FIGURE 45.1 Neurohumoral and mechanical events that lead to death in patients with cardiogenic shock. ANP, atrial natriuretic peptide. (Used with permission from Francis GS. Neuroendocrine manifestations of congestive heart failure. Am J Cardiol. 1988;62(Suppl):9A–13A.)
The Killip classification on uses pure clinical bedside evaluation of the patient to establish prognostic indicators to predict the mortality associated with an acute MI using the physical findings of congestive heart failure (5).
- Class I patients developed no overt signs of congestive heart failure, and these individuals had a low in-hospital mortality rate. This subgroup represented approximately 40% to 50% of all patients who presented with an acute MI. The in-hospital fatality rate was approximately 6%.
- Class II patients demonstrated evidence of impaired ventricular function as manifest by persistent bibasilar rales and an audible third heart sound. This subset of patients accounted for approximately 30% to 40% of patients with acute MI. The in-hospital mortality rate of 17% was triple relative to Class I patients.
TABLE 45.1 Contributing Factors to the Development of Cardiogenic Shock in Myocardial Infarction |
![]() |
- Class III patients were characterized by the development of acute pulmonary edema, which was seen in approximately 10% to 15% of patients admitted to the hospital. A significant mortality rate of 38% was seen in this group treated conservatively before the thrombolytic era.
- Class IV patients had established cardiogenic shock with hypotension and signs of organ hypoperfusion. Cardiogenic shock occurred in 5% to 10% of infarct patients in this series but was associated with a high in-hospital mortality rate of 80%, which was a function of both severity of the underlying illness plus the limited availability of definitive treatment at the time this classification was proposed.
The group at Cedars Sinai Medical Center Los Angeles, also developed a clinical classification of heart failure associated with acute MI, which was subsequently refined by the availability of invasive hemodynamic monitoring using pulmonary artery catheters (PACs; Table 45.2) (10). The Cedars Sinai classification also subdivided patients with acute myocardial into four subsets based on the measurement of the PAOP, cardiac index (CI), and clinical assessment.
TABLE 45.2 Hemodynamic Subsets and Mortality in Myocardial Infarction | |||
![]() |
Class I patients had no clinical evidence of pulmonary congestion or tissue hypoperfusion. Hemodynamic parameters measured in these subjects revealed the PAOP to be less than 18 mmHg and the CI to be in excess of 2.2 L/min/m2. The advent and widespread use of pulmonary artery catheters clarified the concept of the ideal wedge that established the impact of diastolic dysfunction secondary to acute ischemia, with resultant impaired relaxation and elevated filling pressures being required to maintain adequate cardiac output.
Class I patients accounted for 25% of subjects admitted to the coronary care unit, and there was a low in-hospital mortality rate of 1%. Patients who on clinical grounds demonstrated no evidence of hypoperfusion or pulmonary congestion would not be expected to benefit from invasive cardiac monitoring. Frequent clinical reassessments; close attention paid to blood pressure and evidence of organ perfusion would represent adequate care.
Class II patients demonstrated pulmonary congestion as manifest by only an elevated PAOP greater than 18 mmHg with an associated normal cardiac index. Class II patients accounted for approximately 25% of patients admitted to the coronary care unit, but an 11% mortality rate was associated with this group. Mild pulmonary congestion is transiently seen in a significant percentage of patients admitted to the coronary care unit and has a multifactorial etiology. Diastolic dysfunction induced by ischemia with retrograde transmission of elevated filling pressures into the pulmonary venous circuit results in extravasation of fluid into the pulmonary bed when hydrostatic pressure exceeds oncotic pressure. Ischemic papillary muscle dysfunction with mild degrees of mitral insufficiency is also a potential cause of pulmonary congestion in this subgroup. Physical examination of these patients reveals mild to moderate rales and potentially an audible third heart sound associated with radiographic evidence of pulmonary venous hypertension. Dyspnea and orthopnea are the main symptoms superimposed on the clinical presentation of myocardial ischemia. Treatment in this group is centered on reduction of filling pressures to a level that relieves pulmonary venous congestion but does not result in an overzealous reduction of filling pressures below the ideal filling pressure as the reduced cardiac contractility will require some increased filling volume and pressure to maintain adequate stroke volume and perfusion pressure (Starling mechanism). Excessive diuresis should be assiduously avoided, especially in patients who were euvolemic before the onset of their infarct. Despite signs of pulmonary congestion, patients presenting with acute pulmonary congestion frequently are not intravascularly volume overloaded, and diuretic therapy may reduce filling pressures to a level that would impair cardiac output. It is often difficult to ascertain at the bedside which patients are actually euvolemic and which are hypervolemic. Afterload reduction therapy will benefit both groups of patients and may allow time to assess total effective circulating blood volume by indirect measures, such as the existence of hyponatremia, peripheral edema and S4 gallop. Inotropic agents should be considered in such a situation so that pulmonary congestion can be relieved by diuresis if afterload reduction is not immediately effective since the increased inotropic state mitigates against a reduction in cardiac output induced by any reduction in cardiac filling pressures. Oxygenation should be maintained with adequate arterial saturation that may be monitored by oximetry (e.g., SpO2 >90%). Vasodilator therapies in the form of nitroglycerin or inotropic agents with vasodilating capacity such as dobutamine are effective to return the hemodynamic parameters to normal. The usefulness and risk–benefit ratio of invasive hemodynamic monitoring in this subgroup of patients are controversial, although these patients frequently may be managed on clinical grounds.
Class III patients are characterized predominantly by clinical evidence of hypoperfusion. Hemodynamic monitoring reveals a PAOP less than 18 mmHg and a CI of less than 2.2 L/min/m2. The Class III subgroup accounted for approximately 15% of patients with acute MI and was associated with a 23% mortality rate. Patients in this subgroup may be extremely difficult to manage on clinical grounds, and treatment can be facilitated by invasive hemodynamic monitoring to establish the volume status. Relative hypovolemia is determined by measuring the PAOP, which falls below that of the ideal filling pressure as predicted in ischemic states. Excessive diuresis is extremely problematic in this group of patients and may further decrease cardiac output because of the preexistent relative hypovolemia. Class III patients require restoration of intravascular volume to increase filling pressures to a degree that ensures adequate cardiac output and organ perfusion.
Class IV patients demonstrated elevated PAOP in excess of 18 mmHg and a depressed CI of less than 2.2 L/min/m2 and frequently manifested signs of cardiogenic shock with clinical evidence of organ hypoperfusion and dysfunction. This subgroup accounted for approximately 35% of patients with MI and was associated with an in-hospital mortality rate of approximately 50%. Class IV patients may have a mechanical defect such as acute mitral insufficiency, free wall rupture, or VSD underlying the acute MI; these are discussed separately. Oxygenation with the potential assisted ventilation in addition to inotropic and judicious use of vasodilator support is the recommended therapy in this subgroup.
Right Ventricular Infarction
Although isolated right ventricular (RV) infarction is rare, evidence of RV infarction and RV dysfunction is found in up to half of all infarcts and is clinically significant in nearly half of all inferior infarcts (11,12). The clinical diagnosis of RV infarction should be considered when elevated jugular venous pressure is accompanied by hypotension, while the lung fields are clear. But the diagnosis may be difficult to establish clinically unless hemodynamic measurements, special electrocardiographic leads, echocardiography, or nuclear imaging are performed (13). Right-sided precordial leads obtained by electrocardiography that demonstrates at least 1-mm ST elevation is approximately 70% sensitive in the diagnosis of RV infarction and confers a particularly poor prognosis (14). Echocardiography is an easily obtainable noninvasive study that demonstrates RV dilation and RV wall motion impairment. Radionuclide angiography currently is considered to be the most sensitive means to diagnose RV infarction, although more recent data suggest magnetic resonance imaging is comparable (15,16). A decrease in RV ejection fraction that is associated with wall motion abnormalities is more than 90% sensitive in the diagnosis of an RV infarction. Hemodynamic studies which are supportive of significant ischemic involvement of the right ventricle are manifested by increases in right atrial pressures plus demonstration of resistance to diastolic filling, as shown by blunting of the y-descent that follows tricuspid valve opening. A “square root” sign or “dip and plateau” pattern in the diastolic pressure curve is commonly demonstrated in RV infarctions but is not specific and may be associated with pericardial tamponade or restrictive cardiomyopathy (17).
The SHOCK trial registry reported on the clinical characteristics of patients presenting with isolated RV shock (18). Patients with RV shock compared to LV shock were younger, had a lower prevalence of previous MI (25.5% vs. 40.1%), a lower prevalence of anterior MI (11% vs. 59%), and a less multivessel disease (34.8% vs. 77.8%). As expected, the infarct-related vessel involved the right coronary artery more in RV shock (96% of cases) versus LV shock (27% of cases). These patients had a shorter median time between MI and the diagnosis of shock (2.9 vs. 6.2 hours) compared to patients with LV shock. Right atrial pressure was a highly significant distinguisher of right from LV shock (mean pressure 23.0 ± 9.9 vs. 14.2 ± 7.4 mmHg, p = 0.0001), while all other hemodynamic measures were similar. Interestingly, in-hospital mortality was not significantly different between RV and LV shock (53.1% vs. 60.8, respectively). Improvement in survival due to revascularization was similar between groups and multivariate analysis revealed that RV shock was not an independent predictor of lower in-hospital mortality (odds ratio 1.07, 95% confidence interval 0.54 to 2.13). This similarity in survival was despite patients with RV shock being younger, thus RV shock may carry a worse prognosis.
Cardiogenic shock in patients with RV infarction frequently represents a substantial loss of functioning myocardium and carries a poor prognosis. RV infarction accompanied by cardiogenic shock is frequently associated with a variety of conduction abnormalities, including a high-grade atrioventricular block or significant rhythm disturbances. The treatment of RV infarction complicated by cardiogenic shock centers around maintaining RV filling pressures and assurance of adequate volume. Hemodynamic measurements, including echocardiography, may facilitate the estimate of volume loading required. Nitrates, diuretics, and other predominantly vasodilating compounds should be avoided. Atrial fibrillation is frequently poorly tolerated by these patients and may require immediate electrical cardioversion. The use of digitalis in acute RV infarction, even in the presence of atrial fibrillation, is controversial. Adequate inotropic support with vasodilating inotropic agents such as dobutamine is used if cardiac output fails to optimize after adequate volume loading. Percutaneous revascularization should be considered as it has been shown to improve outcomes (19).
Mechanical Defects
A variety of mechanical defects may be associated with cardiogenic shock in the periinfarction stage (Table 45.3). MI resulting in cardiogenic shock from the appearance of mechanical defects such as acute mitral insufficiency, VSD, or free wall rupture represents a major complication and requires aggressive diagnostic and therapeutic interventions if the patient is expected to survive. Despite improvements in imaging techniques plus mechanical assist devices and emergency surgery, the mortality from these complications remains extremely high.
Acute Mitral Insufficiency
The mitral valve is a complicated apparatus and consists of the valvular annulus, leaflets, chorda tendineae, and papillary muscles plus potential functional alterations from involvement of the adjacent myocardium. Abnormalities affecting any of the components of the mitral valve may result in acute or chronic mitral insufficiency. The mitral valve annulus may be dilated and contribute to mitral insufficiency, although this complication is primarily associated with cardiomyopathies or connective tissue diseases such as Marfan syndrome rather than an acute MI. Calcification of the mitral valve annulus is common in the elderly and may alter coaptation of the mitral valve leaflets and result in mitral incompetence.
Acute mitral insufficiency caused by involvement of the valvular leaflets is associated with infective endocarditis from necrotizing organisms such as Staphylococcus aureus or Enterococcus, resulting in destruction of the valvular apparatus. Traumatic penetrating injuries that involve the valve itself are rare. Rupture of the chorda tendineae may also be seen in endocarditis or a variety of connective tissue diseases, including myxomatous degeneration or Marfan syndrome.
Chordal rupture that results in severe impairment of LV function depends on the number of involved structures and the rapidity with which the rupture occurs. Mitral insufficiency in the periinfarction state may result from involvement of the surrounding myocardium or papillary muscles. Papillary muscles located adjacent to the infarction zone may simply become dysfunctional because of alteration of synchrony of contraction related to ischemia or frank rupture from ischemic necrosis.
The degree of mitral insufficiency is a function of the degree of involvement and anatomic competence. The two papillary muscles (posteromedial and anterolateral papillary muscles) have different ischemic vulnerabilities because of the blood supply from the coronary arteries. The anatomic vascular supply represents end arteries that are solely supplied by terminal portions of the coronaries, thus rendering the papillary muscles vulnerable to ischemic involvement during an acute MI. Papillary muscle dysfunction may result from intermittent ischemia during unstable angina or MI with involvement of the adjacent myocardium (20). Papillary muscle dysfunction is characterized by mild flow murmurs which may be grade I or grade II by auscultation. The anterolateral papillary muscle has a dual blood supply, which provides partial protection during ischemia. The diagonal branches of the left anterior descending and marginal branches from the circumflex supply blood to the anterolateral papillary muscle. The posteromedial papillary muscle is generally supplied solely from the posterior descending branch of the right coronary artery increasing its vulnerability to ischemic-related dysfunction.
TABLE 45.3 Complications of Myocardial Infarction | |||
![]() |
Significant ischemia involving the papillary muscle that results in complete rupture with fulminant mitral insufficiency is generally fatal because of the marked volume load ejected retrograde into the left atria and pulmonary venous bed (21). However, if the major ischemia-related necrosis is distal and only involves rupture of the head of the papillary muscle, the resultant mitral insufficiency may be tolerated hemodynamically long enough to allow recognition, proper diagnosis, and surgical intervention. Mild ischemic involvement of the papillary muscle may be increased in hemodynamic significance in the presence of pre-existing LV dilation, which alters the ability of the mitral leaflets to coapt. Severe ischemia-related mitral insufficiency is more frequently a result of posteromedial papillary muscle necrosis resulting from inferior or posterior MIs although one-third of cases may result from anterior infarction (21,22). Less than half of cases present with electrocardiographic evidence of ST elevation or Q waves (22). RV papillary muscle rupture may occur but is uncommon. Involvement of papillary muscles in the right ventricle results in tricuspid insufficiency, which if severe may result in RV failure.
Papillary muscle rupture is a relatively uncommon complication and occurs in about 1% of patients having an acute ischemic event. The incidence has decreased in the thrombolytic era (23). After acute MI with cardiogenic shock, the incidence of acute severe mitral regurgitation is 6.9% (24). The peak incidence of papillary muscle rupture is within the first week with the majority occurring between days 3 and 5 after an acute MI. The diagnosis of papillary muscle rupture may be suspected on physical examination and has been facilitated with the advent of hemodynamic monitoring and echocardiography.
The physical examination in acute mitral insufficiency secondary to papillary muscle rupture differs from the findings associated with chronic valvular regurgitation. In the acute setting a palpable thrill is uncommon and the radiation of the murmur differs from chronic conditions. The systolic murmur is soft, decrescendo, generally ends before the second heart sound, and is best audible at the base of the heart as opposed to the apex with radiation to the neck or the top of the head.
Echocardiography and Doppler ultrasound has been a major advance in the diagnosis of acute mitral insufficiency and its clinical separation from other mechanical lesions associated with a new murmur (25). The left atrium and left ventricle are generally of normal size, and the ejection fraction is increased and frequently hyperdynamic. The mitral leaflet flails and may prolapse into the left atrium. Doppler ultrasound with color flow study determines the presence and severity of mitral insufficiency, presence of an intracardiac shunt and quantifies the degree of mitral regurgitation. Data from the SHOCK trial which randomized patients with cardiogenic shock within 36 hours of an acute MI demonstrated that the severity of mitral regurgitation quantified by Doppler echocardiography is an independent predictor of survival (26).
Pulmonary artery catheter placement with measurement of PAOP and cardiac output is useful in mitral insufficiency. The presence of a regurgitant wave in the PAOP tracing may be visible in acute mitral regurgitant lesion, especially when there is no evidence of a step-up in oxygen concentration in the right atria or right ventricle. Pulmonary artery catheterization is not necessary for diagnosis but the use of invasive monitoring allows optimization of cardiac output, filling pressures, and adjustment of inotropic, vasodilator and diuretic therapy on the basis of induced changes in pressures.
Ventricular Septal Defect
Rupture of the interventricular septum may present in a similar clinical manner as mitral insufficiency with the abrupt onset of congestive heart failure plus a new murmur, making the two conditions difficult to separate on clinical grounds. Rupture of the interventricular septum also occurs in the first week after the acute ischemic event with a peak incidence occurring between days 3 and 5. The prevalence rate of acute ventricular septal defects (VSDs) after an infarction is difficult to accurately determine but occurs within the range of 0.5% to 2.0% and is the cause of death in approximately 5% of all fatal MIs. Incidence of VSD as a cause of cardiogenic shock after acute MI in the SHOCK trial registry was 3.9% (24). Blood supply to the septum is supplied by septal perforating branches of the left anterior descending vessel and acute VSD is more common in anterior MIs. These patients frequently have multivessel disease and are older patients experiencing an initial MI (27).
The diagnosis of acute VSD may be inferred on clinical grounds but frequently requires more sophisticated evaluation to accurately diagnose and quantify the defect which is located in the muscular septum and may be multiple. The physical examination in acute VSD depends on the magnitude of the shunt, which is, in turn, a function of the size of the ventricular defect, RV compliance, pulmonary artery pressures, and the inotropic state. A significant VSD is associated with the characteristic findings of shock in addition to a new holosystolic murmur associated with a precordial thrill. A precordial thrill may be palpated in approximately 50% of patients with an acute VSD and is a function of the magnitude of pressure gradient between the two chambers.
The diagnosis of VSD and its separation from acute mitral insufficiency has been greatly facilitated by the advent of noninvasive and invasive diagnostic procedures. Two-dimensional echocardiography combined with Doppler flow study generally identifies a significant defect (28). Contrast echocardiography using microbubble techniques also may aid in the diagnosis of acute VSD and establish the presence of an intracardiac shunt. Pulmonary artery catheterization demonstrates the absence of a V wave in the PAOP tracing and an increase in oxygen saturation by about 10% in the right ventricle compared with the right atrium. The mortality rate for septal defects is significant, with approximately 25% of patients dying within the first 24 hours and a 50% mortality rate at 1 week. Less than 10% survive 1 year when treated solely with medical therapy (29). When occurring in the setting of cardiogenic shock, in-hospital mortality for MI patients with VSDs has been reported as high as 87% (24).
Free Wall Rupture and Tamponade
Free wall rupture is a major complication of MI and is difficult to diagnose premortem. The prevalence of this complication is unknown but may occur in up to 8% of all MIs with approximately one-third occurring in the first 24 hours after the onset of the ischemic event and the peak incidence between days 5 and 7 (30). The SHOCK trial registry reported a 1.4% incidence of free wall rupture as a cause of cardiogenic shock after acute MI (24). Rupture of the free wall is a major cause of mortality in acute ischemic events and is associated with large transmural infarcts with inadequate collateral circulation. This serious complication occurs more commonly in elderly hypertensive patients. Involvement of the left ventricle is the rule, although free wall rupture involving the right ventricle has been reported. Rupture of the free wall is frequently associated with the ventricular remodeling process in which a segmental infarction results in elevated LV and diastolic pressure with expansion of the infarcted area. Expansion involves thinning of the affected area with regional hypertrophy in the adjacent region surrounding the infarct. A disproportionate dilatation occurs in the infarcted area and the risk of free wall rupture is enhanced with high shearing forces and elevated pressures. Free wall rupture generally occurs in the border zone between the infarcted area and the normal surrounding myocardium. The advent of thrombolytic therapy has been postulated to potentially increase the risk of free wall rupture although not definitely confirmed. Thrombolytic therapy may actually minimize the extent of myocardial necrosis and decrease free wall rupture. The use of agents such as corticosteroids, previously used to blunt inflammatory response and infarct size, has been associated with increased risk of free wall rupture.
Cardiac rupture is a catastrophic event resulting in sudden cardiac death unless a pseudoaneurysm forms. Hemopericardium with cardiac tamponade is difficult to diagnose early enough to institute definitive therapy. Cardiac tamponade after acute MI also may be secondary to hemorrhagic pericarditis but massive hemopericardium is usually due to cardiac rupture with rapid development of electromechanical dissociation and death. The diagnosis of free wall rupture is difficult but should be suspected with sudden hypotension, elevated jugular venous pressures, muffled heart sounds, and a pulsus paradoxus. Echocardiography can document the presence of pericardial fluid and occasionally demonstrates the perforated free wall (31,32). The classic signs of tamponade are present on echocardiography and are caused by the rising intrapericardial pressure compressing the right atrium and right ventricle, resulting in equalization of pressures and RV diastolic collapse. Definitive therapy involves pericardiocentesis plus volume and pressure support with early surgical intervention being necessary for salvage. Untreated free wall rupture is universally fatal although isolated instances of successful aggressive intervention with surgical therapy have been reported (33).
Left Ventricular Aneurysm
LV aneurysm is a relatively common complication of MI and may occur in up to 15% of MI survivors (34). A true aneurysm has a wide base with the ventricular walls composed entirely of myocardium, compared with a pseudoaneurysm, which generally has a narrow base with the walls consisting of pericardium and thrombotic debris. True aneurysms have a relatively low risk of free wall rupture but are associated with increased mortality due to sudden death from ventricular arrhythmias, emboli from mural thrombus, and progressive loss of LV contractile function (35). Aneurysms may develop early in the postinfarction period and can be asymptomatic or present with significant deterioration of LV function. The presence of LV aneurysm may be inferred by persistent ST elevation in the absence of chest pain or enzyme leakage (36).
Echocardiography demonstrating dyskinesis is a valuable tool in diagnosing aneurysms as is LV angiography. LV angiography demonstrates paradoxic systolic distention during ventricular contraction. Successful treatment of the aneurysm may be achieved with resection of the involved myocardium, frequently in combination with saphenous vein or mammary artery bypass grafting because of the high associated prevalence of multivessel coronary artery disease. Surgical resection has been advocated in the presence of post-MI arrhythmias to eliminate the substrate for ventricular tachycardia, but electrophysiologic mapping techniques are necessary to demonstrate that the origin of the arrhythmia arises from the LV aneurysm.
DIAGNOSIS
The clinical manifestations of cardiogenic shock are a function of the underlying cause, and mechanical defects must be aggressively sought because the need for definitive therapy is differs markedly different by cause (Fig. 45.2). Clinical recognition of the shock syndrome frequently requires prompt and aggressive stabilization procedures to be instituted before the definitive diagnosis of the underlying cause. A history and physical examination should be obtained with special attention to mental status, jugular venous pulsations, quality and intensity of heart sounds, presence and localization of a murmur, and presence of oliguria. Diagnostic tests such as electrocardiogram, portable chest radiograph, arterial blood gases, and echocardiography frequently provide adequate clinical information to make a diagnosis and initiate stabilization therapy. A quarter of patients presenting with cardiogenic shock secondary to predominant LV dysfunction do not have evidence of pulmonary congestion (37).
TREATMENT
Percutaneous Revascularization
Prior to 1999, interventions for the management of cardiogenic shock complicating acute MI were not systematically studied. The landmark SHOCK trial demonstrated that a strategy of early revascularization by angioplasty or surgery reduced mortality from 63% to 50% at 6 months (6). This finding has resulted in a major paradigm shift in the management of cardiogenic shock. The first branch-point in the decision algorithm is whether or not shock is present in the setting of an acute MI. If shock is present, patients should undergo immediate coronary angiography with percutaneous intervention if feasible. Primary coronary artery stenting is now recommended for patients with ST elevation or left bundle branch block who develop shock and are suitable for revascularization, irrespective of time delay and need for transfer to a facility capable of coronary intervention (38,39). Thrombolytic therapy may be used if patient is not considered a candidate for percutaneous intervention (39,40).

FIGURE 45.2 Management algorithm for cardiogenic shock. ACE-I, angiotensin converting enzyme inhibitor; ARB, angiotensin II receptor blocker.
The SHOCK trial studied patients with onset of shock within 36 hours of an MI and randomized the patients to immediate revascularization versus initial medical stabilization. Almost all patients required inotropes or vasopressors. Treatment in the revascularization group (64% of patients) was angioplasty or stenting (stents were not available at the beginning of the trial in 1993, but were actively used by the end of the trial in 1998) and coronary artery bypass graft surgery in 36%. In a subgroup analysis, survival was similar between percutaneous and surgically revascularized patients (55.6% vs. 57.4% at 30 days and 51.9% vs. 46.8% at 1 year, respectively) despite a higher incidence of diabetes and multivessel disease in those patients surgically revascularized (41).
Thrombolytic therapy was used in 49% of patients in the revascularization group and in 63% of the medical therapy group. There was no difference in survival at 30 days (53.3% in the revascularization group vs. 44.0% in the medical therapy group), likely a result of improved medical therapy. Age over 75 years was associated with significantly higher 30-day mortality. Follow-up reports have shown persistent benefit to early revascularization with survival rates of 47% versus 34% at 1 year, 33% versus 20% at 6 years (42,43). Of the patients surviving to hospital discharge (143/302), 6-year survival was 62% versus 44% (43).
An exciting aspect of the SHOCK trial was the registry that was created from patients screened for enrollment but not randomized, whose data were reported separately in a dedicated supplement to J Am Coll Cardiol in September 2000. This registry described 1,190 patients and is the largest prospectively collected database for cardiogenic shock (24). Etiology of shock from the registry was LV failure (78.5%), acute severe mitral regurgitation (6.9%), ventricular septal rupture (3.9%), isolated RV shock (2.8%), and tamponade from free wall rupture (1.4%). Electrocardiographic site of infarction was anterior (55%), inferior (46%), posterior (19%), lateral (32%), apical (11%), with multiple sites present in half of the cases. There was ST elevation, Q waves, or new left bundle branch block in 79% of cases. Systolic blood pressure averaged 88 mmHg with a mean heart rate of 96/min. Of the subset of patients with invasive hemodynamics measured, PAOP was 23 mmHg, CI was 2.08 L/min/m2, and LV ejection fraction was 33%. In-hospital mortality averaged 60% and ranged from 55% for acute severe mitral regurgitation, isolated RV shock, and tamponade to 87% for ventricular septal rupture. Of the 717 patients who underwent coronary angiography, 15.5% had significant left main stenosis, 53.4% had three-vessel disease. Coronary artery disease severity also correlated with in-hospital mortality: no or single-vessel disease was associated with a 35% mortality rate as compared with three-vessel disease with a mortality rate of 50.8% (44).
Since the SHOCK trial, stenting has replaced angioplasty alone as the primary treatment for ischemic coronary artery disease because it carries a reduced incidence of restenosis. One recent case series has shown that stenting for cardiogenic shock decreased mortality compared to angioplasty alone (from 68% to 43%) (45). Primary coronary artery stenting is now recommended for patients with ST elevation or left bundle branch block who develop shock within 36 hours of acute MI and are suitable for revascularization that can be performed within 18 hours of shock onset (38). Thrombolytic therapy may be used if early revascularization is not available (40).
While drug-eluting stents which slowly elute a pharmacologic agent (currently either sirolimus or paclitaxel) are now widely used instead of bare metal stents due to their proven efficacy in reducing the incidence restenosis, to date they have not been studied in the setting of cardiogenic shock (46,47).
Pharmacologic Limitation of Infarct Size
Several pharmacologic interventions have been used during acute MI to minimize the extent of irreversible ischemic damage and decrease the likelihood of subsequent development of cardiogenic shock. Quantitative measurements of the extent of myocardial damage by electrocardiographic mapping and creatine kinase (CK) release are imprecise and frequently limit quantitative assessment of the potential therapeutic impact of pharmacologic interventions. Calcium channel blockers, beta-adrenergic receptor blockers (β-blockers), and nitrates have been the main agents that have undergone clinical analysis to minimize myocardial damage, whereas a variety of experimental or uncommonly used therapies have been evaluated in small-scale clinical trials. Nitrates are complex pharmacologic agents with arterial and venodilating activity in addition to other potential beneficial effects, such as alteration of prostacyclin metabolism. Nitrates when administered as topical, oral, or sublingual agents, are predominately venodilators with subsequent venous pooling, decreased venous return, and lowering of PAOP. Reduction in venous return and optimization of PAOP decreases LV volume and improves subendocardial perfusion, thus reducing wall stress with the potential for minimizing infarct extent. Nitrates also have effects on systemic vascular resistance and epicardial coronary arteries, with resultant reduction of impedance to LV ejection and increase in coronary blood flow.
Intravenously administered nitroglycerin has a more balanced arterial and venodilating effect. Clinical trials demonstrate that intravenous nitroglycerin administered at a level to decrease mean aortic pressure by 10% (48), results in a decrease in extension of MI, improves LV ejection fraction and survival (49). Intravenous nitrates minimize the magnitude of infarct size as monitored by CK, and alter infarct expansion with reduction in the subsequent remodeling process and progression to congestive heart failure. Intravenous nitrates are potent vasodilators and require careful blood pressure monitoring to prevent significant hypotension and paradoxical bradycardia. Nitrates may result in a beneficial redistribution of coronary flow to the subendocardium without the coronary steal syndrome, a major detriment of other potent intravenous vasodilators such as nitroprusside.
Calcium channel blockers are important agents in managing patients with classic and vasospastic angina. The calcium channel blocking agents decrease systemic vascular resistance, decrease oxygen demand and increase coronary flow, improving the balance between supply and demand. At pharmacologic doses, these agents also may have other potentially beneficial effects including antiplatelet activity.
Despite the documented beneficial effect of these agents in hypertension and angina, calcium channel blockers have not been proven to be beneficial in the treatment of MI and do not definitely limit infarct size. Studies using nifedipine have been unable to demonstrate benefit in patients with acute MI. Diltiazem has been advantageous in non–Q wave infarction in the Diltiazem Reinfarction Study (50). However, the Multicenter Diltiazem Postinfarction Trial was not able to document a benefit to the administration of diltiazem in the postinfarction state when compared with placebo (51). Subgroup analysis demonstrated a mortality benefit with diltiazem therapy when no pulmonary congestion was present. However, mortality was increased when diltiazem was administered to subjects whose infarction was complicated by pulmonary congestion implying that this agent should not be used in patients with cardiogenic shock. Studies performed in Denmark using intravenous verapamil followed by oral administration did not demonstrate a benefit. Later studies using only oral verapamil demonstrated a mortality reduction although these trials have not been reconfirmed (52). Currently, the evidence for using calcium channel blockers for the treatment of acute MI to limit infarct size and progression to cardiogenic shock is limited.
β-Adrenergic blocking agents have been used in treating hypertension, atrial fibrillation, and a variety of ischemic conditions. β-Blockers act predominantly by decreasing myocardial oxygen demand caused by the negative chronotropic and inotropic activities of these agents. β-Blockers may have several other potentially beneficial effects including antiplatelet activity, regression of LV hypertrophy, and reduction in sudden cardiac death. Clinical trials using β-blockade in acute MI have yielded conflicting results. The Goteborg trial administered metoprolol or placebo to subjects having an acute MI and demonstrated a significant reduction in mortality at 90 days in the group randomly assigned to β-blocker therapy (53). Early administration of metoprolol was associated with a reduction in estimated infarct size, which presumably has an effect on early and long-term survival. Despite the fact that β-blockers are not commonly used as antiarrhythmic agents, there was a documented decrease in sudden cardiac death in the β-blocker group, which has been shown to be secondary to an increase in ventricular fibrillatory threshold.
The Metoprolol in Acute Myocardial Infarction trial was able to demonstrate that the early administration of intravenous metoprolol followed by oral maintenance dose in acute MI was associated with a decrease in mortality in a high-risk subgroup of infarct patients (54). A subgroup study of the Goteborg Metoprolol trial found that early treatment with metoprolol in patients with suspected acute MI and signs of heart failure resulted in significantly reduced mortality at 3 months (10% vs. 19%) which persisted to 1 year (14% vs. 27%) compared to those who did not receive metoprolol (55). Propranolol, a noncardioselective β-blocker, has not uniformly been demonstrated to decrease mortality or limit infarct size when administered early in acute MI patients. However, the Beta-Blocker Heart Attack trial demonstrated reduced mortality when propranolol was administered after the acute phase of the infarction had subsided (56). Intravenous atenolol was studied in the First International Study of Infarct Survival trial and demonstrated a 15% reduction in the early mortality of infarct patients who were given oral atenolol after the intravenous loading doses (57). β-Blockers also have been combined with thrombolytic therapy to limit infarct size. The Thrombolysis in Myocardial Infarction trial (TIMI II-B) studied the impact of three 5-mg boluses of metoprolol administered at 5-minute intervals followed by oral metoprolol compared with thrombolysis plus oral metoprolol. The TIMI II-B trial demonstrated a decrease in nonfatal reinfarctions and recurrent ischemic episodes in the group who received immediate intravenous metoprolol followed by oral therapy compared with the delayed subgroup. More recently, the CAPRICORN (Carvedilol Post Infarct Survival Control in Left Ventricular Dysfunction) trial studied carvedilol (6.25 to 25 mg twice a day in addition to standard therapy of aspirin, angiotensin-converting enzyme [ACE] inhibition, and thrombolysis) versus placebo in a high-risk group of acute MI patients (n = 1,959) with LV ejection fraction of 40% or less. Patients were treated for a mean of 1.3 years. All-cause mortality was lower in the carvedilol group than in the placebo group (58). Patients who had echocardiography demonstrated significantly higher LV ejection fraction and decreased LV end-systolic volume in the carvedilol group at 6 months (59). Another post hoc analysis of CAPRICORN study found that carvedilol suppressed atrial arrhythmias (2.3% vs. 5.4%) as well as ventricular arrhythmias (0.9% vs. 3.9%) compared to the control group (60). The beneficial effect on ventricular remodeling, in addition to the antiarrhythmic effect may be one of the mechanisms by which carvedilol decreased mortality after acute MI in patients treated with ACE inhibitors. Use of β-blockers in acute MI, while now standard of care, must be undertaken with caution because of the potential of precipitating atrioventricular block, reactive airways disease, and hypotension (61).
ACE inhibitors have been administered orally and intravenously in clinical trials to halt progression to congestive heart failure in the SAVE (Survival and Ventricular Enlargement) and Consensus-II trials. The SAVE study used captopril in over 2,000 patients who had an acute anterior MI when enrolled during the period from 3 to 16 days after the acute myocardial event (62). All patients with ejection fractions below 40% were randomized to receive oral captopril or a placebo. Patients receiving captopril demonstrated less congestive heart failure, less recurrent MIs, less hospitalizations, and improved mortality over a 42-month period. The Consensus-II (Cooperative New Scandinavian Enalapril Survival Study) trial used intravenous enalapril in the early phase of infarction followed by oral enalapril but there was no mortality benefit when compared with placebo (63). A review of the major post-MI heart failure trials such as SAVE, AIRE (Acute Infarction Ramipril Efficacy), TRACE (Trandolapril Cardiac Evaluation) between 1992 and 1995, calculated that ACE inhibitors produced a relative risk reduction of 16% while β-blockade in addition to ACE inhibition in CAPRICORN trial demonstrated an additional relative risk reduction of 23% (64). Oral ACE inhibitors are attractive agents because of their effects on hemodynamics, microcirculation, and angiotensin-mediated vasoconstriction and should be administered especially in anterior infarcts with significant reductions in ejection fraction unless there are contraindications (hyperkalemia, known drug sensitivity).
Selective aldosterone blockade with eplerenone for patients with LV ejection fraction of 40% or less after acute MI has been studied in one large, placebo-controlled trial EPHESUS (Eplerenone Post-Acute Myocardial Infarction Heart Failure Efficacy and Survival Study). Eplerenone (in addition to treatment with β-blockers and ACE inhibitors) reduced all-cause mortality by 15%, cardiovascular mortality by 17%, heart failure hospitalizations by 23%, and sudden cardiac death by 21% (65). These outcomes were even more marked in the subgroup of patients with LV ejection fraction of 30% or less. In this group, all-cause mortality was reduced by 21%, cardiovascular mortality by 23%, sudden cardiac death by 33%, and heart failure mortality or hospitalization by 25% (66).
Angiotensin receptor blockade for LV ejection fraction of 40% or less after acute MI has been studied in the VALIANT (Valsartan in Acute Myocardial Infarction Trial) trial (67). This was a multicenter, double-blind, randomized, active-controlled, parallel-group study comparing the efficacy and safety of long-term treatment with valsartan, captopril, and their combination in high-risk patients after MI. This compared three treatment groups consisting of patients receiving standard therapy plus valsartan (n = 4,909), valsartan plus captopril (n = 4,885), or captopril alone (n = 4,909). Valsartan treatment alone resulted in similar outcomes as captopril treatment alone and thus these agents can be used interchangeably. The combination of valsartan plus captopril increased the rate of adverse events (hypotension and renal dysfunction more commonly with valsartan; cough, rash, and taste disturbance more commonly with captopril) with no change in survival.
Adjunctive antiplatelet therapy with the glycoprotein IIb/IIIa inhibitor abciximab during emergent coronary artery stenting for cardiogenic shock has been shown to reduce mortality from 43% to 33% in one case series (45). The glycoprotein IIb/IIIa inhibitor eptifibatide used for non–ST-elevation MI or unstable angina in the PURSUIT (Platelet Glycoprotein IIb/IIIa in Unstable Angina: Receptor Suppression in Using Integrilin Therapy Trial) reduced 30-day mortality in the subset of patients developing cardiogenic shock (68). Bivalirudin may be used as an antiplatelet agent for acute MI instead of a glycoprotein IIb/IIIa inhibitor, however it has had mixed results in cardiogenic shock and so is left to the operator’s discretion (69,70). Clopidogrel in addition to aspirin is now standard of care after percutaneous coronary intervention and has been shown to decrease 1-year mortality in the setting of ST-elevation MI (71). However, clopidogrel significantly increases the risk of postoperative bleeding in patients requiring surgical intervention. More recent data from the ISAR-SHOCK registry suggest that prasugrel may be superior to clopidogrel in patients with acute MI complicated by cardiogenic shock (72).
Several agents have been used in small studies as adjunctive therapy in acute MI but have not reached widespread clinical use. Myocardial damage may be potentiated by the presence of reactive oxygen radicals and free radical scavengers such as superoxide dismutase or catalase may provide potential benefit. Free radical scavengers have been shown to be effective when administered before the onset of experimental infarcts and definitive clinical studies are currently ongoing.
Glucose insulin potassium infusions (polarizing solution) have been used for several years to reduce infarct size by altering free fatty acid metabolism (73). Polarizing solution consists of 300 g of glucose, 50 units of regular insulin, and 80 mMol of potassium in 1 L of water delivered at 1.5 mL/kg/hr. Ejection fraction and wall motion abnormalities have been noted to improve after administering this solution resulting in decreased mortality. Polarizing solution has not been studied extensively in double-blind, placebo-controlled trials and routine administration of this solution has not reached clinical acceptance.
Hyaluronidase may have anti-inflammatory activity and modulate the immune response postulated to play some role in the extent of infarct size. Hyaluronidase has been administered in small clinical studies and was associated with improved mortality and decreased development of Q waves implying myocardial salvage. There are no large-scale clinical trials available (74).
Thrombolysis
Thrombolysis induced by pharmacologic agents or direct angioplasty is an attractive treatment for reestablishing coronary perfusion to minimize the extent of MI and progression to cardiogenic shock. The open artery hypothesis postulates that clinical outcome is dependent on maintaining adequate coronary perfusion to minimize ischemic damage mediated by vascular occlusion secondary to an intravascular thrombus. Recent trials of coronary thrombolysis GISSI (Gruppo Italiano per lo Studio della Sopravvivenza nell’Infarto Miocardio), ISIS (International Study of Infarct Size), GUSTO (Global Utilization of Strategies to Open Occluded Coronary Arteries) demonstrate the prevalence of cardiogenic shock in approximately 2% to 3% of acute MIs on arrival to the hospital with an additional 3% to 4% subsequently developing cardiogenic shock with a combined total of 7% (3,75). Early progression to cardiogenic shock is characterized demographically by elderly patients, the presence of anterior infarctions, low ejection fractions, diabetes, and previous MIs. Despite the theoretic attractiveness of administering recombinant tissue plasminogen activators or streptokinase in patients with established or impending cardiogenic shock, the mortality associated with cardiogenic shock remains high despite thrombolytic therapy with survival rate being only 35% as reported in GISSI-I and GISSI-II trials (76). Prompt administration of thrombolytic agents within the first hour of acute MI may result in improved survival rates if reperfusion of the infarct-related artery can be sustained. Low coronary perfusion pressures in cardiogenic shock may play a potential role in the poor clinical outcome of these patients after thrombolytic therapy.
In vitro experimental infarct studies with reduced perfusion pressure have shown decreased diffusion of thrombolytic agents into clots with resultant impaired fibrinolysis (77). Enhanced pressure increases the rate of dissolution of an intravascular thrombus implying that in cardiogenic shock with systemic hypotension, a reduced transcoronary pressure gradient may decrease efficacy of thrombolytic agents. The metabolic abnormalities associated with cardiogenic shock including lactic acidosis also may alter the conversion of plasminogen to plasmin and limit the efficacy of these drugs in clot lysis. Failure from lytic agents to sustain vascular patency in patients with cardiogenic shock is an indication for early cardiac catheterization and direct angioplasty if no contraindications exist. Persistent hypotension, nonevolving ST elevation, continuing clinical evidence of myocardial ischemia, CK elevations, and clinical instability are potential indications for rescue coronary angioplasty which may result in increased survival (78). Rescue angioplasty has not been systematically studied in randomized controlled trials comparing it to thrombolytic therapy. If thrombolytic therapy does not result in establishment of coronary perfusion, angioplasty should be considered as a therapeutic option. The SHOCK trial reported 49% of patients in the revascularization group received thrombolytic therapy and the early intervention group had a survival benefit (see section above) (6). Additionally, the SHOCK trial reported a survival benefit due to thrombolytic therapy (in-hospital mortality of 54% vs. 64%) (40). Cardiogenic shock secondary to mechanical defects such as papillary muscle dysfunction also has been treated successfully with percutaneous transluminal coronary angioplasty (PTCA), resulting in improved mitral regurgitation with resolution of cardiogenic shock (79).
Thrombolytic agents should be administered to patients with acute MI who demonstrate evidence of the shock state if there are no contraindications in patients not considered candidates for percutaneous intervention (39). Failure of evidence of reperfusion is an indicator for rescue angioplasty.
Pharmacologic Agents
Inotropic Agents
The effectiveness of various inotropic agents in cardiogenic shock depends on the cause and underlying pathophysiologic mechanism of the shock state. With systemic hypotension, adequate perfusion of the coronary arteries must be maintained (Fig. 45.3).
Dopamine. Dopamine is an endogenous catecholamine with positive inotropic properties secondary to stimulation of α- and β-adrenergic receptors plus dopaminergic receptors, which have been divided into two subtypes: DA1 and DA2 (80,81). DA1 receptors are postsynaptic and induce dilation of the coronary, renal, and mesenteric vasculature. DA2 receptors are located in autonomic ganglia and in the postganglionic sympathetic nervous system. Stimulation of DA2 receptors blocks the release of endogenous catecholamines from intraneuronal storage sites. The effect of dopamine on α- and β-activity is dose related. Low infusion dosages of dopamine (2 to 5 µg/kg/min) result in positive inotropic activity secondary to stimulation of the β1-receptors. α-Receptor stimulation occurs at dosages above 10 µg/kg/min and results in a secondary increase in systemic vascular resistance caused by peripheral vasoconstriction. In addition to the inotropic effect, dopamine results in increased atrioventricular conduction from adrenergic stimulation. The effects of dopamine are thus dose dependent, and pharmacologic activity is a function of the amount of dopamine infused corrected for body weight. The individual response may be variable and unless the clinical situation warrants large pressor doses to maintain blood pressure, dopamine infusion should begin at a low rate (1 µg/kg/min) and gradually be increased to clinical responsiveness. Cardiogenic shock with low tissue perfusion accompanied by hypotension may be treated in a more aggressive manner with progressively increasing doses of dopamine at 5-minute intervals.
Low-dose dopamine infusion results in stimulation of DA2 receptors and minimal or no changes in heart rate, cardiac output, or blood pressure. Stimulation of DA2 receptors results in renal vasodilation and increases glomerular filtration rate, renal blood flow, and sodium excretion. Reduction in cardiac output in shock frequently results in shunting of blood away from the renal vasculature and induction of a prerenal state with elevated blood urea nitrogen-to-creatinine ratios and sodium retention. Dopamine reverses the redistribution of cardiac output, increases the amount of sodium presented to the loop of Henle, which allows increased efficacy of diuretics such as furosemide or bumetanide.
Medium dosing ranges of dopamine (5 to 10 µg/kg/min) result in an increase in cardiac output, which may also improve volume status by increasing renal blood flow. The cardiac effects of dopamine in this dosing range are secondary to stimulation of the β1-adrenergic receptors caused by a secondary release of norepinephrine. The effect of dopamine is indirect and depends on a pre-existent adequate storage level of endogenous catecholamines. Longstanding congestive heart failure is frequently associated with reduction in sympathetic receptors in the myocardium and the efficacy of dopamine may be limited if prolonged congestive heart failure was present before the shock syndrome. Dopamine infusion at this dose generally does not result in alterations of venous return secondary to venodilation, and right atrial and PAOP may not decrease. Dopamine may be combined with either direct vasodilating compounds or other inotropic agents such as dobutamine, which combine inotropism with vasodilation. Medium dosing range infusions of dopamine are generally safe and effective in maintaining blood pressure. Acid–base status and electrolyte levels should be optimized to avoid potential induction of arrhythmias with resultant malignant ventricular arrhythmias or marked sinus or supraventricular tachycardias, which would increase myocardial oxygen demand.

FIGURE 45.3 Mechanisms of action of inotropic drugs. β1AR, β1-adrenergic receptor; β2AR, β2-adrenergic receptor; AC, adenyl cyclase; AMP, adenosine monophosphate; ATP, adenosine triphosphate; Ca2+, calcium; CaMK, calmodulin-activated kinase; cAMP, cyclic AMP; Gi, inhibitory G protein with α, β, and γ subunits; Gs, stimulatory G protein with α, β, and γ subunits; P, phosphorus; PDEc, cytosolic phosphodiesterases; PDEp – III, particulate, SR-associated PDE III; PHLMBN, phospholamban; PKA, cAMP-dependent protein kinase A; SR, sarcoplasmic reticulum. (Used with permission from Garcia Gonzalez MJ, Dominguez Rodriguez A. Pharmacologic treatment of heart failure due to ventricular dysfunction by myocardial stunning: potential role of levosimendan. Am J Cardiovasc Drugs. 2006;6:69–75.)
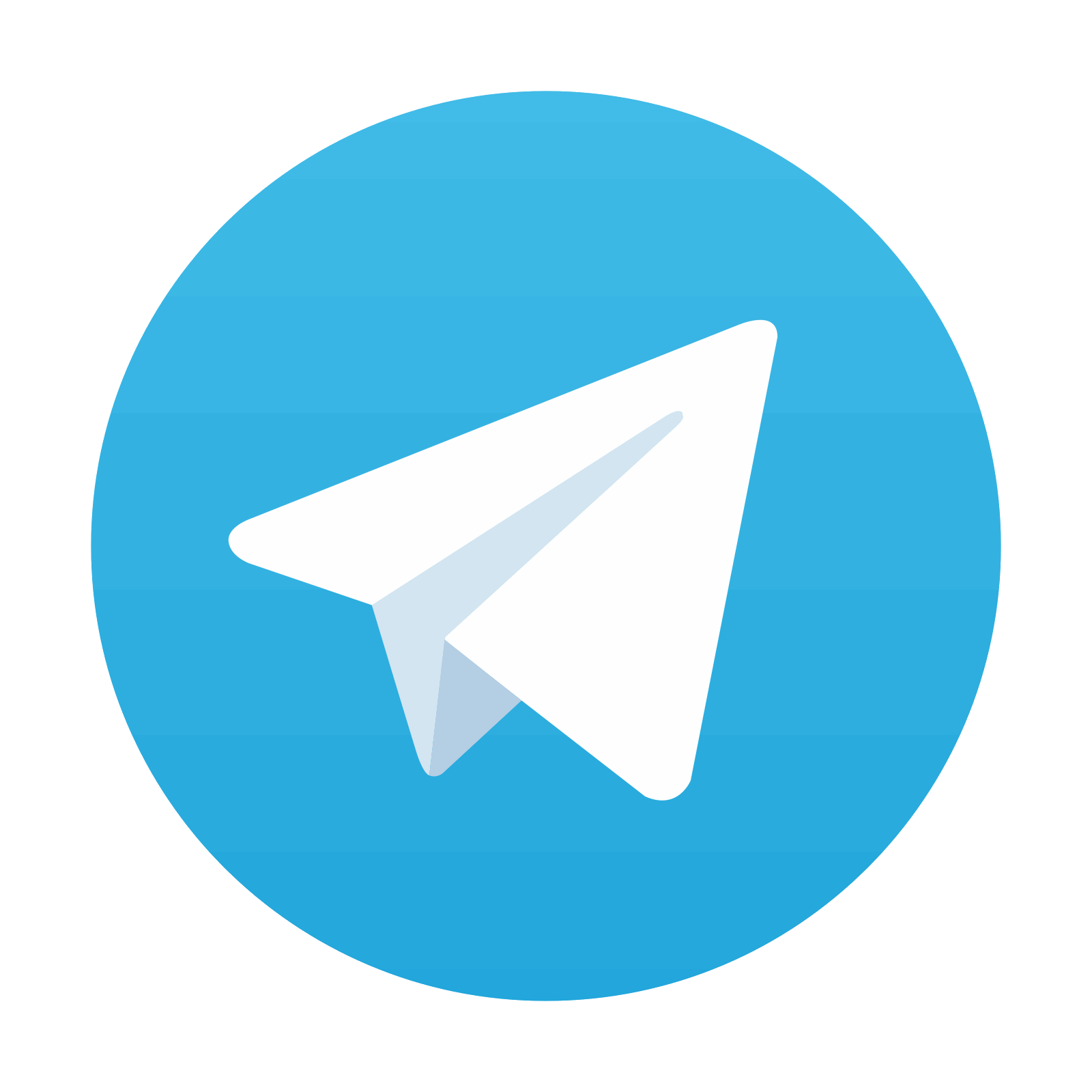
Stay updated, free articles. Join our Telegram channel

Full access? Get Clinical Tree
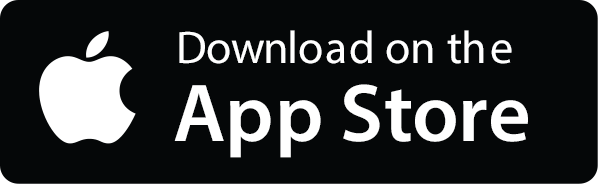
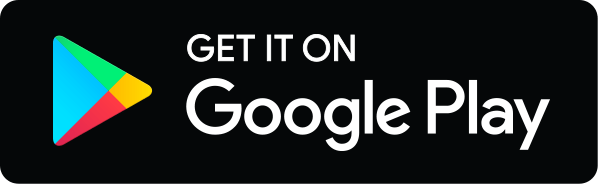