Abstract
The regulation of small blood vessels (arterioles, venules and capillaries) and the formation of interstitial fluid at the capillary beds is an important process in the understanding of the healthy circulation. Traditionally, Starling’s forces have been at the very heart of our understanding of this system. However, more recent work shows that the transvascular fluid flux is significantly lower than what one would expect on the basis of Starling’s forces alone, and hence alternative explanations have been sought to understand the process of tissue fluid formation. In this context the role of the endothelial glycocalyx layer (or EGL) has drawn substantial interest. The EGL is a dynamic, active interface between the blood and the endothelial cells. It is formed by membrane-bound glycoproteins, proteoglycans and polysaccharides, producing a hydrated gel-like layer on the luminal surface of the vascular endothelium of approximately 500–2000 nm thickness. In this paper we review some of these emerging concepts and propose alternative ideas to understand some frequent clinical conditions and their treatment.
After reading this article, you should be able to:
- •
provide an abbreviated and abridged review of Starling’s principles
- •
describe the structural differences between arterioles, venules and capillaries
- •
describe the functional anatomy of the ‘interstitium’ or the ‘interstitial compartment’ and elaborate how the different components may behave in health and disease
- •
describe the endothelial glycocalyx layer (EGL) and the separation of the intravascular volume into the effective circulating volume and the subglycocalx space (SGS)
- •
elaborate the possible role of the endothelial glycocalyx layer (EGL) in sepsis and oedema formation
- •
elaborate the potential consequences of albumin therapy when the EGL is damaged or disrupted in disease
The microcirculation was originally described by Malpighi in 1661, who with the aid of a single lens microscope was the first person to describe the functional capillary unit as we now know it. It represents a complex interface between the macrocirculation and individual cellular structures. It is a highly integrated, functional system, maintaining the local milieu and thus facilitating essential cellular function. Evolutionarily it has developed as organisms have evolved into multicellular structures and diffusion alone was ineffective in supplying the essential needs of tissues. For example, based on Fick’s principles at a distance of 0.1 micrometres (the distance between the erythrocyte and the capillary endothelial cell) diffusion of an oxygen (O 2 ) molecule would take 2 microseconds but when extended to 1 cm the time taken for diffusion becomes several hours. Our understanding of the microcirculation has come some way since Harvey in 1628 postulated the existence of invisible ‘ pores to the flesh ’. Much of our understanding of the functions of the microcirculation as a concept derives from Starling’s original work. The aim of this article is to provide an abridged review of Starling’s principles while exploring new concepts that build upon these original constructs.
Structure of the microcirculation
The term microcirculation includes terminal arterioles, capillary beds and venules. In addition, we include the surrounding interstitial fluid (ISF), lymphatic channels and collecting ducts ( Figure 1 ). In reality, it is a complex, dynamic system comprised of up to 10 billion capillary beds, continually fluctuating between their perfused and collapsed state under the influence of systemic and local factors. Often for simplicity the description is of the average net perfusion of these units, although inherently these units are independent and fluctuate rapidly.

Arteriole
The arterioles are well-innervated vessels, encircled by smooth muscle, meaning their diameter can change many-fold in response to autonomic nervous inputs. Arterioles branch multiple times before reaching the diameter of 5–9 micrometres where they supply blood to the capillaries under the control of the pre-capillary sphincter. The arterioles terminate in the met-arteriole, differentiated by a non-continuous encircling of smooth muscle. This too provides direct branches to the capillary bed, under the control of the pre-capillary sphincter. Direct vessels may branch off the arteriole side without an associated pre-capillary sphincter and can be defined as an arteriovenous bypass.
Venule
Venules are larger and have a less established smooth muscle coat. Flow through the venule system is of much lower pressure, and as such despite the poor formation of the muscular layer, it is still able to generate significant changes in flow in response to changes in autonomic nervous tone.
Capillary
Capillary walls are formed by a single layer of endothelial cells surrounded by a basement membrane. They are devoid of surrounding intima or smooth muscle, having a wall thickness of 0.5 micrometres. The vessel diameter of 5–9 micrometres is just large enough to facilitate the passage of erythrocytes, although their flow through these vessels is often dependent on their inherent deformability. In pathological states, this deformability may be impaired, resulting in the obstruction to flow through the capillary bed. Such abnormal or poor capillary flow may also be observed when the viscosity of blood is increased in states of dehydration. The permeability of these capillaries is highly tissue specific, and largely dependent on the presence and size of the inter-cellular clefts. These clefts are formed at the junctions of adjacent endothelial cells, and are approximately 6–7 nm in diameter, slightly smaller than the diameter of an albumin protein molecule. These clefts theoretically produce no resistance to the passage of water and most water-soluble substances. Variations on these pores occur in specific tissues. For example within the brain the endothelial cells are connected by ‘tight junctions’, which regulate the passage of constituents between luminal and extraluminal spaces, selectively facilitating the free passage of small elements such as water, carbon dioxide (CO 2 ) and O 2 only. Conversely, in the liver the wider intracellular clefts allow almost uninterrupted passage of substances, facilitating its essential metabolic and immunological functions. All other tissues lie somewhere within this spectrum, largely individualized for their specific functions.
Interstitium and interstitial fluid (ISF)
This space is often perceived as a passive reservoir, holding all filtrated fluid from the microcirculation before returning it via the lymphatic system to the general circulation. However, this is structurally complex space contains two major structural components, together forming the extracellular matrix . Key components of the matrix include ( Figure 2 ):
- •
Collagen fibre bundles – provide tensile strength and structural support.
- •
Proteoglycan filaments – small filaments, composed almost universally of hyaluronic acid (98%) and protein. They form a ‘brush’ of fine reticular filaments, trapping water to prevent movement by mass flux.
- •
Free water comprises approximately 1% of ISF in health, although there is a significant increase in this component (often >50%) in the development of tissue oedema.
Lymphatic system
It is essential for returning filtrate from the interstitium back to the intravascular compartment. This ultimately occurs at the thoracic duct at the junction of the left internal jugular vein (IJV) and subclavian veins (SCV), or the right lymphatic duct which drains at the junction of right IJV and SCV. Total daily lymphatic flow is approximately 2–3 litres/day, the majority of which is derived from the enteric circulation. In addition to ultrafiltrate, lymphatics also carry fats, with an observed large surge in the fat content following consumption of meals. Lymphatic flow has inherent reserve, and can increase almost 20-fold in response to increased interstitial volume and pressure. An increase in interstitial pressure (P i ) will increase centripetal lymphatic flow, while reducing ultrafiltrate production by opposing transcapillary flux (J v ). Thus transcapillary pressures in health will oscillate around an equilibrium. However, under extreme circumstances, as often seen during significant microcirculatory dysfunction, there is a significant increase in P i , to values of 1–2 mmHg above atmospheric pressure. This results in direct external compression of lymphatic vessels, impeding lymph flow. Ultimately this results in a plateauing of lymph flow with increasing J v ( Figure 3 ). This uncoupling of J v and lymphatic flow results in further interstitial oedema, and its subsequent detrimental effects.


The presence of valves ensures flow remains unidirectional and this constant flow ensures the maintenance of relative negative interstitial pressures. Flow through the lymphatic system is augmented by two discrete mechanisms:
- •
Inherent contractility – sequential segmental contraction in response to stretching occurs throughout the system.
- •
External intermittent compression – caused by movement, skeletal muscle contraction and pulsatility of nearby arterial vessels. In exercise this can increase lymphatic flow by 20- to 30-fold, whereas in the sedated patient this may be markedly decreased.
Total lymphatic flow is dependent on relative hydrostatic and oncotic pressures, capillary permeability and lymphatic drainage. This can be summarized utilizing Starling’s theorem of transcapillary haemodynamics.
Starling’s theorem
Transcapillaryflow(Jv)=(Pc−Pi)−(Πc−Πi)
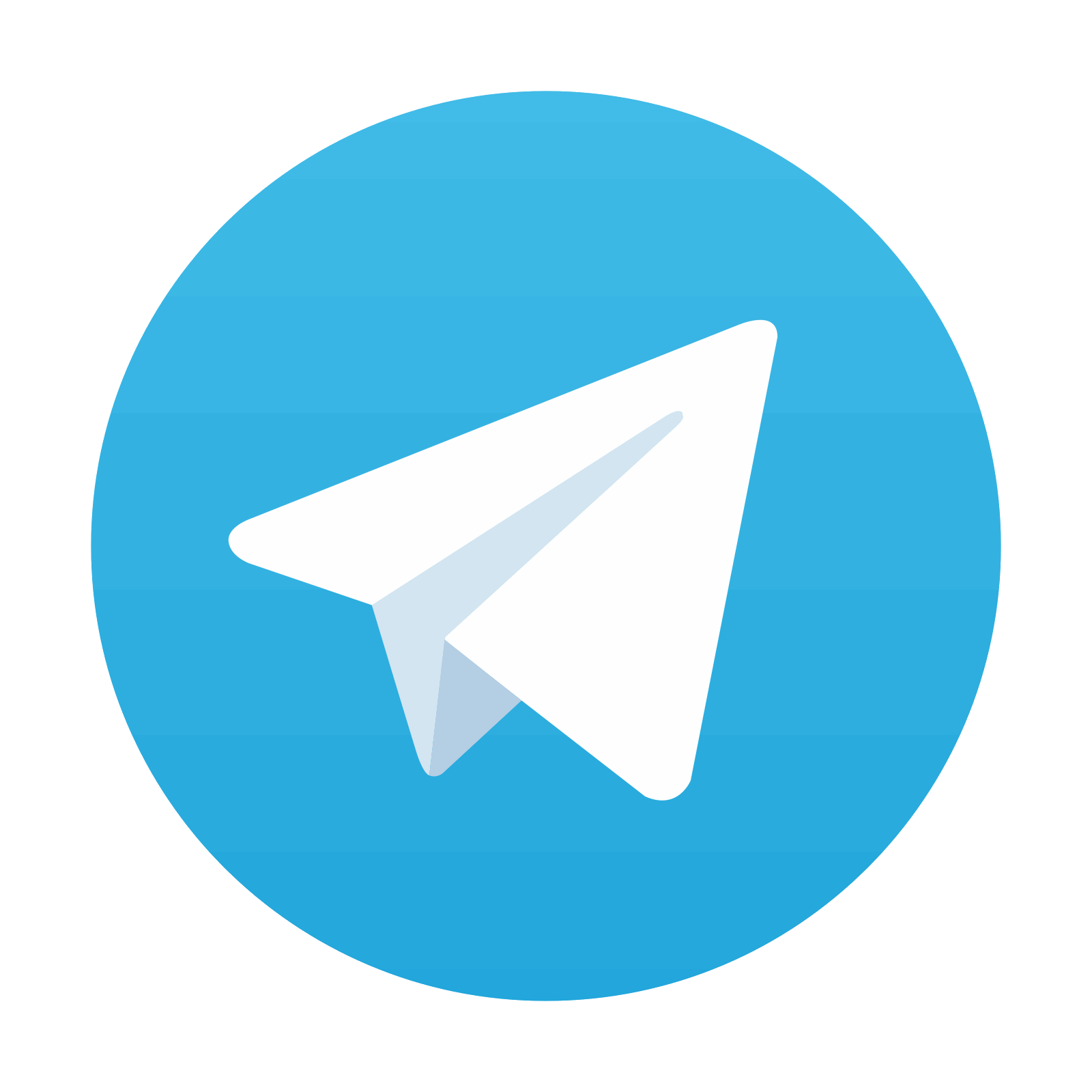
Stay updated, free articles. Join our Telegram channel

Full access? Get Clinical Tree
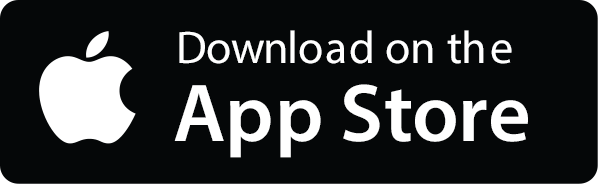
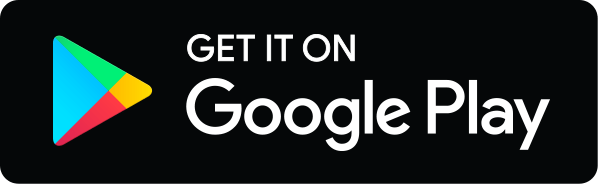
