be attempted as soon as possible, with optimal CPR until the defibrillator is ready to deliver a shock. For the first defibrillation attempt, 2 joules/kg of delivered energy should be administered (Fig. 39-5). After shock delivery, CPR should resume immediately with chest compressions for five duty cycles (2 minutes). If one shock fails to eliminate ventricular fibrillation, the incremental benefit of another immediate shock is small. Resumption of CPR is likely to confer a greater benefit than another shock. CPR may provide coronary perfusion, increasing the likelihood of defibrillation with a subsequent shock. It is important to minimize the time between chest compressions and shock delivery and between shock delivery and resumption of postshock compressions.20 Approximately 2 minutes of CPR should be delivered before a second attempt at defibrillation at twice the original energy level (4 joules/kg).20
If ventricular fibrillation or pulseless ventricular tachycardia persists beyond the second defibrillation attempt, standard doses of epinephrine should be administered (with subsequent doses every 3 to 5 minutes during persistent cardiac arrest). After 2 minutes of chest compressions, defibrillation should be attempted again, followed by administration of amiodarone (5 mg/kg) or lidocaine (1 mg/kg) with subsequent defibrillation attempts. It is not necessary to increase the energy level on each successive shock during defibrillation after the second dose. However, successful defibrillation has been reported with currents in excess of 4 joules/kg without adverse sequelae, up to a maximum dose not exceeding 10 joules/kg or the adult level, whichever is less.20 This sometimes occurs when a fixed energy level, adult automated external defibrillator (AED) is used in a small child.
Automated External Defibrillation
Use of AEDs is now standard therapy in out-of-hospital resuscitation of adults.19,30 AEDs are now deemed appropriate for use in children older than 1 year. If available, use of pediatric attenuator pads or a pediatric mode on the AED should be used in children 1 to 8 years of age, but if unavailable (and a standard defibrillator is similarly unavailable), an unmodified AED should be used.
Vascular Access and Monitoring during Cardiopulmonary Resuscitation
Vascular Access and Fluid Administration
One of the key aspects of successful CPR is early establishment of a route for administration of fluids and medications. If intravenous access cannot be established rapidly, the intraosseous or endotracheal route should be used (see Chapter 48).
Intraosseous Access
The intraosseous route can be used to administer all medications and fluids used during CPR, including whole blood. An intraosseous needle also may be used to obtain initial blood samples, although acid-base analysis will be inaccurate after administration of sodium bicarbonate via the intraosseous needle. Intraosseous access should be considered a temporary measure during emergencies when other access is not available. The placement of an intraosseous needle in the older child (older than 10 years) and adult, although possible, is difficult owing to the thick bony cortex; however, a 50% success rate has been reported in these age-groups.33
The technique of placing an intraosseous line is straightforward. A specialized intraosseous needle or, if not available, a standard 16- or 18-gauge needle, a spinal needle with stylet, or bone marrow needle is inserted into the anterior surface of the tibia 1 to 2 cm below and 1 cm medial to the tibial tuberosity (avoiding the epiphyseal plate). The needle is directed at 90 degrees to the anteromedial surface of the tibia, just distal to the tuberosity (see Fig. 48-6 and E-Fig. 48-1). When the needle passes through the cortex into the marrow, a sudden loss of resistance is sensed. Successful placement has been achieved if the needle is in the marrow cavity, as evidenced by the needle standing upright without support. If the needle has slipped into the subcutaneous tissue, its upright position cannot be maintained without support. Free flow of the infusate without significant subcutaneous infiltration should also be demonstrated. The technique has a small complication rate,34 although possible complications include osteomyelitis, fat and bone marrow embolisms, and compartment syndrome. To avoid these potential complications, intravenous access should replace intraosseous access as soon as possible. The onset of action and concentration of most drugs after intraosseous administration are comparable with venous administration.35 A relatively new device, the EZ-IO (Vidacare, Shavano Park, Tex.) provides the most rapid means for intraosseous access (see E-Fig. 48-1).
Endotracheal Medication Administration
In the absence of other vascular access, medications including lidocaine, atropine, naloxone, and epinephrine (mnemonic LANE) can be administered via the endotracheal tube.36,
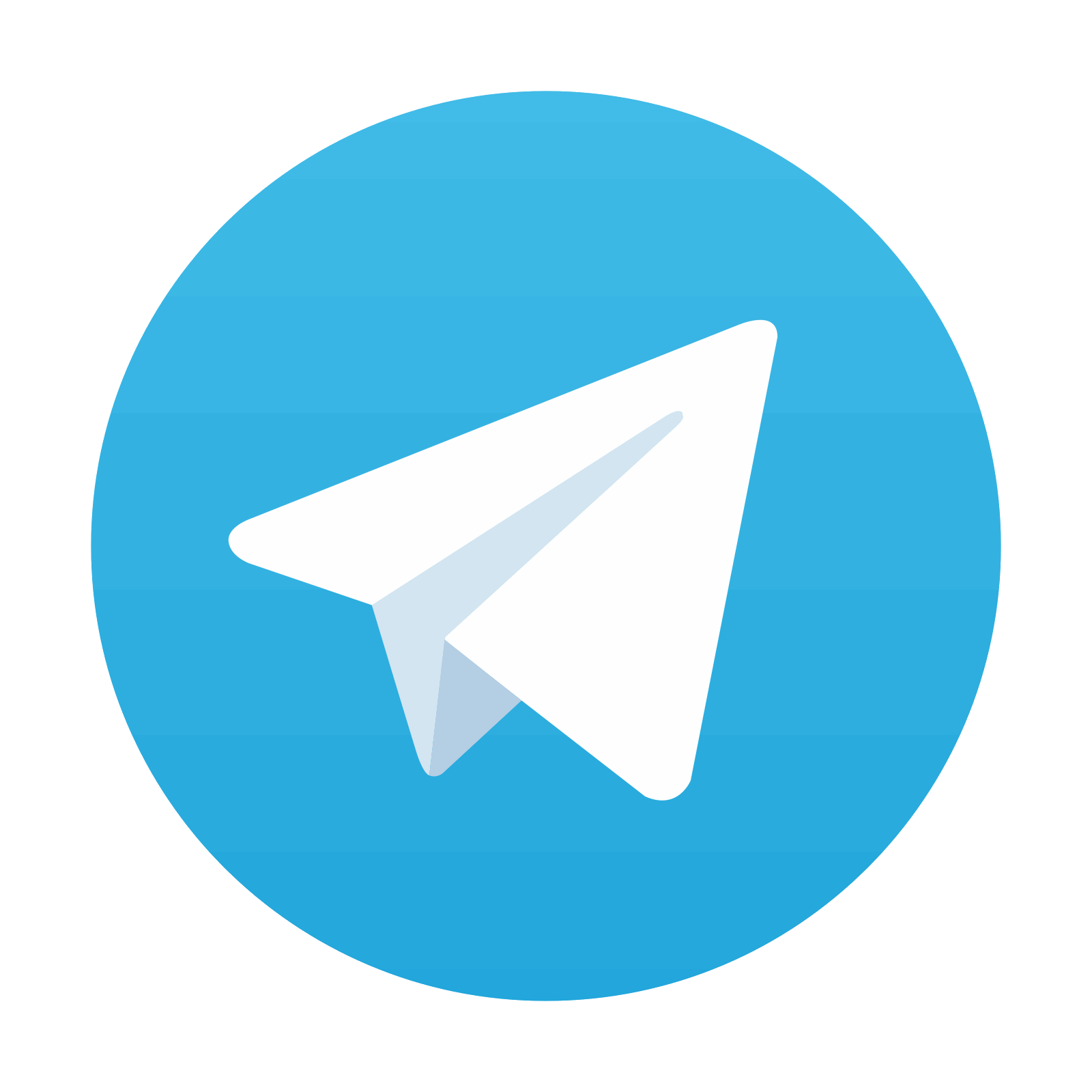
Stay updated, free articles. Join our Telegram channel

Full access? Get Clinical Tree
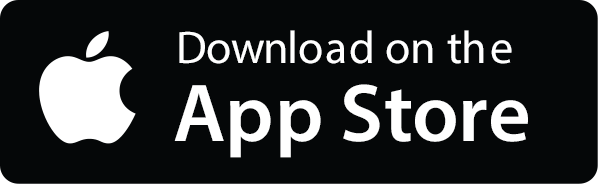
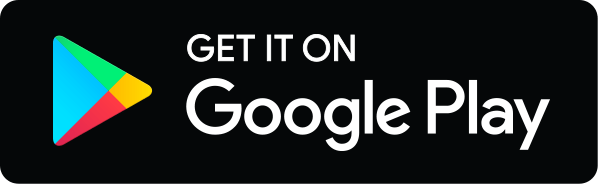