Chapter 12 Jon C. Rittenberger and Vincent N. Mosesso, Jr Although detailed algorithms and consensus guidelines exist for management of cardiac arrest, often referred to as Basic Life Support (BLS) and Advanced Cardiac Life Support (ACLS), there are unique practical and scientific considerations that may affect the execution of resuscitation efforts in the out-of-hospital setting. EMS medical directors and field personnel including EMS physicians must be aware of these factors when developing protocols for prehospital resuscitation. They must also understand the scientific basis for and the controversies surrounding recommended resuscitation actions. This chapter reviews scientific and practical considerations for carrying out BLS and ACLS in the prehospital setting. For specific treatment algorithms, the reader is referred to the American Heart Association (AHA) Emergency Cardiac Care (ECC) guidelines [1]. Chest compressions are essential in cardiac arrest resuscitation. Paradis demonstrated that only chest compressions generate coronary perfusion pressure (CPP) and that a CPP of at least 20 mmHg is important for achieving return of spontaneous circulation (ROSC) [2]. Multiple studies highlight the role of early chest compressions in survival from cardiac arrest [3–6]. The most recent BLS and ACLS guidelines emphasize the delivery of continuous chest compressions with as few interruptions as possible [1]. Kern et al. demonstrated that several consecutive chest compressions are necessary to generate adequate CPP [7]. CPP drops off immediately when chest compressions are discontinued [8]. The proportion of resuscitation time without chest compressions, termed hands-off time or no-flow fraction, is inversely associated with cardiac arrest survival [9]. Compression depth, rate, and full recoil are also critical characteristics for effectiveness. Prior work highlighted the often substandard CPR performed by prehospital and in-hospital providers. In a series of prehospital cardiac arrests in Europe, Wik et al. showed that chest compressions were delivered on average only half of the time while the patient was in arrest and that most compressions were too shallow [10]. Abella et al. found similar observations in an in-hospital series [11]. Delivering chest compressions during cardiac arrest resuscitation poses practical challenges. The treating EMS team must provide continuous chest compressions with as few interruptions as possible and must ensure high-quality chest compressions with adequate depth, rate, and recoil. To achieve these chest compression goals, additional rescuers should be dispatched to provide assistance at cardiac arrests. Team members providing chest compressions should rotate frequently, ideally every 1–2 minutes [1]. Several cardiac monitors use a compression paddle or other technology to measure the depth and rate of chest compressions [10,11]. These monitors are able to provide real-time audio and/or visual feedback, indicating to the rescuer whether or not to increase the depth or rate of compressions. Preliminary data suggest improved clinical chest compression performance through the use of feedback systems [12]. Various mechanical devices for automating chest compressions are now available. The Thumper (Michigan Instruments, Grand Rapids, MI) has been used for approximately 40 years and provides chest compressions using a pneumatic piston [13]. The Autopulse Resuscitation System (Zoll Corporation, Chelmsford, MA) facilitates chest compressions using a circumferential load-distributing band [14,15]. The Lund University Cardiopulmonary Assist Device (LUCAS) (Lund, Sweden) provides active compression and decompression through a pneumatic piston attached to a suction cup on the chest [16]. Use of mechanical compression devices is fairly widespread in Europe and is growing in the United States. The scientific data evaluating the effectiveness of these devices remain inconclusive. One urban EMS agency evaluated the Autopulse in a before/after fashion and found increases in both ROSC and survival [14]. However, a larger, multicenter, randomized controlled trial demonstrated worsened outcomes with the intervention [15]. The authors attributed this observation to potential delays in CPR required to place and activate the device. The LUCAS device was effective in a small study of 100 patients, but its benefit was only noted in those who received it within 15 minutes from the ambulance call [16]. Additional innovations and trials are sure to follow. It is important to note that each device requires time to place on the patient, during which no compressions occur. Any protocol that incorporates the use of mechanical devices must stress the importance of continuing manual compressions as much as possible until the device starts; some experts suggest withholding use of mechanical devices during the initial resuscitation effort. Defibrillation of ventricular fibrillation or ventricular tachycardia (VF/VT) is the most effective intervention for resuscitation from cardiac arrest. Ideally, automated external defibrillators (AEDs) are present at the site of the arrest for use by willing trained or untrained bystanders, perhaps at the prompting of the 9-1-1 call-taker. All medical first responders and BLS providers, including all BLS ambulances, should be equipped with AEDs. Most ALS personnel deliver shocks by manually operating a cardiac monitor-defibrillator after determining the patient has a shockable rhythm. Although many devices are configured with both hand-held paddles and cables for “hands-off” self-adhesive pads, use of the pads is believed to be safer, particularly in the uncontrolled field setting. An important technical consideration is the type of electrical waveform delivered by the defibrillator [17]. Older defibrillators use monophasic electrical current. In this mode, the device delivers electrical current in a single direction only. To compensate for increased impedance (electrical resistance), older protocols specified escalating energy levels for each successive rescue shock. Now that shocks are given one at a time, each shock should be at maximum energy output, typically 360 J. Although most current defibrillators are biphasic, some EMS systems may still use monophasic defibrillators. In a biphasic defibrillator, electrical current flows first in one direction, then in the opposite direction. This modality theoretically purges excess electrical charge from the heart. Biphasic defibrillators measure the impedance across the chest and adjust the voltage and/or duration of current appropriately. Different models also alter the pattern of delivered current, most often using a rectilinear or truncated exponential waveform. Compared with monophasic defibrillators, biphasic defibrillators demonstrate higher rescue shock success at lower energy levels and have been associated with increased rates of ROSC [18]. Energy levels for biphasic defibrillators should be based on manufacturer recommendations because each model has different waveform and delivery characteristics that affect shock efficacy. Although some manufacturers endorse non-escalating low-energy shocks (150 J), recent data suggest that higher energy biphasic shocks may increase rescue shock success without impairing cardiac function [19]. Another consideration is the interface between BLS and ALS defibrillation equipment. Some AEDs can be converted to manual mode by ALS personnel. This feature is an important logistical consideration because switching from the BLS AED to the ALS defibrillator may incur delays. Some brands use the same defibrillation pads on BLS and ALS models, allowing providers to simply unplug the connector from one device and plug into the other. The EMS physician should be aware that the AED “analyze and shock” algorithm may add 49–59 seconds of hands-off time [20]. This is an important consideration when ALS rescuers care for a patient with an AED attached. One method of minimizing hands-off time is to continue chest compressions until just before defibrillation. Recent data have suggested that continuing chest compressions during defibrillation may be feasible [21]. Others have demonstrated that standard examination gloves may be insufficient protection from electrical shock during external defibrillation [22]. Balancing safety and minimizing hands-off time remains an active area of research. A current scientific controversy is whether initial defibrillation should precede or follow an initial course of chest compressions. Prior AHA ECC algorithms specified rescue shocks first for VF, regardless of arrest duration [23]. However, several factors support performing initial chest compressions before rescue shocks, including the prolonged duration of most out-of-hospital arrests before treatment is initiated, which leads to the depletion of myocardial high-energy phosphates, cellular damage resulting from accumulated free radicals, and the development of severe acidosis [24,25]. Theoretically, a period of chest compressions may perfuse the heart and reduce the severity of these anomalies, better preparing the heart for defibrillation. However, a recent randomized clinical trial between chest compressions before rhythm analysis and immediate rhythm analysis showed no difference in the rate of good neurological outcome between groups (19.4% in early analysis versus 18.5% in chest compressions before analysis cohorts) [26]. Two clinical studies have demonstrated improved outcomes in prolonged (>4 minutes) VF arrests when chest compressions were delivered before defibrillation [27,28]. Current ACLS guidelines recommend immediate defibrillation in witnessed arrests but delivering chest compressions for about 2 minutes before rhythm analysis in unwitnessed arrests [1]. For many years airway management received emphasis in cardiac arrest care, and ALS rescuers placed high priority on endotracheal intubation (ETI) of cardiac arrest patients. However, the results of several studies question the wisdom of intubation during out-of-hospital cardiac arrest resuscitation. Some of the adverse events noted were tube misplacement, tube dislodgment, multiple laryngoscopy efforts, and failed ETI efforts [29–32]. Aufderheide et al. found that inadvertent hyperventilation often occurs after ETI, raising intrathoracic pressure and compromising CPP [33]. Perhaps most important is the frequent and often prolonged interruption of chest compressions [34]. The application of these findings to EMS practice poses important challenges. Although bag-valve-mask ventilation is theoretically adequate for resuscitation, the technique is difficult to execute in the prehospital setting, in which providers may need to deliver ventilations with the patient situated on the floor, in the back of a moving ambulance, or on a moving stretcher [35]. Most EMS agencies still perform ETI for cardiac arrest, but many try to limit the number and duration of ETI attempts [32]. Capnography should be used to verify endotracheal tube placement [36]. Although dependent on the quality of chest compressions, capnography waveforms in low-flow states are still useful for ensuring endotracheal tube position. Another emerging approach to cardiac arrest airway management is to use supraglottic airways (such as the King LT®) instead of ETI. These devices are inserted blindly into the airway without the need for direct laryngoscopy, and can typically be placed very quickly without pausing compressions (see Volume 1, Chapter 3). Several EMS agencies have chosen this approach, reasoning that they cannot perform traditional ETI without compromising chest compressions [37]. Retrospective studies have conflicting results. In a review of out-of-hospital cardiac arrests in Japan between 2005 and 2008, there was no difference in the rate of good neurological outcome in patients receiving endotracheal intubation rather than supraglottic airways (odds ratio (OR) 0.71; 95% confidence interval (CI) 0.39, 1.30) [38]. A subanalysis of the ROC PRIMED trial demonstrated a higher rate of survival with good functional status in patients receiving endotracheal intubation rather than supraglottic airway placement (OR 1.40; 95% CI 1.04, 1.89) during the resuscitation [37]. Another important consideration is the role of ventilation during chest compressions. Recent data question the need for ventilation with bystander CPR in patients with short-duration VF [7,39–43]. The theoretical bases for this approach include: Although “no ventilation” has practical value for bystander care, it is not clear how or if these principles should be applied to EMS care. There is some evidence that prearrival instruction for compression-only CPR by dispatchers results in delivery of earlier and more chest compressions, but not an increase in survival [47,48]. Some EMS systems have adopted protocols making active ventilation optional during the initial resuscitation, instead placing an oral airway and oxygen mask until sufficient rescuers are on scene. This allows the first-arriving crew to focus on compressions and defibrillation. Considerable scientific data have highlighted the importance of controlled ventilation during resuscitation. Aufderheide et al. demonstrated in cardiac arrests that hyperventilation increases intrathoracic pressure, resulting in decreased preload and CPP [33]. They also showed that inadvertent hyperventilation occurs frequently during resuscitation efforts, despite specific training to avoid this phenomenon. Ventilation during cardiac arrest should consist of tidal volumes of 500–600 mL at a respiratory rate of 8–10 breaths/min. The impedance threshold device (ITD) is a ventilation adjunct that may be attached to either a face mask or an endotracheal tube and contains a one-way valve that permits exhalation during the downstroke of chest compression but prevents passive inhalation during the upstroke of chest compression. As a result, the ITD generates increased negative intrathoracic pressure during chest recoil, increasing cardiac preload and CPP. While preclinical and small trial data were favorable, a large randomized trial of the ITD versus sham device yielded similar rates of survival with good neurological outcome between groups (ITD 5.8% versus sham device 6.0%; p=0.71) [49–54]. Although numerous medications may be used during treatment of cardiac arrest, the primary agents are vasopressors (e.g. epinephrine and vasopressin) and antiarrhythmics (e.g. lidocaine and amiodarone). ACLS algorithms provide specific guidelines for the use and doses of these agents [1]. No drug has demonstrated improved outcomes following cardiac arrest in humans [55–58]. The continued use of these drugs is based on tradition, theory, and animal research, and the selection of specific agents in each class is largely a matter of individual choice. EMS physicians must be aware that the only medications evaluated by randomized clinical trials are epinephrine, amiodarone, vasopressin, and magnesium [56,59,60]. Current ACLS guidelines downplay the use of these medications in favor of quality CPR performance [1]. Vasopressors serve two intended purposes in cardiac arrest: vasoconstriction, and exerting positive inotropy and chronotropy. Alpha-agonists increase peripheral resistance, shunting blood flow to the brain and heart. Beta-agonists increase inotropy and chronotropy. In the clinical context, these agents should help to sustain coronary and cerebral perfusion before restoration of pulses. AHA guidelines suggest administering 1 mg of IV epinephrine every 3–5 minutes and provide an option to substitute one dose with 40 units of vasopressin [1]. Compelling animal data indicate increased ROSC with the early delivery of epinephrine or vasopressin [61–64]. Although several small clinical series have reported increases in ROSC and survival to admission for patients treated with vasopressin, a randomized trial comparing vasopressin with epinephrine versus epinephrine alone did not demonstrate additional benefit from vasopressin use [59,65–67]. Two randomized controlled trials have shown no improvement in survival to discharge, but have shown increased rates of ROSC [68,69]. With the use of vasopressors, there is a trade-off between increased coronary perfusion and reduced cerebral perfusion (possibly via increased cerebral vasoconstriction). A once-popular ACLS approach was the use of high-dose epinephrine (5–7 mg IV) [55]. Although clinical trials using high-dose epinephrine demonstrated increased rates of ROSC, this did not translate into survival to discharge [55]. Antiarrhythmics are commonly used in cases of VT/VF cardiac arrest and may increase the likelihood of conversion to a perfusing rhythm. Lidocaine and amiodarone are currently recommended antiarrhythmics for shock-refractory VF; both have Class IIb recommendations (conflicting evidence) [1]. One randomized controlled trial of amiodarone demonstrated an increase in ROSC, but not improved survival to hospital discharge [60]. An important point for use in critical situations, especially with limited resources such as the out-of-hospital setting, is that amiodarone is logistically more difficult to administer than lidocaine, requiring the medication to be dispensed from glass vials. ACLS guidelines indicate that amiodarone has a stronger supporting evidence base than lidocaine, as there have been no studies evaluating lidocaine [1]. EMS physicians may choose between an IV bolus of 300 mg of amiodarone or 1–1.5 mg/kg of lidocaine for patients suffering pulseless VT/VF. A randomized, controlled trial evaluating amiodarone, lidocaine, and placebo is currently enrolling and might help to clarify this issue (ClinicalTrials.gov, identifier NCT01401647). Epidemiological studies suggest that pulseless electrical activity (PEA) and asystole are increasingly common in out-of-hospital cardiac arrests [70–73]. Atropine is a vagolytic and reverses cholinergic-mediated decreases in heart rate, blood pressure (BP), and vascular resistance [1]. Although traditionally it has been used in PEA or asystolic cardiac arrest, the limited available research does not suggest any benefit, and this drug is no longer recommended for routine use. Epinephrine should be administered, and potentially treatable causes (the 5 Hs and 5 Ts of ACLS) considered (Box 12.1). An additional drug worth comment is sodium bicarbonate. For years, sodium bicarbonate was administered routinely during cardiac arrest to reverse the metabolic acidosis of cardiac arrest, and hopefully increase the effectiveness of vasopressors and antiarrhythmics. In formal trials, this drug did not improve survival [74]. Sodium bicarbonate may be reasonable in scenarios of suspected hyperkalemic arrest (such as individuals with known renal failure) and in prolonged resuscitations with adequate ventilation. Calcium (chloride or gluconate), however, is the most effective medication in cases of severe hyperkalemia affecting cardiac conduction. Cardiac arrest represents the ultimate scenario of cardiovascular collapse. Consequently, cardiopulmonary bypass or extracorporeal life support (ECLS) may represent one potential solution. In tightly defined populations, ECLS combined with early angiography and hypothermia therapy has yielded good neurological outcomes in a significant proportion of patients [75–77]. In a study which used ECLS only after standard therapy failed, the rate of good neurological outcome was small [78]. Optimal selection of patients for this therapy will require early mobilization of resources given the association between prolonged arrest and poor neurological outcomes [79]. Cardiac arrest care interventions are time-critical. Thus, protocols should allow EMS personnel to initiate resuscitation immediately. Non-physician providers should provide initial cardiac arrest care using standing orders, as there is inadequate time to consult with the direct medical oversight physician for detailed guidance. The protocols should detail interventions for the various ECG rhythms likely to be encountered: VF, VT, PEA, and asystole. Protocols should provide convenient reference to medication dosages, mixtures, and administration rates. Other practical information should also be included, such as criteria for termination of resuscitation. Many systems use current AHA ACLS algorithms as the basis for cardiac arrest protocols [1].
Cardiac arrest: clinical management
Introduction
Specific interventions
Chest compressions
Defibrillation
Airway management
Ventilation
Medications
Additional therapies
Principles of management
Resuscitation protocols
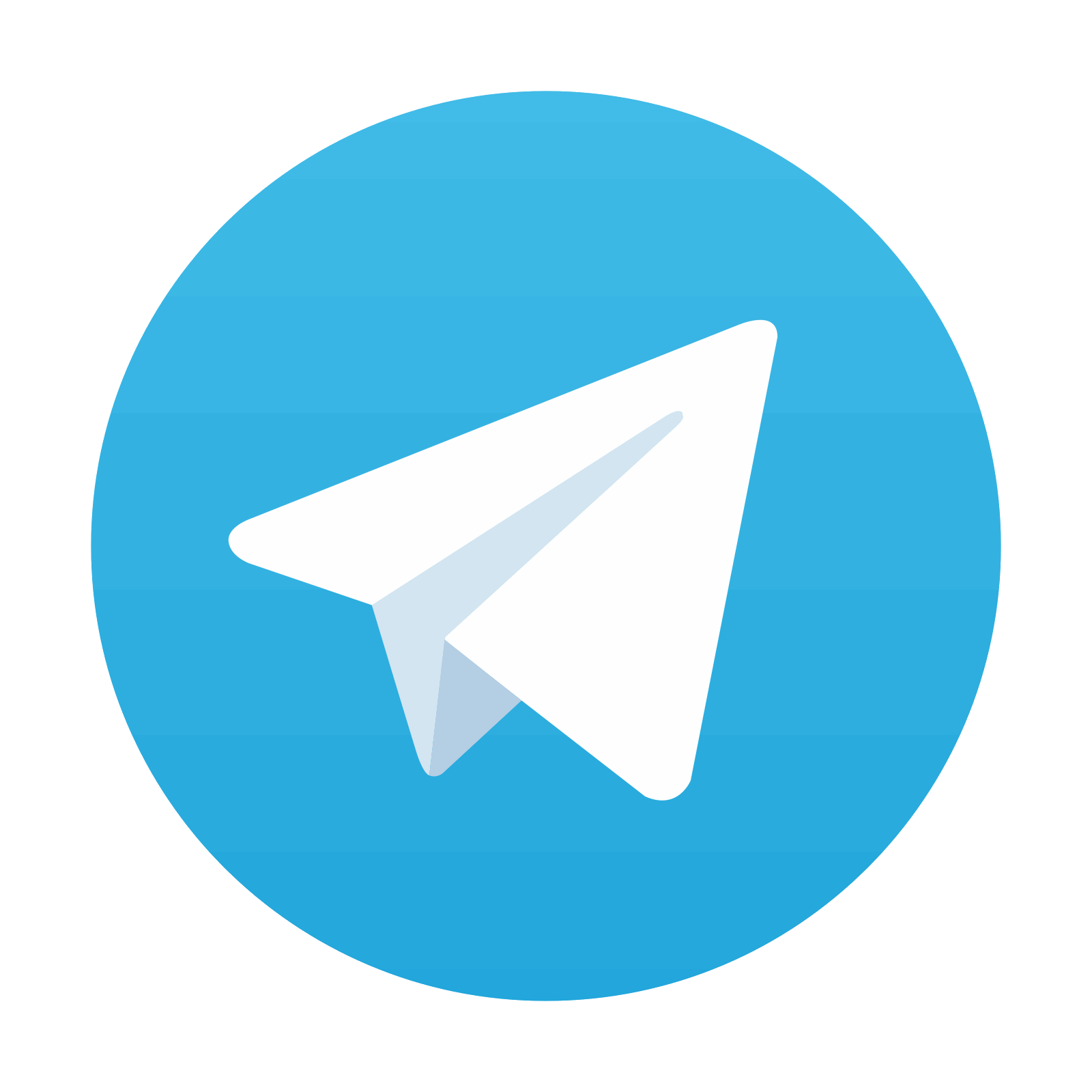
Stay updated, free articles. Join our Telegram channel

Full access? Get Clinical Tree
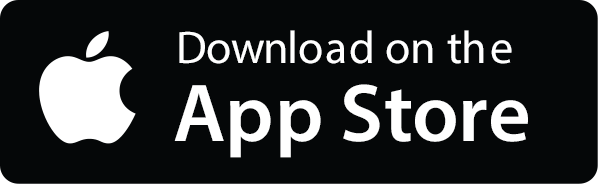
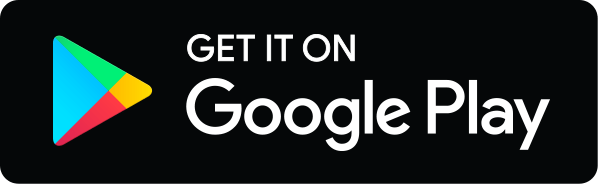