Size of the burn in percent of skin surface. According to the “9% rule” the body regions can be divided into areas which are multiples of 9. The calculation is slightly different in a child up to 10 years, because of a relatively larger head and smaller legs.
This chapter first presents the state of the art for burn care, which is then followed by the modern fluid treatment paradigm, i.e. the background for the large fluid needs of these patients. Different fluid treatment strategies will be discussed, and finally some special situations will be presented when there is need for additional knowledge and specialized practice procedures.
Modern burn care
Before addressing the details about burn fluid treatment, there is a need to present the big changes that have occurred recently (in the past 10–20 years) in modern burn care. This will lead to a better comprehension of the advanced medical needs of this patient group and the corresponding fluid treatment strategies.[1]
Incidence
In burn care, in the past 10–20 years there has been a decreasing incidence of burn injuries. This was first described in the United States but has also been shown for most western countries. A very different picture is found in the developing countries and Asia. In general, a decrease of about 30 percent is most often seen in western countries during that time period.[2]
Treatment outcome
At the same time, burn care treatment has significantly been developed and improved. Most obvious is the observation of the significant decrease in hospital mortality for burns, in the range of 70%. However, it is still important to stress that the number of deaths at the scene of the fire is still relatively constant, particularly for Sweden at about 100–120 deaths per year.[2] And these victims most often constitute individuals who are elderly, are physically or mentally disabled, or have drug problems. The reduced hospital mortality rate is claimed to be due to several factors, of which the most important are: modern fluid treatment, aggressive early surgical interventions, improved critical care, and newer and more potent antibiotics and local antimicrobials used in burn wound care.[1]
Another important effect of the early surgical excisional intervention is the length of hospital stay. Length of stay has decreased to approximately 25% of what it was in the 1980s–1990s. At that time, length of stay calculated as the number of days per % body surface area burned was approximately 3–4 days/% TBSA. Today, the corresponding value is less than 1 day/% TBSA for partial thickness burns and approximately 2 days/TBSA% for full thickness burns.[3] When examining mortality rates, they can be depicted as the burned surface area with a 50% predicted mortality risk (lethal dose [LD] 50%). In the mid-1980s the corresponding burn size (LD 50%) was 45% TBSA. Today, and dependent on age, the corresponding burn size for a young person (<60 years old) is 80–90% TBSA burned. The age-adjusted LD 50% are around a Baux index (Age+TBSA%) of 115.[4,5] Another important change in burn care was the introduction of outpatient burn care in the late 1990s; today, patients with burn injuries of up to 10–15% are often routinely treated as outpatients. Outpatient care is also offered for patients with larger burns when most of their open wounds have healed. From this perspective, it is interesting to note that the principles of fluid treatment have not changed since the late 1970s.[1,6,7]
Fluid balance pathophysiology in burns
The purpose of fluid treatment for the burn-injured is to maintain organ perfusion despite major leakage of fluid from the intravascular space. There are mainly two reasons for this fluid loss, which have both been extensively investigated and have a clear temporal pattern:
Negative imbibition pressure
When fluid losses were investigated in experimental models in the 1960s, the fluid loss could not be completely explained by an increase of permeability, and it was postulated that there needed to be another mechanism involved to explain the total fluid loss. In his thesis in 1961, Gösta Arturson predicted that there needed to be another factor involved to explain the total fluid loss in larger burns.[12] He then suggested that the fluid loss from the blood could be explained by a reduction of the interstitial tissue pressure. In the 1980s Lund and Reed were able to show in in vitro models that there is a build-up of a strong negative interstitial tissue pressure within burn-injured tissue, in the range of −25 to −50 mmHg. This negative gradient, which is called negative imbibition pressure and which explains most of the total fluid loss, is most pronounced immediately after the burn and remains for several hours afterwards (Figure 31.2).[8,9] Importantly, most of the total fluid loss seen is lost within these hours, and today we know that this is a reason for early hypovolemia despite the recommended fluid treatment. We will return to this later in the chapter when we discuss the fluid treatment in more detail.
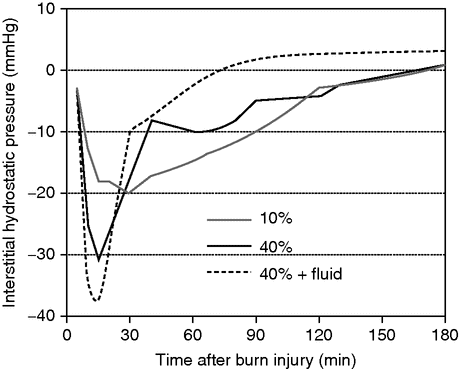
Negative imbibition pressure. Schematic drawing of the build-up of negative interstitial fluid pressure depending on the size of a burn injury and whether fluid is administered.
Interestingly, the fluids provided during fluid resuscitation seem to negatively affect the negative imbibition pressure: providing more fluid to the patient leads to a more negative tissue pressure and a larger fluid demand in the tissue, and correspondingly a larger total fluid need for the patient. The mechanism underlying the development of the negative fluid pressure is not fully understood, but it is suggested that it is due to effects on the tissue integrins, which are the components important for regulation of the hydrostatic pressure of the interstitium. The underlying mechanism is that the integrins lose their ability to maintain a positive effect on the pressure: as they are disintegrated by the burn injury to the tissue, their effect is lost and a negative tissue pressure is created. Furthermore, the burn injury effect on the build-up of a negative tissue imbibition pressure seems to be related to the size of the burn injury, a larger injury leading to a more pronounced negative tissue pressure.[8]
Permeability effects of the burn
It has long been known that burns cause fluid losses from the intravascular space. Another important mechanism is the increase in vascular permeability. Such an effect is seen for a burn injury corresponding to a body surface area of 5% or more. Again, all the details of this effect are yet not elucidated, but there are a number of mediators that have been found to be important. Temporally, in the burn-injured, this effect is also manifested rather early after the injury: most of it is seen within the first 8 hours after the burn. Of the mediators, serotonin [13–15] has been claimed to be the most important from the pathophysiological perspective. Other relevant mediators are: nitric oxide (NO), prostaglandins, and oxygen radicals (see below).[1] Histamine, which historically has been claimed to be important, has recently been questioned.[16]
Another important factor for the fluid loss from the vasculature is the onset of vasodilatation seen in most vascular beds owing to the continuous liberation of pro-inflammatory mediators in injured tissues. This vasodilatation increases the hydrostatic pressure in the microcirculation, leading to fluid loss into the interstitial compartment. It is important, then, to stress that the loss of fluid from the intravascular space affects all the factors that today are claimed as important for the transport of fluid across the capillary/venular wall.
If using the Starling equation to describe the transport of fluid across the capillary, all factors included in the equation are affected negatively (implying a larger fluid loss) in burns. According to the Starling equation, the filtration is dependent on the area of filtration, multiplied by the difference in hydrostatic pressure between capillary and interstitium, minus (the coefficient of filtration) times the difference in colloid osmotic pressure between the capillary and the interstitium. Among these factors, the coefficient of filtration increases greatly, often in the region of 20 times. For the latter parts of the formula, there is an increase in the hydrostatic pressure in the capillary (vasodilatation), together with a decrease in the colloid osmotic pressure in the capillary due to leakage; a decrease in interstitial pressure due to the imbibition pressure; and an increase in the colloid osmotic pressure in the interstitium (due to the leakage of colloid out of the vessels). All of these factors lead to an increase in fluid loss from the vasculature into the tissue. This affects most vascular compartments. The effect on the venular level is claimed to be most important from a quantitative perspective [1] and, although most of the transport across the vascular wall is mediated by smaller molecules, larger proteins are also lost, albeit at a lesser fraction. This is important to note as it underlies the arguments for so-called “colloid rescue” fluid resuscitation in situations of larger burns: this reduces the total colloid loss and corresponding fluid loss, and decreases the risk for compartmental complication.[17]
In clinical practice, the permeability increase is seen in the serum level of albumin, which decreases after the burn as albumin is lost from the intravascular space. It is important also to stress that the intravascular colloid osmotic pressure in the acute phase is dependent not only on albumin but also on the newly synthesized acute phase proteins. Because of uncertainties in the temporal pattern of permeability to proteins locally in the vasculature, no clear advice can be given as to the optimal time when colloids can be administered without the risk of being lost to the interstitium.
There has been an intense debate on when colloids can safely be administered during fluid resuscitation. Today, most burn surgeons would agree that it is adequate to provide colloid after 8–12 hours since the burn if needed to reduce total fluid volume. It is important to understand that providing colloid early may lead to colloid accumulation in the tissue, by early leakage, increasing the risk of higher colloid pressure in the interstitium which in turn increases tissue edema. However, it also needs to be appreciated that only 20% of the crystalloid fluid volume remains intravascularly, and that providing large volumes of crystalloid leads to decreased colloid osmotic pressure intravascularly and thus a further loss of fluid from this compartment. All these effects need to be appreciated when planning the fluid resuscitation for a patient with burns.
Fluid loss in burns – the temporal aspect
When caring for the burn-injured, it is important to examine and discuss the temporal aspects of the fluid loss and the recommended fluid therapy. This is especially the case as recent investigations have shown a clear temporal mismatch between the fluid loss and the fluid volume provided by present guidelines. As previously stated, most of the fluid loss due to the negative imbibition pressure takes place within the first 3–4 hours after the burn. The picture is somewhat different for the fluid loss due to the permeability increase. The most reliable data in humans suggest that this fluid loss occurs from the time of the injury and up to 8 or 10 hours after the burn. Although there is still ongoing debate on this topic, most would agree that the bulk of the loss is during the first 12 hours after the burn.[18,19] One should also take into account the permeability effects of a more or less continuous SIRS (systemic inflammatory response syndrome) reaction that is often seen after the burn, although the magnitude of this is less pronounced if the case is not complicated by sepsis.
Most important is that the present guidelines for fluid resuscitation, especially if based on a pure crystalloid strategy, do not fully account for this early fluid loss, and the patient may be claimed to be in a controlled hypovolemic situation during the first 12–16 hours after the burn. This will be commented on later when discussing the guidelines used for burn fluid resuscitation. In conclusion, it may be stated that the tissue edema reaches a maximum between the first 24 and 48 hours after the burn; this added fluid volume is slowly returned thereafter to the circulation and excreted as urine, often an ongoing process for 7 to 10 days after the burn.
Mediators important for fluid losses
A large number of mediators have been claimed to be important for the underlying mechanisms of fluid losses in burns. Most probable is that there are several that contribute to the fluid loss in different ways. The most important are: nitric oxide (NO), histamine, thromboxanes, prostaglandins (especially PGE2 and F2alpha), serotonin, proteases, oxygen free radicals, eicosanoids, bradykinin, complement factor C3, neuropeptides (especially substance P) and platelet activating factor (PAF), cytokines (interleukin-1,-2,-6,-8, tumor necrosis factor-alpha), and interferons.[1,20] Coagulations and the complement cascade system are thought to be early activated and play a significant role. Interest in the mediators and their possible roles in fluid loss is driven by the hope of finding a blocker that can stop, or reduce, the process. Some attempts have been made in this field, the most successful of which is the use of high-dose vitamin C (as an oxygen radical scavenger), which in a prospective randomized trial showed a reduction in fluid loss in the treatment group.[21–23]
Fluid treatment: practical guidelines
Much of the fluid treatment as we know it today is based on knowledge gained in the 1970s. As long ago as the nineteenth century, there were already theories suggesting that it was the fluid loss, and the corresponding change in the blood, that caused the negative outcomes prevalent at the time. Major breakthroughs in the fluid treatment for burns were made by Underhill who, in the 1920s, started to describe the pathophysiology of the burn injury in more detail. In 1940, following the Cocoanut Grove night-club disaster in Boston, the first attempts to use intravenous fluid treatment in a larger group of injured people were examined. The conclusion was that mortality was significantly lower than expected. In 1953, the first fluid formula to be based on burn size and patient weight was introduced by Evans.[1,20]
The formula most widely used today is the one that was published in 1973 by Charles Baxter, then working at the Parkland Memorial Hospital in Dallas. The formula states, based on experimental findings in dogs and humans, that 2–4 ml of Ringer’s solution per total burnt percent of body surface area (TBSA%) and kilogram body weight should be provided. Half of the calculated volume is given during the first 8 hours after the burn and the other half during the subsequent 16 hours. The main advantages are easily attainable fluid (Ringer’s lactate), low cost, and a treatment strategy that is easy to start and follow. A number of other formulae have been presented over the years, but none has the global impact of the “Parkland” strategy. Some of the alternatives are listed in Table 31.1.[1,20] Today, few centers in Europe or the United States use strategies other than the Parkland option.[24]
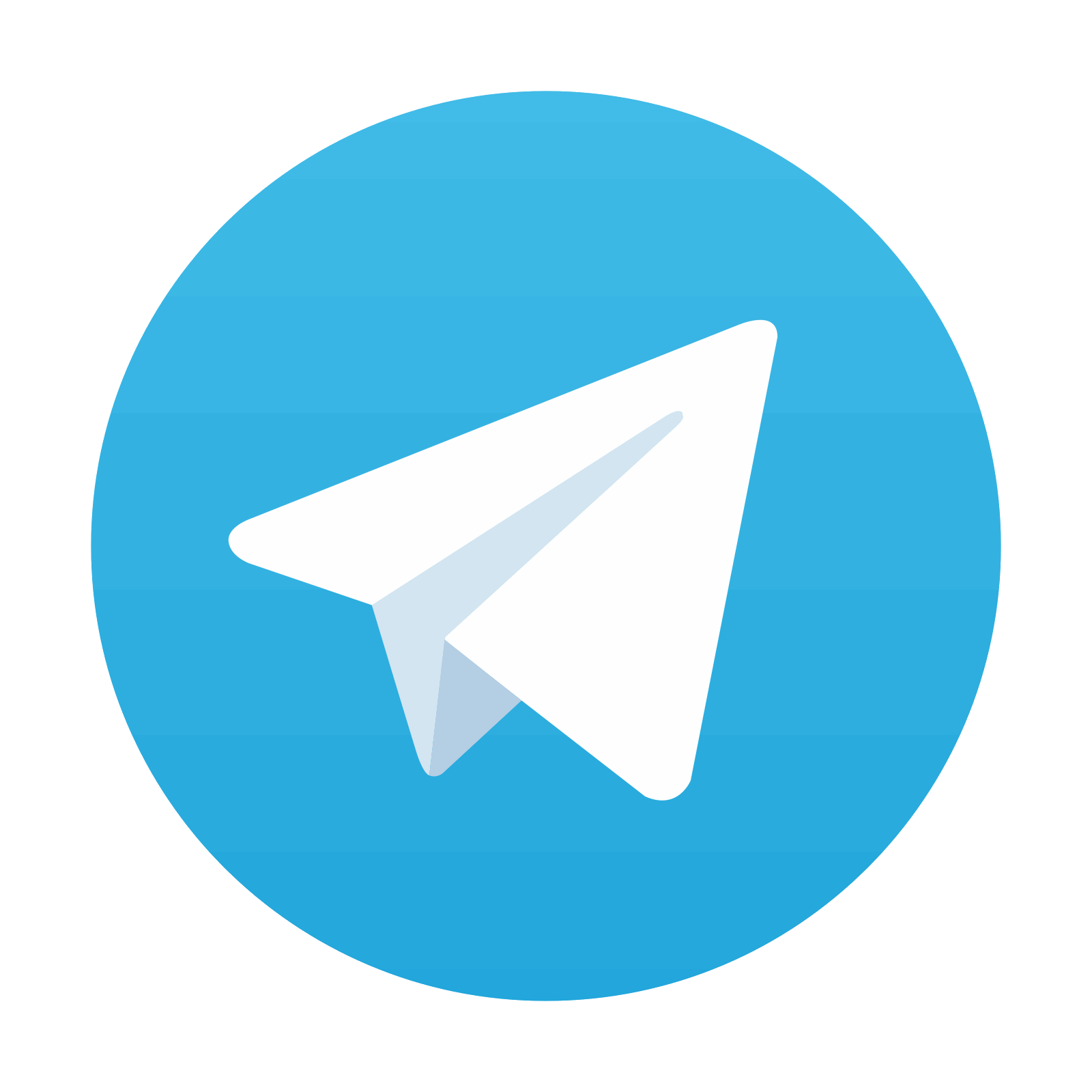
Stay updated, free articles. Join our Telegram channel

Full access? Get Clinical Tree
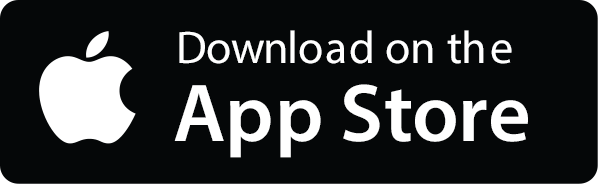
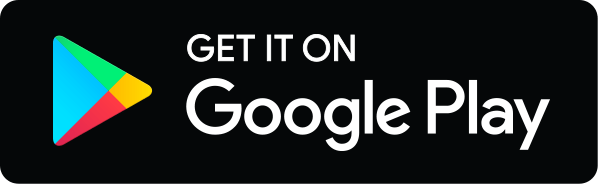