17 Cardiopulmonary Bypass and Management
Basic Aspects of Cardiopulmonary Bypass
Special Coagulation and Hematologic Problems
A Perspective on Blood Preservation: Cardiopulmonary Bypass in Jehovah’s Witness Patients
Phases of Cardiopulmonary Bypass
Particular Aspects of Management on Cardiopulmonary Bypass
Pre-Bypass Anesthetic Management
Anesthesia on Cardiopulmonary Bypass
Effects of Cardiopulmonary Bypass
Transport to the Intensive Care Unit
Basic Aspects of Cardiopulmonary Bypass
The basic principles of CPB remain unchanged from when they were first introduced in the 1950s: the CPB machine assumes the functions of the heart and lungs during the time necessary to complete either an intracardiac or an extracardiac repair. A basic bypass circuit (Fig. 17-1) consists of an oxygenator, heat exchanger, and venous reservoir; pump heads for perfusion, cardiotomy suction, and cardioplegia; and appropriate tubing, cannulas, and monitoring and alarm devices.1 Major differences exist between pediatric and adult CPB, stemming from anatomic, metabolic, and physiologic differences in these age groups (Table 17-1).
TABLE 17-1 Comparison of Pediatric Versus Adult Cardiopulmonary Bypass
Child | Adult | |
---|---|---|
Hemodilution | 3-15× adult | Moderate |
Perfusion pressure | 30-40 mm Hg | Moderate (>50-80 mm Hg) |
Wide flow rates (0-200 mL/kg/min) | Narrow range (CI 2.0-2.4 L/m2/min) | |
Blood gas management | pH-stat (Pco2 20-80 mm Hg or greater) | α-stat (Pco2 30-45 mm Hg) |
Cannulation techniques | Variable | Predictable |
Aortopulmonary collaterals | Uncommon | |
Temperature ranges | Variable | DHCA occasionally |
Glucose management | Predictable | |
Inotropic response | Negative | Positive |
Perfusion circuit | Per kilogram weight | Standard |
Parameters | Hematocrit often >55%-60% | |
Po2 40-80 mm Hg | ± | |
Sao2 75%-85% | ||
Ultrafiltration (MUF/CUF) | ±Ultrafiltration |
CI, Cardiac index; CUF, conventional ultrafiltration; DHCA, deep hypothermic circulatory arrest; MUF, modified ultrafiltration.
The Circuit and Cannulas
Bypass Circuit
Technical advances in the field of oxygenator construction and size, and reduction of priming volumes to as low as 45 mL for neonatal oxygenators, have allowed marked reductions of circuit volumes over the past decade. Also, tubing sizes can be reduced to -inch diameters, which, in combination with shorter length tubing, allows reduction of priming volumes to the range of 100 to 150 mL for neonates. A summary of the Texas Children’s Hospital sizing chart is shown in Table 17-2.
TABLE 17-2 Cardiopulmonary Bypass Circuit Prime Volume and Constituents at Texas Children’s Hospital
Patient Weight | Prime Volume | Prime Constituents |
---|---|---|
<8 kg | 350 mL | Whole blood or PRBC + FFP + crystalloid prime* |
8-15 kg | 650 mL | 100 mL albumin 25% ± PRBC + crystalloid prime* |
15-25 kg | 900 mL | 100 mL albumin 25% + crystalloid prime* |
15-25 kg | 1200 mL | Crystalloid prime* |
FFP, Fresh frozen plasma; PRBC, packaged red blood cells.
*Crystalloid prime: normal serum + Plasmalyte + CaCl2 + KCl.
Heparin Coated Versus Noncoated Circuits
Young children are more susceptible to the adverse effects of CPB than adults, and the inflammatory response to CPB may have serious consequences for neonatal and pediatric patients.2,3 This is in part related to the surface area of the CPB circuit, which is large relative to the child’s blood volume when compared with an adult’s blood volume. For example, a 3-kg neonate with a blood volume of 90 mL/kg has a total blood volume of approximately 270 mL, and with an average priming volume in many centers of 350 mL, the CPB circuit volume thus causes greater than 100% dilution. A 70-kg adult with 70 mL/kg blood volume has an approximately 5000-mL blood volume, and with a CPB circuit prime of 1500 mL, this results in less than 33% dilution. Contact of blood with the surface of the circuit also plays an important role for activation of coagulation and fibrinolysis. Heparin-coated biocompatible bypass systems reduce this activation in children weighing less than 10 kg undergoing CPB.4 They also have been shown to reduce the activation of factor XII and the complement system.5,6 This results in less production of kallikrein and bradykinin, which in turn reduces the secretion of tissue plasminogen activator from endothelial cells. One study has documented more bleeding with the use of a conventional, non–heparin-coated circuit compared with a heparin-coated circuit.6 Overall, children operated on while supported with heparin-coated circuits have a significant reduction in inflammatory mediator release and fewer consequences thereof, such as prolonged postoperative ventilation and stay in the intensive care unit (ICU).7
Cardiopulmonary Bypass Pumps
The two pumps used most commonly for CPB are roller pumps and centrifugal pumps. Roller pumps have the advantages of simplicity, low cost, ease and reliability of flow calculation, and the ability to pump against high resistance without reducing flow.8 Disadvantages include the need to assess occlusiveness, spallation of the inner tubing surface (potentially producing particulate arterial emboli), capability for pumping large volumes of air, and ability to create large positive and negative pressures. Compared with roller pumps, centrifugal pumps offer the advantages of less air pumping capabilities, less ability to create large positive and negative pressures, less blood trauma, and virtually no spallation. Disadvantages of centrifugal pumps include higher cost, the lack of occlusiveness (creating the possibility of accidental patient exsanguination), and afterload-dependent flow that requires constant flow measurement. In the setting of short-term CPB for cardiac surgery, it remains uncertain whether the selection of a roller pump over a centrifugal pump, or of any specific centrifugal pump over another, has clinical importance. Pulsatile perfusion may prove to be beneficial in the future, but further outcome data and technical improvements are needed.9
Cardiopulmonary Bypass Prime
The optimal priming fluid in cardiac surgery is a topic of enduring debate. Crystalloid solutions, colloids, and mixtures of both are used. Children appear to benefit from a colloid prime. If crystalloid is used for priming, it should not contain lactate or dextrose because CPB induces a metabolic acidosis10 that has been shown to be iatrogenic and not splanchnic in origin.11 The addition of lactate to the prime increases postoperative serum lactate concentrations and should be avoided.12 Hyperchloremic metabolic acidosis is the second contributing component of a metabolic acidosis on CPB. This is often only detected by measuring the strong ion difference via the Stewart approach to the acid-base homeostasis.13 Both acidifying events are attenuated by the dilutional hypoalbuminemia induced by the administration of the pump prime. Because a hyperchloremic acidosis of a mild degree seems to be well tolerated and not associated with a poor outcome, no intervention seems necessary. Understanding the nature of CPB-associated acidosis, however, is likely to prevent unnecessary investigations or interventions.
The proportionally large volume of the bypass circuit compared with the child’s blood volume has a significant impact on the coagulation factors and cellular components. Platelet count decreases and coagulation factors, including fibrinogen, are diluted after bypass; these factors may contribute to a coagulopathy. The fibrinogen concentration at the end of bypass has been shown to correlate with the 24-hour chest drainage in children weighing less than 8 kg.14 This is seen more frequently in infants and neonates in whom an average decrease in plasma concentrations of hemostatic proteins by 56% immediately on initiation of bypass can be observed.15 Overall, younger age represents the single most important risk factor for coagulopathy and bleeding complications.16
One approach to the just-mentioned problems is the addition of whole blood to the circuit prime. Proponents cite two advantages: (1) improved hemostasis and (2) a decreased SIRS with less edema formation and less organ dysfunction. One study has disproved these perceived advantages; the investigators found that the use of fresh whole blood increased perioperative fluid requirements, leading to a longer duration of mechanical ventilation and ICU stay than in the single component group.17 The only advantage found by their study was the lesser number of donor exposures, a problem we try to overcome by matching packed red blood cells (PRBCs) and fresh frozen plasma (FFP) from the same donor.18 In addition, whole blood is frequently not available. An alternative approach is the use of FFP in the prime.19 Other investigators found that the use of FFP led to greater fibrinogen concentrations at the end of surgery. On average, children in the FFP group needed 1.3 fewer donor exposures and tended to need fewer PRBCs. The lower donor exposure was primarily because of fewer transfusions of cryoprecipitate.19 FFP may be safely substituted by 5% albumin in the prime in children with less complex repairs and acyanotic lesions.20 Whenever possible, we prefer fresh blood less than 5 days old. Fresh PRBCs are presumably more balanced metabolically than stored PRBCs; the former contain less potassium, a greater concentrations of glucose, reduced concentrations of lactate, and a greater pH.21 Also postoperative morbidity increases with increasing age of red blood cells.22 Pulmonary complications, acute renal failure, and increased infection rates were among the main complications associated with increased red blood cell storage time. As far as potassium levels and acid-base balance are concerned, PRBC priming can be safely performed with stored PRBCs if the priming solution is circulated for 20 minutes before the initiation of CPB.23
The average prime volume of the circuits we use is shown in Table 17-2. Other prime additives are heparin, antifibrinolytics, antiinflammatory agents (corticosteroids), antibiotics, vasodilators, and, sometimes, diuretics (mannitol, furosemide). At the end of the case and before separation from bypass, blood gas analysis is done to ensure that the electrolytes (including calcium and magnesium ions), glucose, and hematocrit are within a desired range. Acid-base changes and sodium concentration are corrected with sodium bicarbonate, and residual lactate is washed out with the help of the hemofiltration.
Antifibrinolytic Agents (See Chapter 18)
Aprotinin
The first use of aprotinin in pediatric cardiac surgery was reported in 199024; a high-dose regimen was administered to 28 children at increased risk of bleeding. This population included those undergoing transposition of the great arteries or reoperations, and children with endocarditis. No reduction in blood loss or drainage was observed; there were no adverse effects, and chest closure time was reduced.
Despite the high cost of aprotinin, follow-up studies have had more favorable results and its use has been shown to reduce overall costs, with decreased patient charges as a result of reduction in the number of blood products used, operative time, duration of postoperative ventilation, and hospitalization.25,26 This was confirmed in a recent comparative analysis amongst antifibrinolytic medications.27 However, this benefit was observed only in complex repairs and the use of a high-dose regimen.28 The lesser effect of a low-dose regimen may be attributable to the dilutional effects in pediatric surgery compared with the adult population.29 Pediatric lung transplantation has been studied as a potential target group for aprotinin use.30 As in most high-risk groups, a significant benefit was found for children with repeat operations (defined as repeat sternotomies or repeat transplantations), either with a high- or a low-dose regimen. This is consistent with our experience. Also, in general, infants younger than 6 months of age and those with repeat sternotomies seem to get a particular benefit from a high-dose regimen of aprotinin31 compared with reduced doses, despite greater drug costs. Economic studies have shown a cost-effective benefit of aprotinin in repeat cardiac procedures.25,26
Aprotinin seems to have an influence on the inflammatory response to CPB in children.32 Less time mechanically ventilated postoperatively33 and an improved Pao2/Fio2 (ratio of arterial oxygen concentration to the fraction of inspired oxygen, or P/F ratio) as an indicator of an attenuated reperfusion injury of the lung with the use of aprotinin have been reported.34 The clinical relevance of its antiinflammatory action remains unclear but points toward significant antiinflammatory properties.
Although a standard dosing regimen has yet to be defined in children, pediatric studies have demonstrated decreases in operative time post CPB, exposure to donor blood, and postoperative chest tube drainage with the use of aprotinin.33 In vitro plasma concentrations of aprotinin have been related to antifibrinolytic and antiinflammatory activity at concentrations of 50 to 125 kallikrein inhibitor units (KIU)/mL and 200 KIU/mL, respectively.25,35,36 Anaphylactic and anaphylactoid reactions may occur with aprotinin, and a test dose should be given before administration of the loading dose or addition of aprotinin to the CPB circuit. In a retrospective review of 681 children, reactions occurred in 1% of first exposures, 1.3% of second exposures, and 2.9% of more frequent exposures.37
We used aprotinin for complex neonatal repairs, such as arterial switch operations or Norwood procedures, as well as for most reoperative procedures and organ transplantations.38,39 Because of safety concerns in adults, the drug is currently unavailable in the USA and Europe. The decision to withdraw the drug was based on data that were obtained entirely from adults, who present with a different profile of complications following cardiac surgery than do children. Aprotinin has been shown to be safe and efficient in the neonate.40 Furthermore, serious questions have been raised regarding the statistical method used in the sentinel study that questioned the safety of aprotinin. Aprotinin continues to be used in Australia and New Zealand and has been reintroduced for adult coronary artery bypass graft surgery in Canada. Currently, the FDA in the United States is reevaluating the role of aprotinin in anesthesia. Our dosing regimen is weight based, with 60,000 KIU/kg as a loading dose and in the pump prime. Aprotinin is started before the incision and blood levels are maintained with a continuous infusion of 7000 KIU/kg/hour; this is discontinued just before leaving the operating room. Regimens based on body surface area are also used, along with a CPB prime dose based on priming volume designed to achieve a plasma level above 200 KIU/mL. An example is 0.85 to 1.7 × 106 KIU/m2 loading dose, and into the CPB prime, and 2.0 to 4.0 × 105 KIU/m2/hour infusion.25
The Lysine Analogues: Aminocaproic Acid and Tranexamic Acid
Despite meticulous surgical technique, it is still frequently difficult to achieve adequate hemostasis after CPB, particularly in neonates. ε-Aminocaproic acid (EACA) and tranexamic acid (TXA) are analogs of the amino acid lysine. They exert their antifibrinolytic effect by interfering with the binding of plasminogen to fibrin, thereby preventing the activation of the active plasmin. TXA may also improve hemostasis by preventing plasmin-induced platelet activation. Both TXA and EACA exercise some antiinflammatory properties but not to the same extent as aprotinin. In one study, the use of EACA reduced bleeding postoperatively in 25 of 71 children undergoing cardiac surgery on CPB and was found to benefit only the children with cyanotic heart disease.41 The EACA loading dose was 75 mg/kg followed by an infusion of 15 mg/kg/hour; an additional 75 mg/kg was added to the CPB prime. Because the effective dose of EACA is unknown, another study used a regimen of 150 mg/kg bolus followed by an infusion of 30 mg/kg/hour of EACA. Intraoperative blood loss was reduced, but postoperative blood loss was not different between treatment arm and placebo.42 Blood coagulation measured with a thromboelastograph showed less fibrinolysis with EACA.
EACA compares favorably with TXA; a beneficial effect has been reported only in children with cyanotic heart disease.43 Those with acyanotic defects or undergoing repeat sternotomies had no benefit from TXA. Their dosing regimen, however, was only 50 mg/kg as a single bolus before incision. In children, the TXA plasma concentration between the post bolus peak and the end of CPB has an 80% decline when a continuous infusion is not used.44
In conclusion, though less efficient than aprotinin, TXA and EACA are equally effective in reducing perioperative blood loss in pediatric cardiac surgery.45 Given their safety profile, they may be even more appealing in the future. Further studies are needed to delineate their pharmacokinetic profiles and their efficacy. We use EACA based on simulation results from a study by Ririe and coworkers.46 An initial loading dose of 75 mg/kg over 10 minutes and a maintenance infusion rate of 75 mg/kg/hour is used with 75 mg/kg placed in the pump to maintain serum concentrations above the therapeutic concentration (assumed to be 130 µg/mL) in more than 95% of children.
Special Coagulation and Hematologic Problems
Heparin-Induced Thrombocytopenia
The use of unfractionated heparin for anticoagulation for CPB in adults produces antiheparin antibodies in 25% to 50% of patients within 10 days postoperatively. In a small minority of these patients, high-titer IgG platelet-activating antibodies form and make immune complexes with heparin and platelet factor 4 (PF4).47 This results in activation of platelets (via their Fc receptors) and formation of procoagulant platelet microparticles, leading to thrombin generation and thrombosis. Thus, the major problem in heparin-induced thrombocytopenia (HIT) is thrombocytopenia several days after heparin exposure accompanied by thrombosis, often in major vessels or structures. HIT appears to be less common, of milder course, and probably underrecognized in neonates and children. About 1% of children exposed to CPB have PF4 antibodies when tested before their second CPB, and actual HIT is much less common.48 When HIT is suspected, either PF4 enzyme-linked immunosorbent assay or a functional assay for HIT can be used to make the diagnosis; if positive, no further heparin should be given. If CPB is necessary, alternatives, such as the direct thrombin inhibitors argatroban, lepirudin, and bivalirudin, may be used. None of these agents is approved for use in children for anticoagulation for CPB, but case reports and small series have documented their successful use when HIT is diagnosed.49–51 The partial thromboplastin time (PTT), activated clotting time (ACT), and a specialized clotting time called the ecarin clotting time can be used to follow anticoagulation with these agents, but there is no reversal agent for them. Thus, treatment of post-CPB bleeding involves administration of blood products and coagulation factors as well as recombinant factor VIIa (see later discussion).
Antithrombin III Deficiency
Heparin produces anticoagulation by combining in a 1 : 1 ratio with antithrombin III (ATIII), which then binds to and inhibits thrombin, leading to anticoagulation. Of adult patients, 4% to 13% have a resistance to normal doses of heparin for CPB; most instances occur because of a partial deficiency of ATIII, rendering heparin less effective at producing anticoagulation.52 In children this is often unknown, and the first suspicion of ATIII deficiency may occur when the standard heparin dose of 300 to 400 units/kg fails to adequately anticoagulate before CPB, that is, the ACT remains less than 300 seconds. The usual response is to apply another dose of heparin from a different vial and remeasure the ACT, but if the ACT is still not adequately prolonged, a diagnosis of ATIII deficiency may be suspected. Infants less than 6 months of age and children with congenital heart disease have decreased ATIII concentrations.53 Therefore, heparin may not achieve adequate anticoagulation, and disorders in hemostasis and thrombosis and an exaggerated inflammatory response may occur. In this case, blood can be sent for ATIII levels, but to proceed with CPB, the ATIII must be increased. This can be accomplished in two ways: (1) by supplementing ATIII with recombinant ATIII, 75 units/kg, and ensuring that the ACT is adequately prolonged before proceeding with CPB, or (2) by adding FFP (which has ample levels of ATIII) to the CPB prime or administering it to the child.52,54
Recombinant Factor VIIa for Massive Hemorrhage
Recombinant factor VIIa (rFVIIa) was originally approved for use in patients with hemophilia who possess inhibitors to factors VIII or IX, and was shown to be effective at treating bleeding in these patients at doses of 90 µg/kg (see Chapter 10).55 Endogenous factor VII circulates at low concentrations in the plasma. At a site of tissue or blood vessel injury, tissue factor (TF) is exposed, and the extrinsic coagulation pathway is activated by the binding of factor VII to TF, resulting in the activation of factor X to factor Xa, leading to the generation of thrombin from prothrombin, with further activation of platelets and the coagulation cascade.56 High concentrations of rFVIIa result in major activation of the extrinsic pathway at the site of injury, theoretically without resulting in systemic hypercoagulability. However, thrombotic complications are increased after its use. rFVIIa also activates platelets, adding to the potential benefit of this agent in significant hemorrhage. Thus, this therapy seems attractive for the treatment of surgical bleeding. A recent analysis of factor VII concentrations during pediatric cardiac surgery recommended that it be used to treat postoperative coagulopathies that were resistant to conventional therapy, when no identifiable surgical cause of bleeding could be determined.57
Sickle Cell Disease
Sickle cell disease (SCD), one of the most common hemoglobinopathies among patients of African-American or West Indian origin (with a prevalence of 0.2% to 0.3% in that population), results from the substitution of valine for glutamic acid in position 6 of the β-hemoglobin chain. Normal adult hemoglobin is referred to as HbA, whereas hemoglobin containing the mutant β-hemoglobin chains is referred to as HbS. SCD is represented by a homozygous genotype (HbSS) with fractional concentrations of HbS in the range from 70% to 90%. Sickle cell trait, on the other hand, is a heterozygous manifestation (HbAS) with a prevalence of 8% to 10% in the same population. The definitive diagnosis of any sickle cell hemoglobinopathy is confirmed by hemoglobin electrophoresis (see Chapter 9).
Children with SCD are at a particular risk for perioperative complications.58,59 Sickling can be triggered by hypoxia, dehydration, acidosis,60 hypothermia, stress, and infections. Hypoxia induces opening of a Ca2+-activated K+ channel (Gardos channel) that causes intracellular dehydration.61 Chain formation occurs and leads to increased blood viscosity with vaso-occlusion. Opening of the Gardos channel is an important mechanism of sickle cell dehydration, which is temperature dependent, with greater potassium efflux at lower temperatures.62 Shrinkage of sickle erythrocytes may also result from activation of a K+/Cl− cotransport pathway under acidotic conditions.63 Activation of this pathway can be blocked by increasing the abnormally low level of intracellular magnesium in sickle erythrocytes. The use of magnesium and hydroxyurea in the perioperative period therefore seems to be beneficial.64
CPB, particularly for more complex surgical procedures, may involve periods of low flow or even circulatory arrest, as well as hypothermia with consequent local vasoconstriction, hypoxemia, and acidosis. There is some evidence that CPB can be safely undertaken in SCD.65 Flow conditions are an important determinant of sickle erythrocyte adherence to endothelium. Under low-flow conditions sickle cell adhesion to endothelium increases with contact time in the absence of endothelium activation or adhesive proteins, whereas under venular flow conditions sickle cell adhesion occurs only after endothelial activation. During CPB, both low-flow conditions and endothelial activation may occur. Multiple triggers of sickling are likely to occur during CPB, and close attention should be paid to the conduct of all aspects of bypass.
In the past, routine exchange transfusion has been recommended to prevent these complications.66 More recent experience provides evidence that not all children require an exchange transfusion.67 The growing evidence of the harmful effects of blood transfusion adds to the need to carefully reconsider routine exchange transfusion.68 For uncomplicated bypass surgery without periods of cardiac arrest, the omission of exchange transfusion has led to good outcomes.
Guidelines have been proposed for the perioperative management of children with sickle cell disorders.67 It is essential to avoid hypothermia using tepid or warm CPB in its stead; blood transfusion only for a decrease in hematocrit to less than 20%; maintenance of intravascular volume and body temperature while on CPB; the avoidance of vasopressors; the use of postoperative multimodal pain therapy; and early incentive spirometry to prevent pulmonary complications.69 In our practice, we utilize cerebral near-infrared spectroscopy (NIRS) to help determine an acceptable hematocrit for the individual child.
For children undergoing hypothermia, successful management with70 and without71 partial or complete exchange transfusion on bypass has been reported. Exchange transfusion can be performed preoperatively or on initiation of CPB.72 For exchange transfusion during CPB, the extracorporeal circuit is primed with blood and the usual components. When CPB is commenced, the child’s blood volume is drained into storage bags and separated. The platelet-rich plasma is reinfused at the end of CPB, and the concentrated sickle cells are discarded. Platelet and plasma sequestration in conjunction with exchange transfusion reduces the need for postoperative transfusion and protects the platelets from the negative effects of CPB.73
There seems to be no consensus as to a suitable target HbS level. Reducing the absolute level of HbS may be of greater benefit than achieving a particular ratio of HbA to HbS because the remaining sickle-prone cells are still at risk for sickling.74 In SCD, exchange transfusion has been shown to favorably affect cerebral tissue oxygenation.75 Exchange transfusion will decrease both the proportion and absolute amount of HbS, but it does not remove every cell that may sickle; it may also have favorable effects on hypoxic pulmonary vasoconstriction.75 In this context, these children may benefit from continuous hemofiltration to reduce inflammatory mediators and improve pulmonary recovery.76 Inhaled nitric oxide also has been suggested as an adjunct for the prevention of sickle cell crisis. It may improve the binding of oxygen, thereby reducing the formation of sickle cells; reduce pulmonary hypertension; and improve pulmonary function without adverse effects on normal hemoglobin.77
A Perspective on Blood Preservation: Cardiopulmonary Bypass in Jehovah’s Witness Patients
Acute isovolumic reduction of hemoglobin down to 5 g/dL is tolerated in healthy individuals under anesthesia and does not appear to reduce tissue oxygenation significantly.78 Reduction of oxygen delivery to 7 to 8 mL/kg/min under resting conditions does not lead to an oxygen debt and is compensated by increased extraction, an increase in cardiac index, and a subsequent decrease in systemic vascular resistance.79,80 In a retrospective study of the tolerance of reduced hemoglobin concentration in Jehovah’s Witness patients, the hemoglobin concentration of those who died was less than 5 g/dL.81 A safe limit of hemodilution in children has not been established. One report showed that hemodilution up to 50% in acyanotic children appears to be safe.82 In cyanotic children, however, the limit was estimated to be around 40%. If this level of hemodilution is exceeded, hemodynamic instability and inadequate oxygen transfer can occur. Evidence suggests that hematocrit levels of 21.5% in infants on CPB leads to significantly worse psychomotor developmental outcome, compared with 27.8%.83
The most important and simplest way to avoid transfusion in the setting of cardiac surgery is to limit blood loss. Unnecessary and reduced amounts of blood sampling help to preserve blood.84 Pharmacologic agents, such as aprotinin and tranexamic acid, reduce the risk of perioperative blood loss.85 Hormonal stimulation of erythropoiesis with preoperative recombinant erythropoietin is another strategy acceptable to Jehovah’s Witnesses. The administration of erythropoietin in the cardiac surgery setting has been shown to reduce the risk of exposure to allergenic blood.86 However, the associated increase in hematocrit with erythropoietin use may be potentially thrombogenic and could lead to an increase in the incidence of perioperative venous thromboembolism. The cost of using erythropoietin can be large, and cost analysis suggested that its use in cardiac surgery was not cost effective.87
Intraoperative recovery of blood with a cell salvage device is also acceptable to many Jehovah’s Witnesses. This involves the removal by suction of blood from the operative field followed by washing, filtering, and return of red blood cells to the patient. A randomized controlled trial of intraoperative cell salvage in cardiothoracic surgery has demonstrated a reduction in red blood cell transfusion and an increase in postoperative hemoglobin.88
Acute normovolemic hemodilution involves the preoperative removal of a volume of blood from the patient with the simultaneous administration of crystalloid or colloid to maintain circulating volume.89 The collected blood is then reinfused during the operation. Some Jehovah’s Witnesses find this process acceptable, especially if the access line is maintained in continuity with the patient. Acute normovolemic hemodilution has other advantages, including lower costs, because the blood does not need compatibility testing; reduced possibility of administrative error; and a saving in patient time (see Chapter 10). The development of artificial red cell substitutes could potentially abrogate the need for compatibility testing, as well as vastly reduce infection risks, with none of the immunomodulatory side effects of allogeneic blood.90 Some of these products would also be acceptable to Jehovah’s Witness families. Substitutes include perfluorocarbons, hemoglobin solutions, intramolecular cross-linked hemoglobin, and liposome encapsulated hemoglobin. None of these has reached clinical practice. Lastly, autologous retrograde priming has been used in Jehovah’s Witness patients and can further reduce the hemodilutional effects of the prime.89,91
In the great majority of adult patients, open-heart surgery can be performed without administration of blood or blood components. In children and small infants weighing less than 5 kg, bloodless open-heart surgery is more complicated. Preoperative iron supplementation (6 mg/kg/day) and erythropoietin (200 to 400 U/kg/wk) have been used successfully to augment preoperative hemoglobin levels.92
Postoperative care involves minimal blood sampling, and only on special indications. Noninvasive monitoring allows uncomplicated weaning from the ventilator.93 The first report of successful outcomes in Jehovah’s Witness children with congenital cardiac defects was in 198594; 110 children older than 6 months of age successfully underwent operation, with a perioperative mortality rate of 5.3%. Only one death was attributed to blood loss. A weight less than 5 kg is considered by some as a contraindication for open-heart surgery and palliative procedures were advocated in the past.95 For some lesions, however, no palliation is possible. The development of miniaturized circuits, preoperative optimization, use of high-dose aprotinin, vacuum-assisted drainage to allow smaller tubing and cannula sizes, as well as the use of modified ultrafiltration, enabled the safe expansion of surgery into the neonatal population. Individualized heparin level–based anticoagulation management further results in a reduction of coagulation problems, blood loss, and transfusion requirements.96 The addition of desmopressin, 0.3 µg/kg, also not proven, is thought by some to improve platelet activity and stimulate the release of von Willebrand factor after protamine infusion.
Myocardial Protection
Myocardial protection during cardiac surgery has evolved over the years. Melrose and colleagues introduced the concept of chemical cardioplegia in 1955.97 Before the popular use of chemical cardioplegia, topical cardiac hypothermia was used. In the late 1970s and early 1980s, the concept of cold hyperkalemic blood cardioplegia was introduced.98 Potassium concentrations in cardioplegic solutions ranging from 12 to 30 mEq/L are typically used to achieve cardiac standstill within 1 to 2 minutes under hypothermic conditions, with higher concentrations (or longer induction times) required for normothermic conditions. Myocardial edema after bypass and global ischemia can be reduced by a number of strategies that involve modifying the conditions of delivery and composition of cardioplegia solutions as they affect the movement of intracellular and interstitial fluid. In contrast to studies in adults, most studies conducted in neonates have shown little difference between blood and crystalloid cardioplegia.99,100 Hypothermia also decreases myocardial oxygen consumption. The benefits of this approach appear to be optimal at myocardial temperatures between 24° C and 28° C. However, there is growing evidence that warm, intermittent blood cardioplegia may be advantageous to either cold crystalloid or cold blood cardioplegia.101 The benefits of blood cardioplegia are more pronounced in younger, cyanotic children who require longer aortic cross-clamping. For acyanotic children, the cardioplegic technique is probably not as critical.102 Avoidance or reduction of myocardial edema occurs by limiting the pressure of cardioplegia infusions and by providing moderately hyperosmolar cardioplegia solutions that contain blood. Buffering the acidosis that results from ischemia is achieved by including tromethamine, histidine-imidazole, or both in the cardioplegia solution. Close management of myocardial calcium balance to avoid extremes of intracellular hypercalcemia or hypocalcemia, especially during reperfusion, is very important.103,104 The addition of magnesium may solve this dilemma by preventing damage from higher cardioplegic calcium concentrations by its action as a calcium antagonist.104,105 This prevents mitochondrial calcium overload as a consequence of reperfusion injury. Magnesium also prevents the influx of sodium into the postischemic myocardium, which is exchanged for calcium during reperfusion.
Every cardiac program has its own philosophy regarding cardioplegia and myocardial protection. At Texas Children’s Hospital, plain crystalloid cardioplegia is used. The prime blood gas and electrolytes should mimic physiologically the child’s arterial blood gas as closely as possible. If whole blood or packed cells are added to the prime, the target hemodilution range should be 28% to 30%; the prime should be recirculated continuously and warmed between 35.0° C and 36.5° C before initiation of bypass. In neonates and infants, albumin is added to the cardioplegic solution to maintain an appropriate colloid osmotic pressure. This may decrease edema formation of the arrested heart. In children undergoing circulatory arrest, long cross clamp times, and large pump suction return cases, 20 mg/kg methylprednisolone is used, up to a maximum of 500 milligrams, to reduce the production of inflammatory mediators that result in myocardial dysfunction. Table 17-3 summarizes the Texas Children’s Hospital protocols for cardioplegia and myocardial protection.
Phases of Cardiopulmonary Bypass
Surgical cases requiring CPB are divided into several basic phases.
Cannulation and Initiation of Bypass
After sternotomy and mediastinal dissection, the aorta is cannulated, along with either the right atrium, if single venous drainage is planned, or the superior and inferior venae cavae for bicaval venous drainage. A large dose of heparin (300 to 400 units/kg) is administered intravenously, and the adequacy of anticoagulation is measured using the ACT before initiating CPB. The target ACT is usually 480 seconds. High ACTs are maintained during CPB with the addition of heparin to the prime as needed, because larger doses of heparin lead to a reduced degree of consumptive coagulopathy, which translates into reduced blood product therapy requirements.96 Other methods of measuring anticoagulation include the Hepcon system (a plasma heparin concentration assay), which may allow for more accurate titration of heparin and protamine dosages.106 The thromboelastogram may also be used as a baseline measure of the coagulation system and then may be repeated during bypass, with heparinase added to more objectively assess each child’s anticipated need for coagulation products.107 An improved preservation of the hemostatic system with subsequent reduction of blood loss and a reduction in transfusion requirements has been demonstrated after maintenance of high heparin levels during CPB.108 The additional maintenance of high ATIII concentrations may further contribute to a reduction of hemostatic activation.109
In most centers, bicaval cannulation is used for all but the smallest children (less than 2 kg) to prevent venous return from interfering with the surgical field. A gradual transition to full CPB is then performed to minimize myocardial stress, using a prime that has essentially the same composition as the child’s blood with regard to temperature, pH, calcium, potassium, and hematocrit. CPB flows of 150 mL/kg/min are used for infants weighing less than 10 kg, and 2.4 L/min/m2 is used for children weighing more than 10 kg. Flow rates may be reduced during periods of hypothermia (see later), although many centers now prefer to maintain greater flows throughout the bypass period. Misplaced cannulas can lead to significant morbidity. Obstruction of the inferior vena cava (IVC) by a misplaced IVC cannula can lead to increased venous pressure, which causes ascites and decreased perfusion pressure in mesenteric, hepatic, and renal vascular beds. Misplacement of the cannula in the superior vena cava can result in cerebral edema from inadequate venous drainage and a subsequent reduction in cerebral blood flow, potentially resulting in ischemia. Arterial cannula misplacement can also occur. If the cannula inadvertently slips beyond the takeoff of the right innominate artery, preferential perfusion to the left side of the brain can be observed. This can be detected on the NIRS monitor, which may be an important monitor, particularly in pediatric cardiac surgery.110
Removal of Aortic Cross Clamp and Rewarming Phase
After completion of the intracardiac repair and de-airing of the heart, the aortic cross clamp is removed, allowing reperfusion of the myocardium. Optimally, normal sinus rhythm and myocardial contractility are restored during this time, while the child is slowly rewarmed. During rewarming, surgery is completed, inotropic and vasoactive agents are started, and ventilation begins. Hemofiltration and blood transfusion are used to achieve the desired hematocrit. Left atrial and/or pulmonary artery monitoring lines, if indicated, are placed at this time, as are temporary atrial and ventricular pacing wires. If the child is incompletely rewarmed before separation from CPB, a significant afterdrop with precipitous post-bypass reduction in core body temperature can occur. This can lead to vasoconstriction, shivering, increased oxygen consumption, and acidosis. However, postischemic hyperthermia can lead to delayed neuronal cell death.111 Mild degrees of hypothermia and certainly the avoidance of hyperthermia are essential in the perioperative period.112 In children, rectal temperature mostly reflects peripheral temperature. One study showed that the temperature of the foot was more sensitive than the temperature of the hand.113 Another study revealed that for anatomic or physiologic reasons, temperature gradients in the toes develop more readily than those in the fingers.114 Several endpoints have been proposed, such as nasopharyngeal temperatures greater than 35.0° C, bladder temperature greater than 36.2° C, or skin temperatures greater than 30° C115,116; we use an endpoint of 35.5° C rectal temperature.117
Post-Bypass Period
This phase lasts until chest closure and transfer to the ICU have been accomplished. During this time, modified ultrafiltration (MUF) may be performed for 10 to 15 minutes after cessation of CPB. Cardiac function and the quality of the surgical repair are assessed via TEE, and, if found to be satisfactory, protamine is administered to neutralize residual heparin. The usual dose of protamine is 1.0 to 1.3 mg/100 units of heparin given at the onset of bypass. Limiting protamine to this dose prevents an overdose with its associated effects on platelet function (reduction of the interaction of glycoprotein Ib receptor interaction with von Willebrand factor).118 If the ACT remains increased or prime blood is given back to the child, an additional 25% of the initial dose of protamine is added and the ACT is rechecked. However, particularly in infants, the administration of protamine and the persistent treatment of a suspected incomplete heparin reversal should not distract and delay the treatment of other commonly associated post-bypass coagulopathies, such as thrombocytopenia, platelet dysfunction, and other coagulation factor deficiencies.
Protamine reactions occur much less frequently in children younger than 16 years of age, approximately 1.76% to 2.88%.119 Independent risk factors are a female gender, a larger protamine dose, and smaller heparin doses. Type I reactions or effects during administration are rare and adding calcium does not change the hemodynamic consequences of injection.120 Fortunately, severe anaphylactic reactions (type II) or catastrophic pulmonary vasoconstriction (type III) are rare but have been observed by us and others.121 Administering the protamine over no less than 5 minutes reduces the severity and precipitous nature of any protamine reaction.
Because CPB can have a multitude of adverse physiologic effects, attempts are made to minimize both the duration of CPB and ischemic (aortic cross clamp) time; thus, as much of the surgery as possible is performed outside of these phases. In general, physiologic responses to bypass are more extreme with decreasing age and size of the child. The neonate experiences a greater degree of hemodilution on bypass and colder temperatures on bypass and frequently requires longer aortic cross clamp times, all of which can result in a greater inflammatory response. Table 17-4 summarizes clinical management issues during the major phases of CPB.
TABLE 17-4 Checklist for Bypass Management
1. Check temperature; maintain normothermia during induction and preparation.
3. Ensure noninvasive monitoring: blood pressure, ECG, pulse oximetry, stethoscope.
4. Perform inhalational induction after preoxygenation; intravenous induction, if cannula is in place.
5. Peripheral intravenous placement(s)
6. Neuromuscular blockade and ventilation
7. Intubation and mechanical ventilation according to shunt lesion (CO2, O2 control)
10. Deepening of anesthetic level
11. Antifibrinolytics and corticosteroids, as indicated
12. Heparin, 300-400 U/kg, before arterial cannulation
13. Check activated clotting time >400 seconds.
1. Stop ventilation and drips when full flow is reached.
3. Evaluate quality of perfusion (perfusion pressure, central venous pressure, diuresis, arterial blood gases, temperature gradient).
5. Set and control temperature and rewarming (heating blanket, room temperature).
7. Check arterial blood gases in preparation for discontinuation of CPB; correct abnormalities.
2. Fine-tune blood pressure; consider direct blood pressure measurement for hypotension at the aortic cannula; volume ± drips.
3. Consider modified ultrafiltration.
4. Check arterial blood gases.
5. Evaluate with transesophageal echocardiography for residual defects.
6. Give protamine, 1-1.3 mg/100 IU of initial heparin.
7. Check activated clotting time and arterial blood gases.
8. Chest closure and recheck arterial blood gases.
CPB, Cardiopulmonary bypass; ECG, electrocardiogram; TEE, transesophageal echocardiography.
Particular Aspects of Management on Cardiopulmonary Bypass
pH-Stat Versus α-Stat Management
Some degree of hypothermia is used for nearly every cardiac operation to slow the metabolism and oxygen consumption of all organs, particularly the brain and heart.122 During cooling, the carbon dioxide contained in blood becomes more soluble and its partial pressure decreases. The Paco2 sensed by the body decreases as body temperature decreases, with the result that at a core temperature of 17° C to 18° C, if pH and Paco2
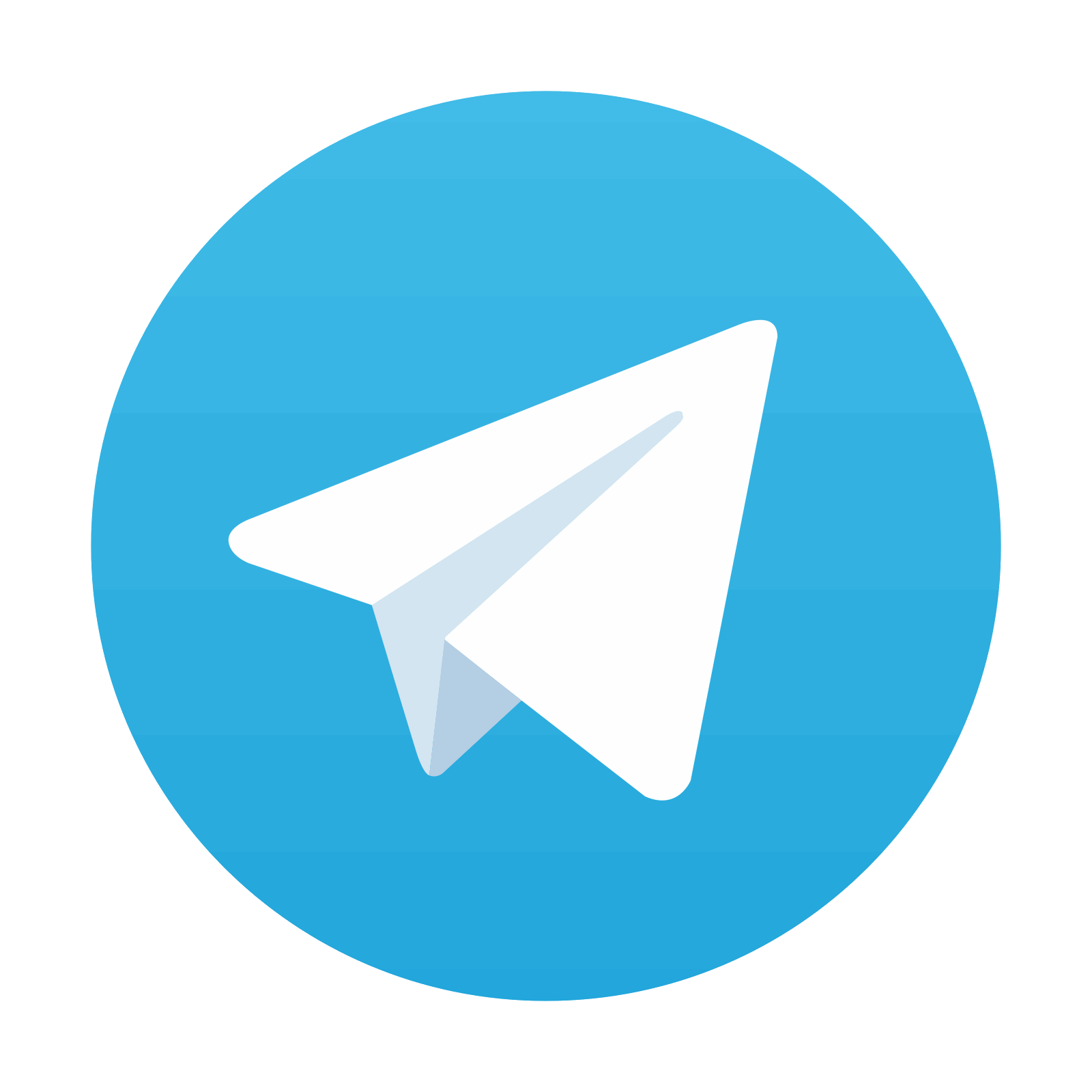
Stay updated, free articles. Join our Telegram channel

Full access? Get Clinical Tree
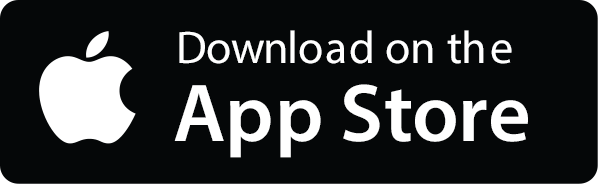
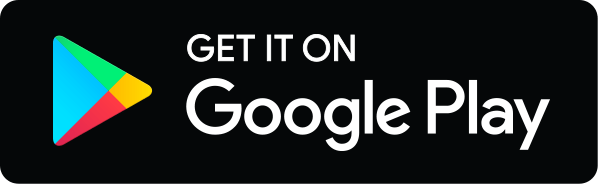