Key points
- ▪
The morbidity and mortality associated with burns vary with total area burned, depth of burn, presence of inhalation injury, and coexisting diseases.
- ▪
Adequate fluid resuscitation, appropriate analgesia, and early excision of wounds are vital to improve the outcome in burn patients.
- ▪
Understanding the pathophysiologic changes associated with major thermal injury, particularly the hypermetabolic response, is essential for perioperative care of burn patients.
- ▪
Surgical burn patients are susceptible to hypothermia due to evaporative heat loss from exposed skin burn surface and donor areas.
- ▪
Topical epinephrine, tourniquets, and a staged procedure reduce blood loss during tangential burn excision.
- ▪
Burn patients rapidly become tolerant to opiates. Perioperative analgesic needs can be high and are often underestimated.
- ▪
Adjuncts to opiate analgesia include acetaminophen, ketamine, nerve blocks, and tumescent analgesia.
- ▪
A hyperkalemic response to succinylcholine can occur 2 days to 6 months after thermal injury from proliferation of acetylcholine receptors. During this period, patients become resistant to nondepolarizing neuromuscular blockers.
Perioperative management of patients with severe burn injuries presents significant challenges to the anesthesiologist. An estimated 1.25 million patients with burn injuries are treated each year in the United States, up to 100,000 of whom require hospitalization. More than 6500 patients succumb to their thermal injuries. A better understanding of the pathophysiology of burn injuries, coupled with advances in burn resuscitation, critical care, and surgical practice, has resulted in improved survival in severely burned patients over the past three decades. In 2001 the American Burn Association (ABA) developed evidence-based guidelines for the management of acute burn injury.
Modern care for the severely burned patient can be divided into four overlapping phases: (1) initial evaluation and resuscitation, (2) initial excision and biologic closure, (3) definitive wound closure, and (4) rehabilitation and reconstruction. The anesthesiologist’s services may be called on for airway management, intravenous access, and fluid resuscitation, in addition to providing sedation and analgesia in the acute phase. Administration of analgesia and sedation for wound care and provision of anesthesia for excision and grafting are even more challenging tasks. Reconstructive surgery poses special challenges because of the development of contractures, making airway management and positioning difficult.
Pathophysiology
The primary determinants of severity of burn injury are the size and depth of the burn. However, patient age, body part burned, presence of pre-existing disease, and associated nonburn injuries have an important impact on the outcome. The size of the burn is usually estimated in adults by using the “rule of nines” and expressed as percentage of total body surface area (TBSA) ( Fig. 18-1 ). The burn depth is classified as superficial, partial thickness, and full thickness ( Table 18-1 ). First-degree (superficial) burns affect only the epidermis and are characterized by erythema and edema of the burned areas without blistering or desquamation. Superficial burns are treated with daily dressing and wound care until epithelialization occurs. Second-degree (partial-thickness) burns involve the epidermis and a portion of the dermis. In most cases, partial-thickness wounds can be expected to heal spontaneously in 1 to 4 weeks, although surgical treatment may be necessary for extensive or deep second-degree burns. Pain is characteristic of partial-thickness burns. Third-degree (full-thickness) burns extend entirely through both the epidermis and dermis and will not heal spontaneously.

Classification | Burn Depth | Outcome |
---|---|---|
Superficial (first degree) | Epidermis only | Heal spontaneously |
Partial thickness (second degree) | Epidermis and dermis | |
Full thickness | ||
Third degree | Destruction of epidermis and dermis | Wound excision and grafting necessary |
Fourth degree | Fascia, muscle, bone burned | Complete excision required; functional limitation likely |
Severe burn injury results in release of circulating mediators that evoke a physiologic response, the systemic inflammatory response syndrome (SIRS), throughout the body ( Fig. 18-2 ). These mediators include histamine, serotonin, cytokines, tumor necrosis factor alpha (TNF-α), endotoxin, oxygen-derived free radicals, nitric oxide, and complement.

Cardiovascular Effects
There is an increase in capillary permeability and “third spacing” of fluid in tissues surrounding the burn. Interstitial edema and organ dysfunction in distant organs result from combination of the vasoactive mediators and hypoproteinemia in severe burns. Increased capillary permeability is seen in the burned tissue for more than 72 hours and in the nonburned tissue for up to 24 hours. TNF-α, O 2 -derived free radicals, and endothelin-1 exert a negative inotropic effect and reduce cardiac output acutely. The cardiovascular response to both endogenous and exogenous catecholamines is attenuated because of decreased adrenergic receptor affinity and decreased production of second messengers. Systemic vascular resistance (SVR) increases in the initial postburn period. Later, in the hypermetabolic phase, cardiac output is increased and SVR is reduced.
Metabolic Changes
Circulating levels of catecholamines are increased up to 10-fold after severe burn injury. Along with wound-released mediators, hormones, and bacterial products from the gut and wound, this results in SIRS, manifested as hyperdynamic circulation and large increases in basal energy expenditure (hypermetabolic response). Glucagon and cortisol secretion increases with postinjury insulin resistance, resulting in use of amino acids to fuel production, leading to muscle wasting and nitrogen imbalance. The supraphysiologic thermogenesis is associated with resetting of the core temperature to higher levels, proportional to the size of the burned areas. Damaged skin is no longer able to retain heat and water, and the vasomotor thermoregulatory responses are impaired; large evaporative losses ensue. Loss of barrier function of skin and blunting of immune response increase susceptibility to infection and bacterial overgrowth within the eschar. Adequate pain control, alleviation of anxiety, maintenance of a thermoneutral environment, and treatment of infection are important steps in limiting catecholamine secretion and thus hypermetabolism.
Hematologic Effects
Hematologic and coagulation factor changes after burn injury depend on the magnitude of burn injury and time from injury. Hematocrit is typically maintained early in the postburn period but drops during the weeks of care as erythrocyte half-life is reduced. Platelet count diminishes with formation of microaggregates in the skin and smoke-damaged lung, although this is rarely a clinical problem. Both thrombotic and fibrinolytic mechanisms are activated after major burns. Clinically, hypercoagulability may be a problem in late postburn injury period, and patients should receive thromboembolism prophylaxis.
Renal Function
The incidence of acute renal failure in burn patients ranges from 0.5% to 38%, depending on the severity of burns. In the early postburn period, renal blood flow is reduced as a result of hypovolemia and decreased cardiac output. In addition, increased levels of catecholamines, angiotensin, vasopressin, and aldosterone contribute to renal vasoconstriction. Myoglobinuria and sepsis can also aggravate renal dysfunction. Despite an increase in the renal blood flow during the hypermetabolic phase of burn injury, tubular function and creatinine clearance may be reduced and renal function variable.
Pharmacologic Effects
Burn injury also affects the pharmacodynamic and pharmacokinetic properties of many drugs. A decreased level of serum albumin in these patients leads to increased free fraction of acidic drugs such as thiopental or diazepam, whereas an increased level of α-acid glycoprotein results in decreased free fraction of basic drugs (with pK a > 8) such as lidocaine or propranolol. Renal and hepatic function may be impaired in patients with large burns, in turn impairing the elimination of some drugs, whereas increases in renal blood flow and glomerular filtration rate in the hyperdynamic phase of burns may enhance renal drug excretion. Some drugs, such as gentamicin, may be lost through the open wounds. The response to muscle relaxants (other than mivacurium) is altered because of proliferation of acetylcholine receptors away from the synaptic cleft of the neuromuscular junction (see later discussion). Pharmacokinetics of morphine are unchanged after burn injury. Although lorazepam has an increased volume of distribution, increased clearance, and a reduced half-life, the elimination half-life of diazepam is significantly prolonged in burn patients.
Inhalation Injury
Most airway inhalation injuries are caused by inhalation of smoke. A history of closed-space exposure to hot gases, steam, or smoke; singed nasal vibrissae; carbonaceous sputum; and elevated levels of carboxyhemoglobin or cyanide suggest the clinical diagnosis. Inhalation injury is a predictor of increased morbidity and mortality in burn victims.
Upper airway injury
Direct thermal injury to the subglottic airway is rare, unless superheated air or steam is inhaled. The severity of inhalation injury depends on the fuels burned, intensity of combustion, duration of exposure, and confinement. Unless steam is involved, heat injury to the airway is supraglottic, causing swelling of the posterior pharynx and supraglottic regions, leading to potential upper airway obstruction. The natural history of upper airway inhalation injury is edema formation that narrows the airway over the initial 12 to 48 hours. Early tracheal intubation is recommended in patients who present with stridor, wheeze, or voice changes. Burns to the face and neck can result in tight eschar formation, making airway management difficult when combined with pharyngeal edema.
Lower airway injury (smoke inhalation injury)
Lower airway or pulmonary parenchymal damage results from inhalation of the chemical constituents of smoke, usually becoming apparent 24 to 72 hours after the injury. Findings include dyspnea, rales, rhonchi, and wheezing. Gas-phase constituents of smoke include carbon monoxide (CO), cyanide, hydrochloric acid, aldehyde gases, and oxidants. These gases can cause direct damage to mucociliary function and bronchial vessel permeability, as well as produce bronchospasm, alveolar destruction, and pulmonary edema. Small airway occlusion results from endobronchial sloughing and resultant debris, whereas alveolar, interstitial, and chest wall edema may cause intrapulmonary shunting and reduction in compliance. The risk of pulmonary infection and barotrauma is also increased. The clinical picture is identical to that of acute respiratory distress syndrome (ARDS). Delayed ARDS (6-10 days after burn) may also develop in the absence of inhalation injury in burn victims. Bronchoscopy reveals carbonaceous endobronchial debris and/or mucosal ulceration. The usefulness of serial chest radiographs or radioisotope scanning with xenon or technetium for diagnosis and predicting prognosis is questionable.
Meticulous pulmonary toilet is the cornerstone of early care. Tracheal secretions are often extremely viscous and may contain carbonaceous particles and pieces of mucous membrane.
Carbon Monoxide and Cyanide Poisoning
Carbon monoxide has a high affinity for hemoglobin (250 times more than for O 2 ) and can interfere with O 2 delivery to the tissues at higher concentrations. Administration of 100% O 2 reduces the half-life of carboxyhemoglobin from 2 <SPAN role=presentation tabIndex=0 id=MathJax-Element-1-Frame class=MathJax style="POSITION: relative" data-mathml='12′>1212
1 2
hours to 40 minutes and facilitates the elimination of CO. Hyperbaric oxygen therapy has limited indications because of the logistical challenges presented by transport of patients with concomitant burns to such chambers. Cyanide causes tissue hypoxia by uncoupling oxidative phosphorylation in mitochondria. Treatment with sodium nitrite, sodium thiosulfate, hydroxocobalamin, or dicobalt edetate should be considered for cyanide poisoning in patients with unexplained severe metabolic acidosis associated with elevated central venous O 2 (therefore patients are clinically not cyanotic), normal arterial O 2 content, and low carboxyhemoglobin.
Signs such as hyperthermia, tachycardia, leukocytosis, and tachypnea cannot be used to diagnose sepsis in burn victims. Other identifiers, such as thrombocytopenia, enteral feeding intolerance, and hyperglycemia, have been used instead.
Preoperative preparation
The preoperative evaluation of burn patients should take into account the continuum of pathophysiologic changes caused by burns. Patient age, percentage of TBSA burned, depth of burns, time after injury, sites and extent of planned excision and donor areas, presence of infection, other injuries (especially inhalation injury), and the presence and extent of comorbidities should all be assessed.
Careful airway assessment uses the standard bedside tests. Mallampati class; thyromental distance; head, neck, and jaw mobility; presence of facial or airway burns (or edema); and contractures of face and neck are used to plan the perioperative airway management technique. The patient at risk for airway complications should have a difficult airway cart available containing various-sized endotracheal tubes, Eschmann stylet, laryngeal mask airways (LMAs), Fastrach LMA, fiberoptic bronchoscope, and fiberoptic stylets.
Fluid Resuscitation
The widely quoted Baxter (Parkland) formula for initial fluid resuscitation of burn victims is 4 mL of Ringer’s lactate per kilogram of body weight per %TBSA burned, one half to be given during the first 8 hours after injury and the rest in the next 16 hours. Hypertonic saline may be useful in early shock, and colloids are most effective when used in the 12- to 24-hour period of resuscitation. It is widely believed that the Parkland formula underestimates resuscitation volumes, particularly when concomitant smoke inhalation is present. Repeated bedside observations and clinical evaluations are useful to judge the adequacy of resuscitation. Normal mentation, stable vital signs, and urine output of 30 to 50 mL/hr can be used as end points, whereas use of core-periphery temperature gradient may be unreliable. However, several studies have shown advantages to invasive hemodynamic monitoring (with pulmonary artery catheter) in adults with serious burns who do not respond as expected to fluid resuscitation. Serial lactate levels, monitoring the base deficit, and optimization of intrathoracic blood volume (ITBV) are also useful guides to successful resuscitation ( Box 18-1 ).
What was the mechanism of injury for the burns? Body surface area burned? Depth of burns?
Was closed-space confinement involved?
Did patient have black sputum?
What is elapsed time since injury?
Has adequacy of resuscitation been maintained?
What is the extent of the planned excision? Location of burn areas to be excised and donor areas?
Has surgical position been determined? Need for intraoperative change of position?
Are pain scores available? What is the patient’s 24-hour analgesic requirement?
Any associated injuries?
Any coexisting diseases?
Fasting Requirements
Metabolic complications in burn patients are directly related to extent of burn. Thermal injury leads to hypermetabolism and protein hypercatabolic state. Early postpyloric enteral feeding, which can be continued in the perioperative period, is recommended by the ABA evidence-based guidelines. Early institution of enteral feeding in these patients decreases infections and sepsis, improves wound healing and nitrogen balance, and reduces stress ulceration and duration of hospitalization. Gastric emptying may not be delayed in burn patients, and gastric acid production may actually be reduced in the early postburn period. The safety and advantages of perioperative enteral feedings have been reported. At the authors’ institution, enteral feedings are continued throughout the perioperative period in patients who come to the operating room intubated. In nonintubated patients, shorter fasting times, typically 2 to 4 hours, may be acceptable.
Surgical considerations
Burn patients could present for five types of surgical procedures: (1) decompression procedures such as escharotomy or laparotomy, (2) excision and biologic closure of burn wounds, (3) definitive closure procedures, (4) burn reconstructive procedures, and (5) general supportive procedures such as gastrostomy or line placement.
Excision and Grafting
The need and timing for surgery is determined primarily by the size of the burn injury. The objective is to identify, excise, and achieve biologic closure of all full-thickness burns. The advantages of early excision and grafting (1-5 days after burn injury) include reduction in incidence of septic episodes, reduced hospital stay, and increased survival rates. Extensive burns may need staged excision to limit the physiologic insult of one massive surgery and to allow autologous skin grafts to be available. Excision and grafting involves tangential excision of the second-degree burn wound, in which the eschar is shaved off from the burn until a plane of viable tissue is reached, followed by covering the excised wound with a split-thickness skin graft, allogeneic skin from cadavers, or skin substitutes (e.g., Integra). Excision of third-degree burns requires fascial excision, in which the overlying burned skin and subcutaneous fat are excised down to muscle fascia. Box 18-2 summarizes the required equipment in preparation for excision and grafting.
“Difficult airway” cart; umbilical tape, dental floss, wire for suturing tube
Operating room warmed to 28° to 32 ° C; fluid warmer, radiant heat warmer
Availability of blood products
Adequate intravenous access; consider invasive monitoring
Anesthetic considerations
General anesthesia, with the combination of an opioid, muscle relaxant, and a volatile agent, is the most widely used technique for burn excision and grafting. Succinylcholine administration to patients more than 24 hours after burn injury is unsafe because of the risk of hyperkalemic ventricular dysrhythmias. The time frame during which succinylcholine must be avoided after a burn begins 48 hours after the event. Patients who have been bedridden because of severity of illness or concomitant disease or injury, or those receiving prolonged muscle relaxant therapy to facilitate mechanical ventilation, may be particularly vulnerable. The exact period of risk is unknown, although 6 months can be considered the absolute minimum ; acetylcholine receptors proliferate and spread throughout the skeletal muscle membrane under the burn and at sites distant from the burn injury. The upregulation of acetylcholine receptors, along with altered protein binding, especially to α 1 -glycoprotein, makes patients with thermal injury resistant to the action of nondepolarizing muscle relaxants. In these patients, larger doses of nondepolarizing muscle relaxants may be required to achieve a given degree of neuromuscular blockade; onset of paralysis may take longer, and duration of paralysis may be shorter. The resistance is usually seen in patients with greater than 30% TBSA burns; it develops after the first week following injury and peaks at 5 to 6 weeks. Mivacurium may be immune to this resistance, possibly because of its decreased metabolism from depressed pseudocholinesterase activity in burn patients.
Airway Management
Airway management in burn patients can be challenging. Mask ventilation may be a problem with facial burns. Successful use of an LMA for burn surgery has been reported. However, major procedures in critically ill patients, with frequent intraoperative changes in patient position, are best done with endotracheal intubation. Awake fiberoptic intubation may be indicated if difficulties with intubation and ventilation are identified preoperatively. Inhalation induction, maintenance of spontaneous respirations, and intubation with fiberoptic guidance or Fastrach LMA may be advocated in uncooperative patients.
Location of burns and donor skin sites indicate the need for special positioning, for repositioning the patient during operation, or both. Fixing the endotracheal tube (ETT) for prone positioning in the presence of facial burns is best achieved by wiring it to the teeth or stitching it to the nares. We typically use dental floss to tie the tube to the teeth or tie the tube to an oronasal loop of rubber catheter. A combination of prolonged prone positioning and relatively high fluid volume administration may cause significant airway swelling. It is best to wait until an air leak is present around the ETT before tracheal extubation, because this indicates resolution of edema, especially in older children. If there is still no air leak and the patient is deemed ready for tracheal extubation, direct laryngoscopy may be necessary to determine the extent of residual edema. Once extubated, the patient should be closely monitored for progressive airway obstruction during the subsequent 24 to 48 hours.
Edema, scarring, or contractures may narrow the mouth opening and limit the neck movements, depending on the age of the burns. Surgical release of neck contractures to facilitate intubation has been described in both elective and emergency settings.
Analgesia
Severe pain is an inevitable consequence of a major burn injury, and perioperative analgesic requirements are frequently underestimated. Anxiety and depression are common components in a major burn and can further decrease the pain threshold. Perioperative pain management should be based on an understanding of the types of burn pain (acute or procedure-related pain vs. background or baseline pain), frequent patient assessment by an acute pain service team, and the development of protocols to address problems such as breakthrough pain.
High-dose opioids are needed to manage pain associated with burn procedures, and morphine is currently the most widely used drug. The pharmacokinetics of morphine are similar in burn patients and control subjects. Providing adequate analgesia with morphine reduces the risk of posttraumatic stress syndrome. Most burn patients rapidly develop tolerance to opioids. With individual variation in response to morphine, “titration to effect” and frequent reassessment are especially important.
Fentanyl is also a useful analgesic perioperatively. Continuous infusion of fentanyl in the preoperative period may induce a rapid tolerance in burn patients.
Methadone has the advantage of N -methyl- d -aspartate (NMDA) receptor antagonist activity, which helps in preventing the development of central sensitization, secondary hyperalgesia, and neuropathic pain. In addition, the long duration of action helps in achieving postoperative analgesia and it can be administered orally in the postoperative period.
Nonsteroidal anti-inflammatory drugs (NSAIDs) reduce pain perception and modify the systemic inflammatory response through inhibition of cyclo-oxygenase. The incidence of gastric ulceration, increased operative blood loss, and exacerbation of asthma is reduced with the use of selective COX-2 inhibitors. However, potential for renal tubular dysfunction does exist. These drugs have not yet been systematically evaluated in burn patients.
Acetaminophen is a useful adjunctive analgesic in combination with opioids. Its antipyretic action is particularly useful in burn patients. Doses of 15 mg/kg can be given orally or rectally every 6 hours to a maximum of 4 g/day. Liver function tests and acetaminophen levels should be checked weekly in patients receiving long-term therapy.
Tumescent local anesthesia with a maximal dose of 7 mg/kg of lidocaine has been shown to be safe and the sole potentially effective locoregional anesthetic technique for the surgical treatment of pediatric burns. Postoperative pain from split-skin donor sites is often more intense than the pain at the grafted site. Addition of bupivacaine or lidocaine to the “Pitkin solution” (subcutaneous crystalloid injection) can provide analgesia for pain originating from the donor areas. A continuous fascia iliaca compartment block can also be used to reduce the pain at the thigh donor site. Intravenous lidocaine (1 mg/kg) has provided significant postoperative analgesia for up to 3 days.
Ventilation
Mechanical ventilation is necessary for patients with respiratory complications, inhalation injury, or large burns. Hypermetabolic state after burn injury increases the carbon dioxide production, and these patients need higher minute ventilation to maintain normocapnia. In patients with acute lung injury who need high levels of positive end-expiratory pressure (PEEP > 15 cm H 2 O) or peak inspiratory pressure (PIP > 50 cm H 2 O) to maintain gas exchange, use of sophisticated intensive care ventilator and anesthesia maintenance using total intravenous anesthesia technique (TIVA) may be warranted.
Regional Anesthesia
Regional anesthesia alone or in combination with general anesthesia can be used in patients with small burns or for reconstructive procedures. For procedures on lower extremities, lumbar epidural or caudal catheters can be used to provide intraoperative and postoperative analgesia. The greatest limitation to the use of regional techniques is the extent of surgical field; most patients with major burns have a wide distribution of injuries and need skin harvesting from areas too large to be blocked by a regional technique. The presence of a coagulopathy or systemic or local infection may also contraindicate regional anesthetic techniques in these patients.
Monitoring
Monitoring for burn surgery should be based on knowledge of the patient’s medical condition and the extent of surgery. Standard electrocardiographic electrodes may not adhere to burned surfaces. Needle electrodes or alligator clips attached to skin staples may be effective alternatives. If skin sites for pulse oximetry monitoring are limited, the ear, nose, tongue, or penis can be used with standard probes. The alternative is to use reflectance pulse oximetry.
Arterial line placement allows repeated blood sampling for estimation of arterial blood gas tensions, hematocrit, electrolytes, lactate, and coagulation profiles, in addition to continuous blood pressure monitoring. The decision to use invasive monitoring such as a central venous or pulmonary artery catheter should be based on coexisting medical conditions or burn-related complications. Core temperature (bladder or esophageal), urine output, and degree of neuromuscular blockade should be routinely monitored.
Hypothermia is a common complication of excision and grafting and often delays extubation. Body temperature is best maintained by a thermoneutral environment (room temperature of 28°-32° C) with the additional use of an over-bed warming shield and warming of intravenous fluids. Dry-air warmers used directly over the burn wound can cause tissue desiccation. Forced-air warming devices are less effective in these patients because of the significant area of burned and donor skin sites that must remain exposed. Use of “space blankets” (aluminum foil coverings on nonexposed areas), plastic sheets over the head and face, heat and moisture exchangers in the breathing system, and low fresh-gas flow with circle absorber can also help to reduce the heat loss.
Blood Loss and Transfusion Requirements
Burn excision can result in massive and sudden blood loss that increases with delay to primary burn excision, with a peak at 5 to 12 days after burn injury. Other factors that correlate with increased blood loss include older age, male gender, and larger body size; area of full-thickness (third-degree) burn; high wound bacteria counts (derived from quantitative tissue cultures); total wound area excised; and operative time. A mean blood loss of 2.6% to 3.4% of a patient’s blood volume for each %TBSA excised has been reported.
Several techniques have been used to reduce blood loss during primary burn excision. Intraoperative tourniquet use on burned extremities reduces overall blood loss. Postexcision compression dressings and topical epinephrine have been used to reduce blood loss during excision and grafting procedures. Application of bandages soaked in 1:10,000 epinephrine after excision of burned skin and/or use of thrombin spray, fibrin sealant, or platelet gel is effective in producing a bloodless surface for placement of skin grafts. Extremely high levels of catecholamines in the blood have been measured after the use of this technique. Sinus tachycardia and hypertension are common, and thus heart rate and blood pressure cannot be used to reliably titrate anesthetic or analgesic agents. Serious dysrhythmias are fortunately rare (See Box 18-2 ).
Subcutaneous crystalloid is injected in generous amounts using pressure-bags and Pitkin syringes (tumescent technique) to facilitate donor skin harvesting and reduce blood loss. Epinephrine and local anesthetics such as bupivacaine or lidocaine may be added to this therapy. Quantifying blood loss is typically difficult in burn patients, and transfusion is best guided by serial hematocrit estimations. Adequate venous access is a prerequisite to burn excision and grafting procedures. At least two intravenous access routes should be established (peripheral or central), and these lines should be sutured securely to prevent accidental dislodgment while positioning. Blood products should be readily available before excision begins. Femoral venous catheters placed through burned skin have been shown to be safe, although this issue has been questioned. The decision to transfuse blood products should be individualized by carefully weighing the risks of transfusion, including immunosuppression, versus the benefits of correcting anemia in the setting of hypermetabolism and increased oxygen demands. If blood loss is excessive, it is prudent to rule out coagulation abnormalities. Burn patients have a consumption coagulopathy that, combined with hemodilution during surgery, results in a clinically significant deficiency of coagulation factors II, VII, and X, despite reactive elevation of coagulation factor VIII and fibrinogen. Platelets or coagulation factors may need to be replaced, guided by the coagulation profile.
Although infrequently used in current clinical practice, intraoperative blood salvage in excisional burn surgery, using a cell saver, has been shown to recover more than 40% of shed red blood cells with acceptable levels of bacterial contamination and inflammatory mediators.
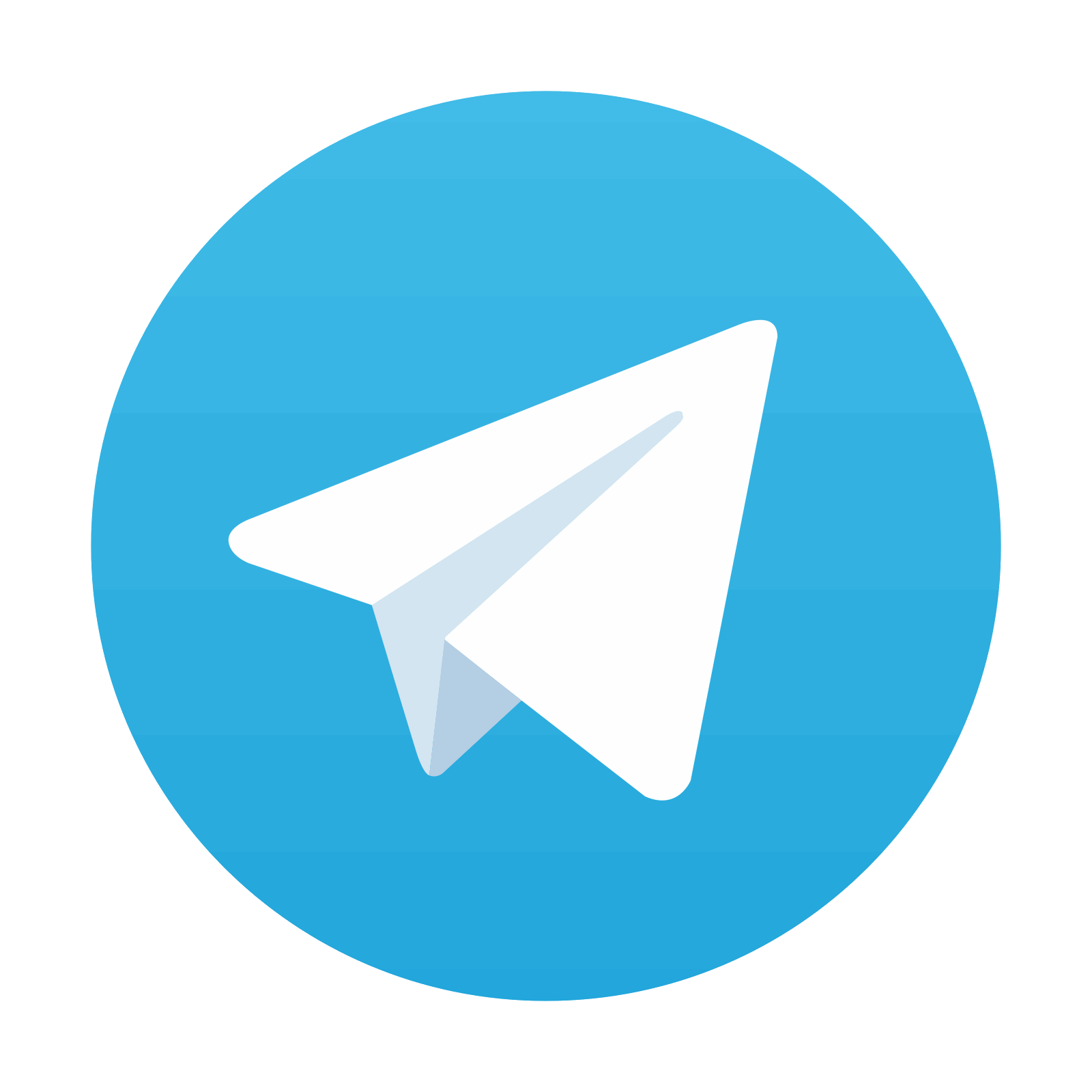
Stay updated, free articles. Join our Telegram channel

Full access? Get Clinical Tree
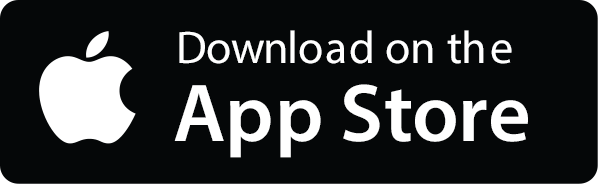
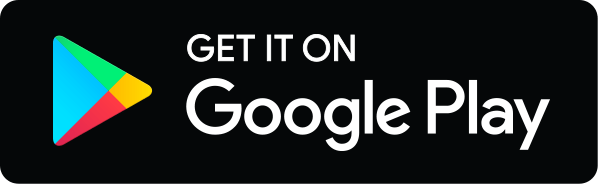