Colloid infusion during the resuscitation of acutely injured patients has been debated for some time. Acute burn injury leads to capillary permeability, which allows loss of intravascular albumin into the interstitial spaces of the acutely injured patient. Currently, the application of albumin or fresh-frozen plasma is considered after the initial 8-hour period postburn in an attempt to avoid loss of the colloid secondary to capillary permeability (99–102).
Adequate resuscitation is measured by end-organ perfusion. Currently, exact measures of end-organ perfusion are being developed and tested. A surrogate measure of the success of fluid administration is the measurement of urine output. Renal function is highly dependent on renal blood flow, which can be adequately assessed by the rate of urine output. Most practitioners view a urine production rate of 0.5 to 1.0 mL/kg ideal (or adjusted) body weight per hour as adequate. Secondary measures of perfusion are also important in the resuscitation plan. The combination of blood pressure, heart rate, oxygenation, and central venous pressure are used in tandem to determine the adequacy of treatment.
Measures of adequate resuscitation, such as blood pressure, heart rate, and central venous pressure used alone, must be monitored cautiously. Postburn tissue edema will decrease the accuracy of cuff blood pressure measurements, and the vasoconstriction caused by catecholamine release will adversely affect the accuracy of indwelling arterial lines. Central venous pressure measurements require the interaction of multiple physiologic and environmental parameters to provide the practitioner with meaningful measurements.
Patients who begin to lag in their urine output during resuscitation should have their fluid rates adjusted. Urinary rates of less than one third the predicted value based on the patient body weight over two consecutive hours should prompt an increase in intravenous fluid administration. On the other hand, patients running one third or more over their expected urine output may benefit from decreased fluid administration. A graded increase or decrease of the intravenous fluid rate of 20% per hour is a measured and conservative response to these situations. Those patients who do not respond as expected to calculated fluid administration or who require more than 6 mL/kg per percent TBSA fluid administration should be considered for more invasive monitoring, such as pulse-waveform analysis (FlowTrack, PICCO, LiDCO, and bedside Echo) or pulmonary artery catheter monitoring. With measurements obtained through this more advanced monitoring, a decision can be made to either support the CO or reduce the SVR, or both. Small doses of hydralazine may be used to reduce peripheral resistance in situations where the CO remains low. Hydralazine doses on the order of 0.5 mg/kg have been shown to be effective when used in this situation. In animal models of burn injury, sodium nitroprusside and verapamil decreased SVR and supported CO with good effect. This approach should be used with caution in the severely burn-injured patient to avoid further tissue hypoperfusion, which may exacerbate the condition of the partially burned tissues, increase fluid creep, worsen tissue edema, and may precipitate abdominal compartment syndrome (103).
At-risk patients for volume over-resuscitation include pediatric patients with an increased TBSA to weight, patients with inhalation injuries, electrical injuries, intoxication, and delayed fluid resuscitation. Fluid creep during resuscitation is worsened by exceeding the Parkland formula early or pushing high urine output during goal-directed therapy. Other factors that contribute to increased fluid administration include sedation and pain management with opioids, propofol, and benzodiazepines, or patient factors like morbid obesity (104–106).
Insensible water losses become more significant with larger TBSA burns. Evaporative water losses from the open wounds/burns usually peak on the third day postburn and then trail off until the wounds are completely closed. An estimation of insensible water losses may be calculated as follows:
The body surface area may be estimated using the formula of DuBois and DuBois as follows:
where W is the weight in kilograms and H is the height in centimeters. Initially, the replacement fluid is free water initially and then it is altered based on electrolyte measurements (107,108).
ELECTRICAL INJURY
Electrical injuries can be divided into those due to low voltage (<1,000 volts) and those due to high voltage (≥1,000 volts) (109). These injuries have varying patterns: Low-voltage injuries range from the circumoral injuries noted in children who have bitten home electrical cables to deaths caused by dropping electrical appliances in a bathtub full of water; high-voltage injuries have a range that includes the more severe episodes of instant death, massive tissue loss, and secondary clothing ignition. Some of the less dramatic injuries include thermal injury, central nervous system–related trauma, and fractures. With high-voltage injuries, there is a high ratio of limb amputations, highlighting the danger of this modern-day source of power. Herein, we will concentrate on high-voltage injuries.
Electrical energy interacts with human anatomy following the basic principles of physics. Current flowing through tissue is related to the voltage drop across the resistance of that tissue. Heat produced by this current can be represented mathematically as follows:
where I is the current, R is the resistance, and T is the time in seconds. Body tissues have differing electrical resistances. Given the above equation, it appears that differing tissues would create varying degrees of heat and subsequent damage; interestingly, clinical findings do not wholly support this concept. The highest resistance is found in the bone, fat, and tendons, whereas the lowest resistance has been identified in the muscles, blood, and nerves; skin has an intermediate resistance. Clinical findings in electrically injured patients support the idea that the body represents a volume conductor with a resistance on the order of 500 to 1,000 ohms. In this model, the relative differences in tissue resistance are small enough that the body is considered a single resistor. Heat generated by the current flowing through the resistor is related to the cross-sectional area of the entry or exit wound and the local anatomy.
Contact wounds on the hands and feet are common. Each of the contact areas might have a low cross section, releasing more heat in that area; as the current crosses the “bottleneck” areas of the ankles and wrists, there may be more tissue damage generated at those sites. At its most extreme, heat released by high-voltage injuries produces coagulation necrosis of the tissues and varying other effects on the organs as the electricity passes through.
Arc injuries are less common, but just as destructive. Electricity can travel 2 to 3 cm/10,000 volts, and may travel 10 ft or more to its target. Temperatures at the contact points range from 2,000 to 4,000°C, with spikes of up to 20,000°C; this intense temperature leads to severe and deep tissue damage.
Electrical injuries have specific organ effects in addition to the thermal injury described above. With high-voltage injuries, cardiac standstill and ventricular fibrillation are the most lethal cardiac injuries. Other electrocardiographic (ECG) findings and rhythm changes that have been reported include atrial fibrillation, focal ectopic arrhythmias, supraventricular tachycardia, right bundle branch block, and nonspecific ST-T segment changes. These clinical findings are thought to be associated with direct myocardial muscle damage, coronary vasospasm, and coronary endarteritis.
Renal injury may be direct, although this is rare, or may take on the more familiar form of acute renal failure secondary to rhabdomyolysis. Large quantities of muscle protein, hemoglobin, and other tissue proteins released from the tissues coagulated by high voltage and current are filtered into the renal tubules, causing acute renal failure with oliguria or polyuria. Up to 15% of patients injured in high-voltage accidents will suffer from this type of renal injury.
Central nervous system injury ranges from the devastating effect of high voltage and current on the brain and brainstem, leading to instant death or to more subtle findings. Altered levels of consciousness with varying degrees of recovery have been reported, while progressive neurologic deterioration has been noted in both the central and the peripheral nervous systems. With high-voltage injuries, progressive deterioration of the microvascular nutrient vessels to the nerves has been identified, and is thought to lead to ischemia, necrosis, and fibrosis of the injured nerve; progressive loss of function can be seen as a late developing problem (110).
Other organ systems may be directly affected by the passage of current through them. Each is susceptible to both the current and the resistance-induced production of high temperatures and tissue damage. Cataracts may form in the eyes of a patient who has had an electrical injury in which the current pathway is through the orbits; this complication has been reported in up to 30% of patients suffering from this injury (111,112).
INHALATION INJURY
The pathophysiology associated with smoke inhalation injury falls into three broad categories: (i) upper airway injury; (ii) asphyxiant gases and hypoxic environments; and (iii) carbonaceous particle deposition (113–116).
The diagnosis of inhalation injury is based on several site-specific and clinical findings surrounding each burn patient. For example, closed space injuries or explosions are some of the circumstances surrounding inhalation injury. Noxious fumes noted at the scene by the scene responders, as well as facial burns noted on the patient, are other findings consistent with inhalation injury. Clinical findings on examination, such as large burns, carbonaceous sputum, hoarseness, or an abnormal lung examination, are associated with this injury, and elderly patients are more susceptible to inhalation injury.
Once inhalation injury is suspected, upper airway pathology should be expected. Heated smoke or ambient air may injure the supraglottic airway from the lips to the vocal cords. This injury may occur abruptly, leading to significant edema and swelling of the face and oropharynx, as well as affecting the region around the vocal cords.
True heat injury below the cords is rare, with the exception of steam injuries or ignition of flammable gases in the airway. Signs and symptoms of a true airway burn injury include hoarseness; stridor and/or wheezing; carbonaceous sputum; singed nasal hair, eyebrows, or facial hair; and edema or inflammatory changes in the upper airway. The resultant upper airway edema formation can threaten the airway and the patient’s breathing.
Asphyxiant gases and hypoxic environments lead to the second area of pathology associated with inhalation injury. In fires involving structures, the ambient oxygen level markedly decreases; this lack of oxygen may lead to carbon monoxide generation, as this molecule is a byproduct of incomplete combustion in the burning structure. Carbon monoxide binds tightly to hemoglobin, reducing the amount of oxygen delivered to end organs, which may cause a hypoxic injury. Aside from carbon monoxide, other toxic gases can be released by the flames. Cyanide generation is associated with burning plastics; this molecule is highly lethal. Treatment of carbon monoxide intoxication includes a high concentration of oxygen and, sometimes, hyperbaric oxygen. Cyanide poisoning treatment includes delivery of oxygen, as well as a three-part regimen to bind the cyanide compound in the blood.
The third broad area of pathology associated with smoke inhalation injury is related to the deposition of carbonaceous particles in the airway. The flame-generated toxins that are bound to the carbon particles will slowly be released after the latter are deposited in the lungs, inducing a chemical tracheal bronchitis. This effect is manifested by impaired ciliary function and edema, significant inflammation, and ulceration or necrosis of the respiratory epithelium. The clinical sequelae of tracheal bronchial injury include bronchorrhea, bronchospasm, distal airway obstruction, and atelectasis, as well as pneumonia. Epithelial injury leads to sloughing of the mucosa and blockage of the airways with this cellular debris. Air trapping in this situation leads to atelectasis and the development of barotrauma and pneumonia.
Treatment for each area of pathology is based on standard clinical practice. When presented with a patient who is suspected of having an inhalation injury and upper airway edema, endotracheal intubation to protect the airway should be performed early to avoid the consequences of airway compromise. Patients suffering from carbon monoxide exposure, and showing clinical signs of intoxication, should be treated with 100% oxygen to displace the molecule from hemoglobin. If immediately available, and if the patient is stable, high levels of carboxyhemoglobin and severe neurologic symptoms should be treated with hyperbaric oxygen therapy. The tracheal-bronchial injury associated with inhalation injury and carbonaceous particle deposition should be treated with humidified oxygen by face mask, and frequent examination of the airway should be performed to evaluate for signs of compromise that may require endotracheal intubation.
Cyanide toxicity presents clinically with lethargy, nausea, headache, weakness, and coma. Cyanide combines with cytochrome oxidase, thereby blocking oxygen use and inhibiting high-energy phosphate compound production. Cyanide toxicity begins at 0.1 μg/mL of serum and quickly leads to death at concentrations of 1 μg/mL. Laboratory studies show a decreased arteriovenous oxygen difference with severe metabolic acidosis; this acidosis is unresponsive to fluids and oxygen administration.
S-T segment elevations may be seen on the patient’s electrocardiogram, mimicking a myocardial infarction. Treatment of this condition includes the administration of 100% oxygen and a three-part medication regimen. Initially, the administration of amyl nitrate pearls, by inhalation for 15 to 30 seconds every minute, is followed by 10 mL 3% sodium nitrate solution (300 mg) intravenously over 3 minutes, repeated at one-half the dosage in 2 hours if symptoms persist or recurrent signs of toxicity are present. Sodium thiosulfate is the final medication, given in a dose of 50 mL of a 25% solution (12.5 g) intravenously over 10 minutes, repeated at half the dosage in 2-hour intervals if persistent or recurrent signs of toxicity are present.
INFECTION CONTROL
Historically, mortality from burn injury was associated with burn shock (117–119); the loss of fluid through the burned and damaged skin, as well as fluid shifts related to the release of inflammatory mediators, led to hypovolemia and unrecoverable end-organ failure. As our understanding of the injury suffered during burns improved, so did the survivability of these injuries. Currently, burn shock is well controlled by our fluid resuscitation regimens. The new challenge in burn injury is the concurrent development of infection in a compromised host. Burn injury is associated with a burn size–dependent depression in the immune system in which bacterial, fungal, and viral elements are better able to breach the defense mechanisms of the body, thereby worsening the injury.
As fluid resuscitation techniques advanced, wound colonization and sepsis became a leading factor in morbidity and mortality. The advent of topical antibiotic/anti-infective agents addressed this new area of pathology. Subsequent movement toward early wound excision and skin grafting led to improvements in the rates of wound sepsis and its complications. Although the burn wound has become less of a risk for infection, other portals of entry have persisted in plaguing the burn patient. With our current methods of intubating the respiratory, vascular, and genitourinary systems, we expose the burn patient to other portals of entry for pathogens.
NUTRITION
Burn patients present to the ICU in a severe inflammatory state. This state, along with a wide variety of prehospital factors and premorbid conditions, provides the practitioner with a challenging nutritional problem. Nutritional support for the burn patient can be addressed in several steps: first, an assessment of the patient’s initial nutritional status, and second, monitoring of his or her nutritional status throughout the hospital course, and adjustments based on the monitoring measures. By using a combination of variables, including burn size and severity, time from injury, physical parameters such as age, weight, and the presence of other medical factors, nutrition support can be tailored to each burn patient.
Nutritional assessment begins with measurement of the patient’s weight, estimation of the patient’s calorie and protein needs, measurement of the patient’s serum albumin, prealbumin, and C-reactive protein levels (120–133). Pre-existing illnesses should prompt the practitioner to make adjustments in the rate of feeding, use of additional medications, and the need for additional nutrient support.
Glutamine supplementation has been shown to improve morbidity and mortality when administered to critically ill patients. This response appears to improve with increasing doses of the amino acid, and parenteral administration appears to have an improved response over enteral administration. Not all of the trials reported to date have shown a definitive benefit, and the general consensus is that a large randomized controlled trial would be needed to confirm or refute the benefits of glutamine administration (134,135).
Physiologically, glutamine affects the immune system, the anti-oxidant status, glucose metabolism, and heat shock protein response. These physiologic effects appear to provide benefit with regard to gastrointestinal mucosal integrity, wound healing, gram-negative bacteremia and infection—including with Pseudomonas—as well as a reduction in mortality, and possibly cost savings to burn patients. At the time of this writing, a consensus has not been reached on the length of time for glutamine therapy, the optimal dose, and definite safety aspects of the supplementation of glutamine in critically ill and burn patients. Most studies suggest that clinically important differences appear to commence at doses over 0.2 g/kg body weight per day, and most trials have used 15 to 30 g/day glutamine supplementation.
WOUND MANAGEMENT
With the exception of chemical burns, for which prompt water irrigation to remove the offending agent is required, no specific treatment of the thermal burn wound is needed in the prehospital setting (113,136,137). The patient should be covered with a clean sheet and blanket to conserve body heat and minimize burn wound contamination during transport to the hospital. The application of ice or cold-water soaks, when initiated within 10 minutes after burning, may reduce tissue heat content and lessen the depth of thermal injury. If cold therapy is used, care must be taken to avoid causing hypothermia; this is accomplished by limiting this form of therapy to 10% or less of the body surface and only for the time required to produce analgesia.
Following admission to the burn center, definitive care of the burn wound can begin. Daily wound care involves cleansing, debridement, and dressing of the burn wound. On the day of injury, the burn wound is best cleansed by means of hydrotherapy, a practice that has been used in the treatment of burn patients for many years and remains an integral part of current treatment plans. Hydrotherapy is accomplished by means of showering or use of a spray table. Showering is often used for ambulatory patients who remain capable of independent, or near-independent, wound care. Patients who are near to discharge are encouraged to use the shower, especially if showering is to be used at home.
Use of a spray table is generally reserved for newly admitted patients, those with limited mobility, or those with large open wounds. The patient is placed on the table, and the wounds are washed and rinsed with running water. As an alternative, a stretcher or plinth can be placed over a Hubbard tank. The patient is placed on the stretcher, and the wounds are washed and rinsed as described previously.
Wound debridement involves the removal of all loose tissue, wound debris, and eschar (nonviable tissue); debridement of a burn wound is accomplished through mechanical, chemical (enzymatic), or surgical means.
Surgical, or sharp, debridement requires the use of scalpels and scissors to debride wounds of loose, necrotic tissue. Care must be taken to avoid excessive debridement that results in bleeding and pain. Bleeding may indicate injury to the healthy underlying tissue. In most instances, sharp debridement should be carried out in the operating room to ensure adequate debridement and hemostasis. Definitive management of third-degree burn wounds and deep second-degree injuries may require tangential excision of the eschar or necrotic tissue followed by wound closure with skin grafts or other wound closure adjuncts. This process will not be reviewed here.
Multiple topical antimicrobial agents are used in burn wound care. Mafenide acetate (Sulfamylon), silver sulfadiazine (Silvadene), and silver nitrate are the three most commonly used topical antimicrobial agents for burn wound care. Each agent has specific limitations and advantages with which the physician must be familiar to ensure patient safety and optimal benefit. Mafenide acetate and silver sulfadiazine are available as topical creams to be applied directly to the burn wound, whereas silver nitrate is applied as a 0.5% solution in occlusive dressings. Either cream is applied in a half-inch (about one-third of a centimeter) layer to the entire burn wound in an aseptic manner after initial debridement, and reapplied at 12-hour intervals or as required to maintain continuous topical coverage. Once daily, all of the topical agents should be cleansed from the patient using a surgical detergent disinfectant solution and the burn wounds examined by the attending physician. Silver nitrate is applied as a 0.5% solution in multilayered occlusive dressings that are changed twice daily.
Mafenide acetate burn cream is an 11.1% suspension in a water-soluble base. This compound diffuses freely into the eschar, owing to its high degree of water solubility. Mafenide is the preferred agent if the patient has heavily contaminated burn wounds or has had burn wound care delayed by several days. This agent has the added advantage of being highly effective against gram-negative organisms, including most Pseudomonas species. Physicians using this agent must be aware of several potential clinical limitations associated with its use. Hypersensitivity reactions occur in 7% of patients, and pain or discomfort of 20 to 30 minutes duration is common when it is applied to partial-thickness burn wounds. This agent is also an inhibitor of carbonic anhydrase, and a diuresis of bicarbonate is often observed after its use. The resultant metabolic acidosis may accentuate postburn hyperventilation, and significant acidemia may develop if compensatory hyperventilation is impaired. Inhibition of this enzyme rarely persists for more than 7 to 10 days, and the severity of the acidosis may be minimized by alternating applications of mafenide with silver sulfadiazine cream every 12 hours (138).
Silver sulfadiazine burn cream is a 1% suspension in a water-miscible base. Unlike mafenide, silver sulfadiazine has limited solubility in water and, therefore, limited ability to penetrate into the eschar. The agent is most effective when applied to burns soon after injury to minimize bacterial proliferation on the wound’s surface. This agent is painless on application, and serum electrolytes and acid–base balance are not affected by its use. Hypersensitivity reactions are uncommon; an erythematous maculopapular rash sometimes seen subsides on discontinuation of the agent. Silver sulfadiazine occasionally induces neutropenia by a mechanism thought to involve direct bone marrow suppression; white blood cell counts usually return to normal following discontinuation. With continual use, resistance to the sulfonamide component of silver sulfadiazine is common, particularly in certain strains of Pseudomonas and many Enterobacter species. However, the continued sensitivity of microorganisms to the silver ion of this compound has maintained its effectiveness as a topical antimicrobial agent.
A 0.5% silver nitrate solution has a broad spectrum of antibacterial activity imparted by the silver ion. This agent does not penetrate the eschar, because the silver ions rapidly precipitate on contact with any protein or cationic material. Use of this agent is not associated with more intense wound pain, except from the mechanical action required for dressing changes. The dressings are changed twice daily and moistened every 2 hours with the silver nitrate solution to prevent evaporation from increasing the silver nitrate concentration to cytotoxic levels within the dressings. Transeschar leaching of sodium, potassium, chloride, and calcium should be anticipated, and these chemical constituents should be appropriately replaced. Hypersensitivity to silver nitrate has not been described. Mafenide acetate, silver sulfadiazine, and 0.5% silver nitrate are effective in the prevention of invasive burn wound infection; however, because of their lack of eschar penetration, silver nitrate soaks and silver sulfadiazine burn cream are most effective when applied soon after burn injury.
PAIN MANAGEMENT
Because burn pain is variable in its degree and time course, reliance on a single analgesic regimen is unreliable at best and unsuccessful at worst. Conversely, the diverse spectrum of burn patients—adult versus children, large burns versus small, ICU nursing versus ward setting—makes the routine individualization of analgesic plans overwhelming and impractical. Our recommendation is to determine an analgesic regimen for each individual patient based on two broad categories: the assessed clinical need for analgesia and the limitations imposed by the patient.
The first step is to address background, procedural, postoperative, and breakthrough pain treatment separately, and then consider individual drug choices based on patient limitations. To reinforce this type of approach to analgesic management, detailed institutional guidelines to help physicians and nurses choose and administer specific analgesics are recommended.
Background Pain
In general, because it is a pain of continuous nature, background pain is best treated with mild-to-moderately potent, longer-acting analgesics administered so that plasma drug concentrations remain relatively constant throughout the day. Examples include the continuous IV infusion of fentanyl or morphine (with or without patient-controlled analgesia [PCA]), oral administration of long-acting opioids with prolonged elimination (methadone) or prolonged enteral absorption (sustained-release morphine, sustained-release oxycodone), or oral administration on a regular schedule of short-acting oral analgesics (oxycodone, hydromorphone, codeine, acetaminophen). Such analgesics should almost never be administered on an as-needed (PRN) basis during the early and middle phases of hospitalization.
Procedural Pain
In contrast, procedural pain is significantly more intense but shorter in duration; therefore, analgesic regimens for procedural pain are best composed of more potent opioids that have a short duration of action. Intravenous access is helpful in this setting, with short-acting opioids (fentanyl) offering a potential advantage over more longer-acting agents (morphine, hydromorphone). When intravenous access is not present, orally administered opioids (morphine, hydromorphone, oxycodone, codeine) are commonly used, although their relatively long duration of action (2 to 6 hours) may potentially limit postprocedure recovery for other rehabilitative or nutritional activities. Oral transmucosal fentanyl and nitrous oxide are useful agents when IV access is not present due to their rapid onset and short duration of action.
Postoperative Pain
Postoperative pain deserves special mention because increased analgesic needs should be anticipated following burn wound excision and grafting. This is particularly true when donor sites have been harvested, as these are often the source of increased postoperative pain complaints. In contrast, pain from excised/grafted burns may increase, decrease, or not change postoperatively compared to preoperatively. Typically, this increased analgesic need in the postoperative period is limited to 1 to 4 days following surgery before returning to, or falling below, preoperative levels.
Breakthrough Pain
Breakthrough pain occurs at rest when background analgesic therapy is inadequate. Breakthrough pain occurs commonly in burn patients, particularly in early stages of hospitalization until a stable, appropriate, and individualized pharmacologic regimen can be determined for each patient. Analgesia for breakthrough pain can be provided with IV or oral opioids. When breakthrough pain occurs repeatedly, it is an indication to re-evaluate and likely increase the patient’s background pain analgesic regimen, as it may be inadequate in terms of analgesic dose and/or frequency. Tolerance develops rapidly in these patients and may initially manifest as breakthrough pain.
Patient Limitations
As stated above, the presence of intravenous access directly influences analgesic drug choice, particularly in children. Similarly, patients who are endotracheally intubated and ventilated are somewhat protected from the risk of opioid-induced respiratory depression; thus, opioids may be more generously administered in these individuals, as is often required for painful burn debridements. Also, individual differences in opioid efficacy should be considered in all patients, including opioid tolerance in patients requiring prolonged opioid analgesic therapy or in those with pre-existing substance abuse histories.
An appropriate rationale is to titrate the drug dose to the desired effect, rather than to rely on a particular textbook dose for all patients. Because of the development of drug tolerance with prolonged medical use or recreational abuse of opioids (i.e., increasing drug doses are required to attain adequate levels of analgesia), opioid analgesic doses needed for burn analgesia may exceed those recommended in standard dosing guidelines. Furthermore, because of cross-tolerance, tolerance to one opioid analgesic usually implies tolerance to all opioid analgesics. One clinically relevant consequence of drug tolerance is the potential for opioid withdrawal to occur during inpatient burn treatment. Thus, the period of inpatient burn care is not an appropriate time to institute deliberate opioid withdrawal or detoxification measures in tolerant patients, because such treatment ignores the very real analgesic needs—background pain and procedural pain—of these patients. Similarly, when reductions in analgesic therapy are considered as burn wounds close, reductions should occur by careful tapering, rather than abrupt discontinuation of opioids, to prevent the acute opioid withdrawal syndrome.
Anxiolysis in the Treatment of Burn Pain
Current aggressive therapies for cutaneous burns, together with the qualities of background and wound care pain, make burn care an experience that normally induces anxiety in a large proportion of adult and pediatric patients. Anxiety, in itself, can exacerbate acute pain. This has led to the common practice in many burn centers of using anxiolytic drugs in combination with opioid analgesics. Intuitively, this practice seems particularly useful in premedicating patients for wound care, to diminish the anticipatory anxiety experienced by these patients prior to and during debridement. Low-dose benzodiazepine administration significantly reduces burn wound care pain scores and narcotic requirements. It appears that the patients most likely to benefit from this therapy are not those with high trait or premorbid anxiety, but rather those with high state or the time of the procedure anxiety, or those with high baseline pain scores. Other nonpharmacologic anxiolysis techniques, such as hypnosis and behavioral therapy, could also be considered.
TEAM APPROACH TO BURN PATIENTS
The management of the burned patient is a multidisciplinary effort of burn care professionals to provide optimal care to the burn patient. This multidisciplinary care spans the early resuscitative phases of care through the long-term rehabilitation and reconstructive phases. The Burn Center Director coordinates all activities of the multidisciplinary care of the critically ill burn patients. Team members include burn surgeons, plastic and reconstructive surgeons, critical care specialists, anesthesiologists, critical care burn nurses, physical therapists, occupational therapists, clinical nutritional specialists, psychologists, social workers, and pastoral care support personnel. This multidisciplinary approach affords the patients and their families state-of-the-art resources for optimal outcome, education, and rehabilitation. This concept of team care, originating in the 1950s when the first burn centers opened, has persisted to this day and is a model of coordinated, interdisciplinary, outcome-driven patient care.
Key Points
- Role of inflammation and inflammatory mediators in the depression of cardiac function and increase in capillary permeability.
- Smoke inhalation and its effect on mortality from burn injury.
- Renal replacement therapy and its potential role in modulating the inflammatory effects of burn injury.
- Modulating the hypermetabolic response to burn injury medically as well as reducing the loss of bone density in patients with severe burn injuries.
- Addressing the effects of burn injury on the immune system and reducing the incidence of infection through VAP bundles, catheter management, and improving the rate of wound closure. Further study in supporting the immune system despite the burn injury.
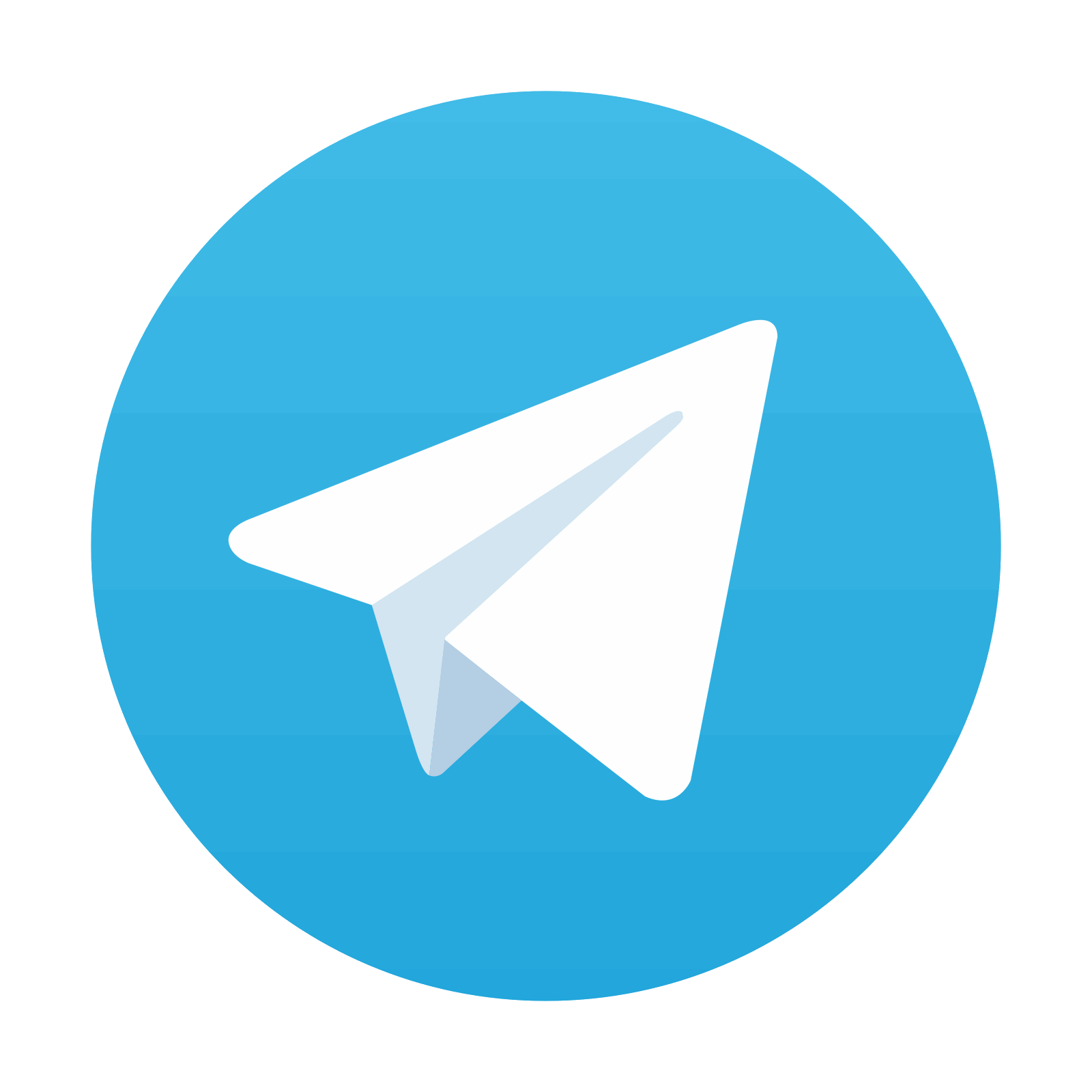
Stay updated, free articles. Join our Telegram channel

Full access? Get Clinical Tree
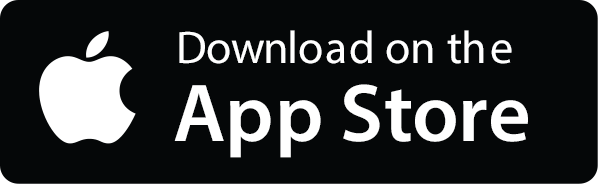
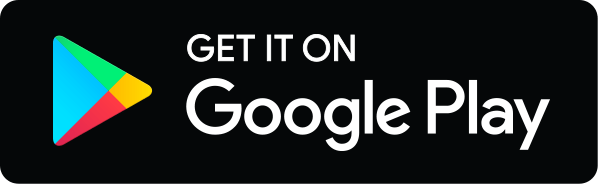