Fig. 22.1
T1 axial (a) and coronal (b) post-gadolinium-enhanced MRI showing multiple peripherally enhancing metastatic lesions in an individual with known metastatic breast cancer
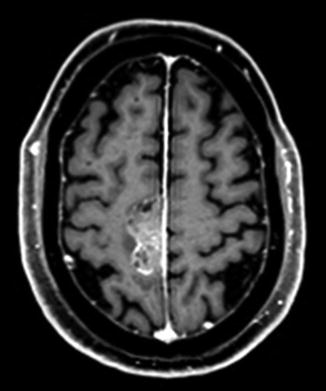
Fig. 22.2
T1 axial post-gadolinium-enhanced MRI showing a dural-based mass lesion along the right side of the falx, histologically confirmed to be a brain metastasis from prostate cancer
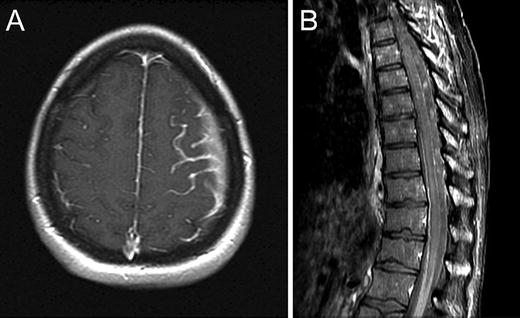
Fig. 22.3
Axial T1 post-contrast brain MRI (a) and sagittal post-contrast spine MRI (b) demonstrating abnormal leptomeningeal enhancement due to leptomeningeal metastasis
Brain metastases characteristically present with focal neurologic dysfunction related to the site of involvement, or progressively worsening headache. Typical radiographic features are a peripherally enhancing lesion or lesions occurring at the cortical/subcortical junction. Posterior fossa lesions, cystic lesions, and tumors with an imaging appearance similar to primary brain tumors may also occur. Headache, confusion/depressed level of consciousness, and cranial neuropathies are the most common presenting signs and symptoms of meningeal carcinomatosis, with imaging showing leptomeningeal or subependymal enhancement. The diagnosis of leptomeningeal cancer is made when cerebrospinal fluid cytology shows malignant cells, and/or characteristic neuroimaging features are present in the appropriate clinical context.
Primary Brain Tumors
Unlike brain metastasis, primary brain tumors arise from cells normally found in the brain (Fig. 22.4a). The overall incidence of primary brain tumors in adults is 21.03/100,000 [2]. Benign tumors are more common (10.5/100,000). Meningioma occurs more commonly in women, with an estimated incidence of 8.36/100,000 person-years in women compared to 3.61/100,000 in men [2]. The incidence increases with age and with estrogen supplementation. Additional risk factors include elevated body mass index, low self-rated physical activity levels, and a history of uterine fibroids [3]. Meningiomas are extra-axial, dural-based mass lesions that characteristically demonstrate avid homogeneous contrast enhancement (Fig. 22.4b), and are graded on a World Health Organization (WHO) scale from I to III depending on their histologic characteristics. Complete surgical removal, when possible, provides the greatest chance of cure. Radiation therapy is an effective treatment for residual or recurrent disease, and is also important in the treatment of grade II and III tumors as these carry a higher risk of recurrence.
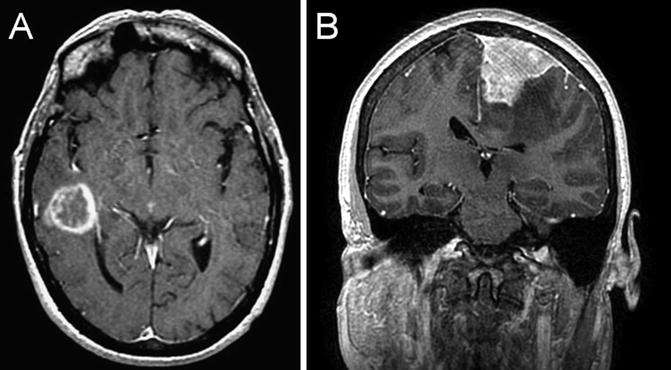
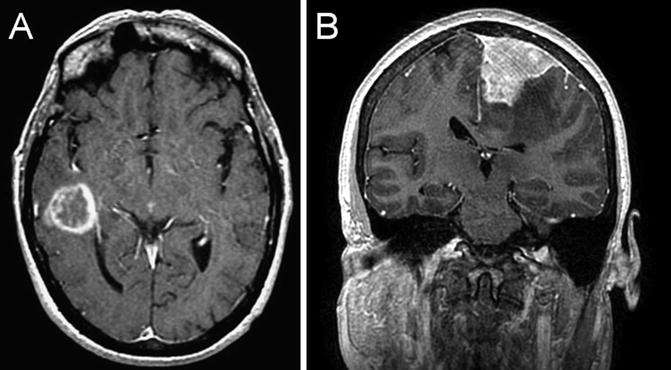
Fig. 22.4
T1 coronal post-gadolinium-enhanced MRI showing an intraaxial enhancing lesion, histologically confirmed to be a glioblastoma (a). T1 coronal post-gadolinium-enhanced MRI showing a dural-based homogeneously enhancing lesion, histologically confirmed to be a meningioma (b)
Gliomas are the most common malignant brain tumor in both adults and children, and represent 1.4 % of all new cancer cases diagnosed in the USA. They comprise 36 % of all brain and CNS tumors, and 81 % of all malignant brain tumors [2]. Gliomas are graded from WHO grades I to IV, with grades II (Fig. 22.5a, b) and III (Fig. 22.6a–c) characterized by increasing cellular atypia and anaplasia respectively, and WHO grade IV (Fig. 22.7a–e) demonstrating vascular proliferation and necrosis. Medulloblastoma (Fig. 22.8a, b), ependymoma (WHO grades II–III) (Fig. 22.8c), pilocytic astrocytoma (WHO grade I) (Fig. 22.8d), and diffuse infiltrative pontine glioma (WHO grade II) (Fig. 22.8e) are more often seen in children, while glioblastoma (WHO grade IV) is the most common malignant brain tumor in adults.
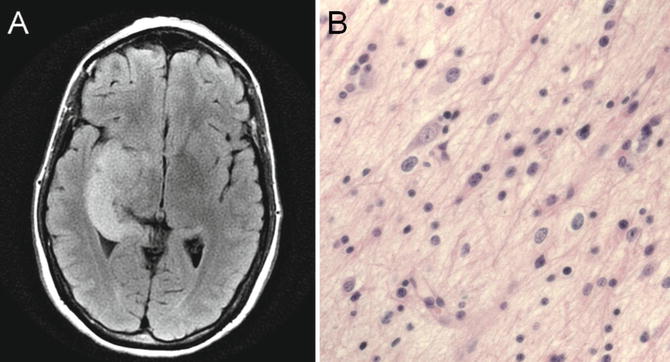
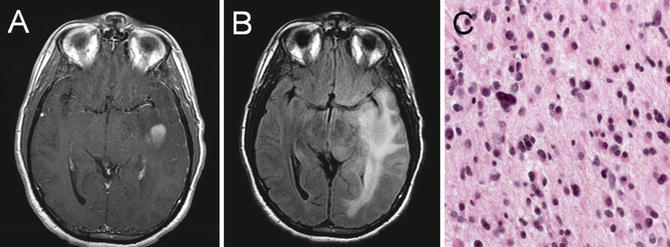
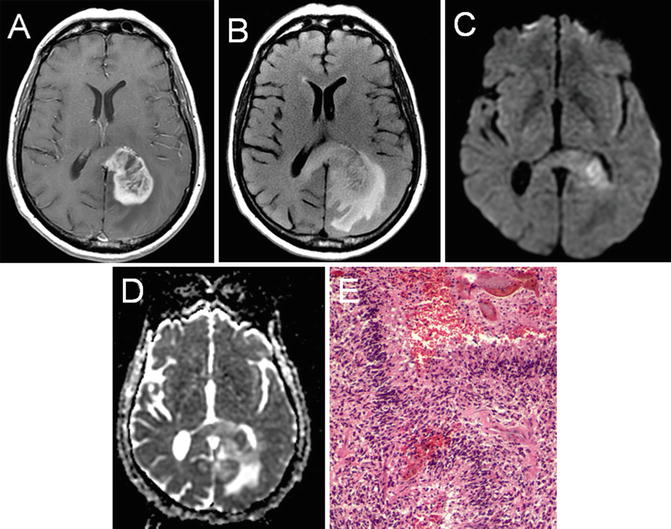
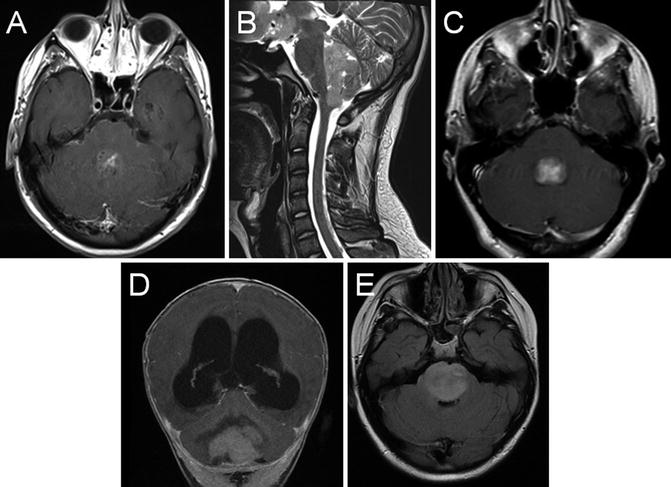
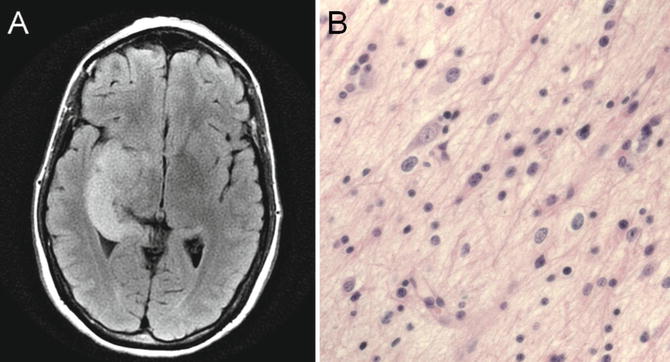
Fig. 22.5
T2 fluid-attenuated inversion recovery (FLAIR) MRI showing a T2 hyperintense, expansile abnormality in the right temporal lobe (a). Histologic analysis revealed a grade II astrocytoma that showed increased cellularity, pleomorphism, and infiltration (b)
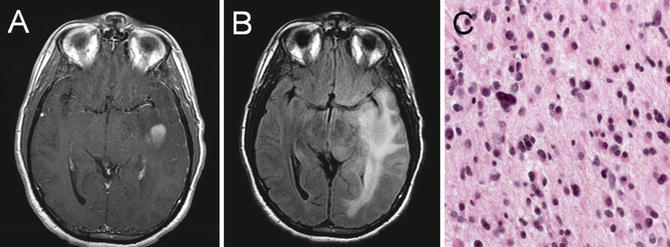
Fig. 22.6
T1 axial post-gadolinium-enhanced MRI (a) and T2 axial fluid-attenuated inversion recovery (b) images showing a left temporal anaplastic astrocytoma with a central focus of homogeneous enhancement. Histologic analysis revealed a grade III astrocytoma that showed high cellularity, pleomorphism, and mitotic figures (c)
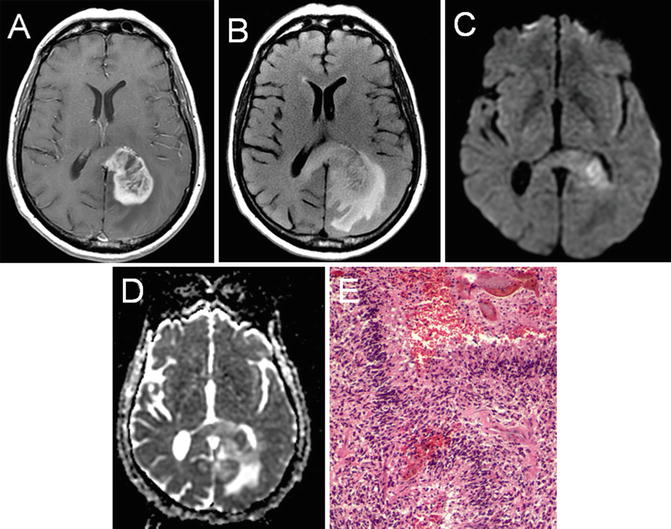
Fig. 22.7
T1 axial post-gadolinium (a), T2 FLAIR (b), diffusion-weighted imaging (c), and apparent diffusion coefficient (d) imaging of a left-hemisphere glioblastoma. The peripheral enhancing pattern and nonenhancing expansile changes crossing the midline via the corpus callosum are characteristic. DWI (c) and ADC (d) imaging reveal an area of diffusion restriction consistent with increased cellular density in the region. Histologic analysis revealed a grade IV glioblastoma that showed high cellularity, pleomorphism, microvascular proliferation, and necrosis (e)
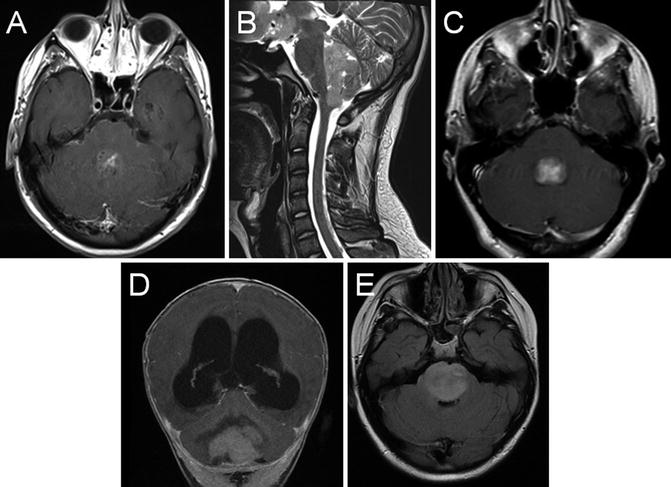
Fig. 22.8
Axial T1 post-gadolinium (a), and sagittal T2 (b) MRI showing a posterior fossa mass lesion with an enhancing component. Surgical resection revealed a medulloblastoma. Axial T1 post-gadolinium (c) shows a fourth ventricular ependymoma. Coronal T1 post-gadolinium (d) shows a cerebellar pilocytic astrocytoma with obstructive hydrocephalus. Axial FLAIR (e) shows a diffuse infiltrative pontine glioma
Classification and Grading
Unlike systemic cancer, staging is not undertaken for primary brain tumors, and spread of systemic malignancy to the CNS would qualify as stage IV disease. However, an extent of disease evaluation in known or suspected CNS malignancies such as CNS lymphoma or brain metastasis presenting when the status of systemic disease is unknown is important. Spine imaging in known or suspected leptomeningeal cancer, especially if there are signs or symptoms of nerve root involvement, is also appropriate.
CNS tumors are traditionally graded upon acquisition of tumor tissue based on histologic appearance. The World Health Organization (WHO) Classification of Central Nervous System Tumors is the most widely accepted histologic grading system [4]. It classifies tumors based on histopathologic and immunohistochemical features, and is revised periodically to reflect advances in the field based on expert consensus. In the WHO classification, grade I and II tumors are designated as low grade, and grade I tumors are considered potentially curable with surgery. Grade III and IV tumors are high grade, with more aggressive growth potential. They often require radiation and/or chemotherapy in addition to surgical resection.
In some tumors, better understanding of the genetic abnormalities in the tumor has led to advances in molecular phenotyping. The Cancer Genome Atlas (TCGA) project, for example, completed genomic sequencing of 206 glioblastomas [5]. TCGA provided new insights into the frequency of specific genetic abnormalities in glioblastoma. The information from TCGA led to a proposed classification scheme in which four distinct molecular phenotypes in glioblastoma were derived by consensus clustering based on abnormalities in platelet-derived growth factor receptor (PDGFR)-A, isocitrate dehydrogenase (IDH)-1, epidermal growth factor receptor (EGFR), and neurofibromatosis (NF)-1 [6].
The four molecular phenotypes are designated classical, mesenchymal, neural, and proneural. The classical glioblastoma subtype is characterized by chromosome 7 amplification paired with chromosome 10 loss. There was also a higher frequency of EGFR amplification, and less frequent TP53 mutation than other GBM subtypes. The mesenchymal subtype was characterized by focal deletions of a region of chromosome 17 that contains the NF1 gene, and high expression of genes in the tumor necrosis factor and NF-kappa B pathways. The neural subtype showed prominent expression of neural markers.
The proneural subtype demonstrated amplification and increased gene expression of PDFGRA and a higher frequency of point mutation in IDH-1 than other subtypes. Proneural classification was somewhat less common, associated with younger age at onset and a tendency toward better overall survival than other GBM subtypes. When the molecular phenotyping was expanded to include analysis of histologic grade II and III gliomas, a number of lower grade gliomas were found to have a proneural molecular signature. These were characterized by the coexistence of IDH1 mutation and CpG island methylator phenotype (CIMP). Codeletion of chromosomes 1p and 19q, long identified as a favorable prognostic feature in oligodendroglial tumors, was found in many grade II and III tumors as well. The combination of IDH-1 mutation/CIMP+ and 1p;19q codeleted molecular signature predicted longer survival when compared to IDH-1 wild-type/CIMP− and 1p;19q non-deleted tumors independent of tumor histology [7].
DNA repair is one mechanism of resistance to chemoradiation in high-grade gliomas. The most common chemotherapeutic agent for glioblastoma, temozolomide, is an alkylating agent with good CNS penetration that acts by methylation of guanine at the O-6 position. Methylation disrupts DNA replication and ultimately leads to apoptosis. The DNA repair enzyme methylguanine methyltransferase, or MGMT, reverses the methylation activity of temozolomide. High-grade gliomas that have a methylated (inactive) MGMT promoter show prolonged median overall survival and improved 2-year survival with concurrent radiation and temozolomide chemotherapy plus adjuvant monthly temozolomide. Tumors with an unmethylated (active) MGMT promoter region have no significant benefit from treatment with alkylating chemotherapy [8]. MGMT promoter methylation is associated with the CIMP phenotype found most commonly in the proneural glioma subtype. Strategies to inactivate the DNA repair activity of MGMT or administer a chemotherapy that is not dependent on DNA methylation are potential strategies to improve outcomes in individuals with MGMT unmethylated tumors.
A more detailed understanding of molecular phenotyping will continue to provide insight into differences in the biologic behavior among malignant glioma subtypes, as well as differences in survival and response to treatment with chemotherapy. Identifying important molecular pathways also provides potential targets for future therapies.
Advances in Diagnosis
Molecular Diagnostic Tools
As the field of cancer genomics provides new insight into specific genetic alterations that characterize CNS cancers, better tests are being devised that achieve less invasive and potentially earlier detection, improve diagnostic certainty, and will give information about prognosis, or one or more specific therapeutic targets.
Cerebrospinal fluid (CSF) analysis is a diagnostic test that, if successful, may provide information to guide treatment without requiring craniotomy. This approach would be especially important for mass lesions that are in areas that are high risk to biopsy. Historically, the main CSF diagnostic tools are CSF cytology, flow cytometry, and a handful of molecular tests including CSF lactate dehydrogenase, beta-2-microglobulin, and Epstein-Barr virus DNA by polymerase chain reaction (PCR). Unfortunately, all of these tests are limited by low sensitivity or specificity.
More recently, proteomics and micro-RNA analysis of cerebrospinal fluid has emerged with the potential to differentiate lymphoma from other CNS inflammatory disorders, and potentially improve the detection of solid tumor CSF spread as well (Fig. 22.9a–c). Compared to conventional cytology, which had a sensitivity of 17 %, elevated levels of proteins associated with B-cell proliferation and migration (interleukin-10 and chemokine CXCL-13) in combination were shown to be 54 % sensitive and 99 % specific in the detection of CNS lymphoma [9]. Elevations in a combination of three specific micro-RNA levels from CSF in individuals with primary CNS lymphoma were 95.7 % sensitive and 96.7 % specific compared to controls [10]. CSF micro-RNAs in the diagnosis of glioma have a 90 % sensitivity and 100 % specificity to identify glioma compared to controls [11]. Micro-RNA levels in CSF have been used to successfully differentiate cancer from nonneoplastic brain lesions, and in some cases to distinguish metastasis from lung cancer versus breast cancer [12]. In solid tumors, elevated levels of CSF vascular endothelial growth factor (VEGF) had 75 % sensitivity, 97 % specificity, and 94 % negative predictive value in the diagnosis of breast cancer leptomeningeal metastasis compared to CSF cytology [13].
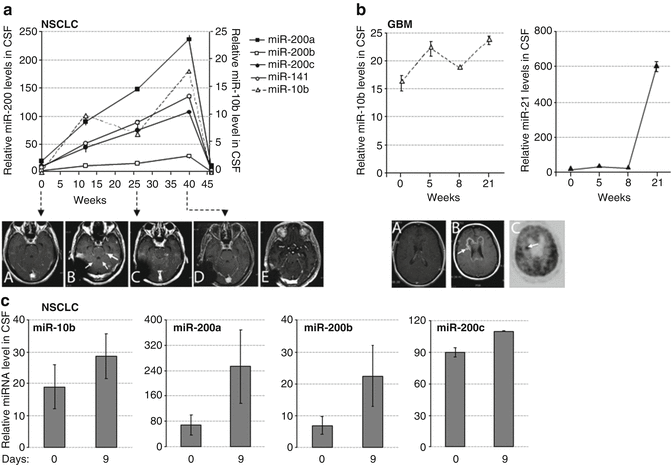
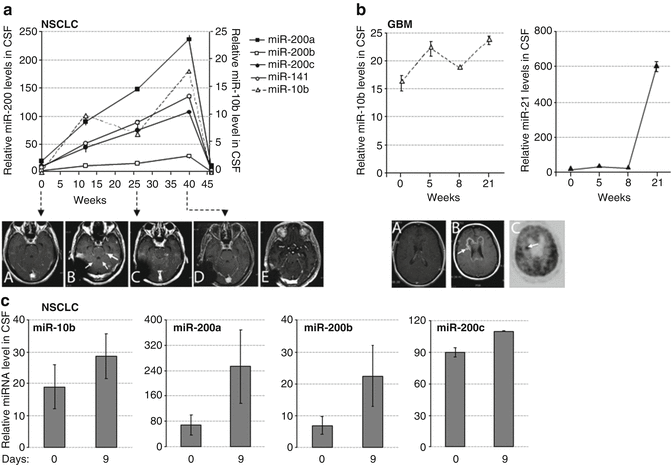
Fig. 22.9
The CSF levels of miRNA markers in metastatic lung cancer and GBM patients during treatment with erlotinib. miRNA levels were examined by qRT-PCR in CSF samples of lung cancer patients (a, c) and GBM patient (b) during the time course of erlotinib treatment. The disease progression and the drug response were concomitantly monitored by MRI, as follows. For Patient A: serial axial post-gadolinium MRIs of lung cancer patient’s brain during course of progression of disease and stability and improvement on MRI with escalating doses of erlotinib. (A) Time 0 weeks while patient on erlotinib, there is no leptomeningeal and parenchymal enhancement and CSF cytology was negative; (B) 3 weeks progression on erlotinib 150 mg daily dosing with new cerebellar leptomeningeal enhancement (small arrows) and nodule (large arrow), erlotinib increased to 600 mg every 4 days at 9 weeks; (C) 29 weeks on showing stable leptomeningeal enhancement and nodule; (D) 40 weeks showing reduction in leptomeningeal enhancement and nodule, erlotinib increased to 900 mg every 4 days at 41 weeks; (E) 64 weeks after six cycles of chemotherapy with carboplatinum and pemetrexed due to lung cancer progression showing further reduction in leptomeningeal enhancement and nodule has disappeared. For Patient B: (A) time 2 weeks for patient with recurrent GBM with prominent mass effect and enhancement felt to be radiation changes rather than tumor based on MRI spectroscopy and FDG-PET scan on erlotinib at 600 mg every 4 days; (B) 26 weeks on treatment showing progression on MRI with new lesion (arrow) concerning for tumor; (C) 27 weeks on treatment showing hypermetabolic area (arrow) on PET consistent with tumor and biopsy confirmed. For Patient C: Had inadequate treatment due to functional status and rapidly progressed over a few weeks, which was reflected by an increase in levels of miR-200 family members in a short interval. (All: Reproduced with permission from Teplyuk NM, Mollenhauer B, Gabriely G, Giese A, Kim E, Smolsky M et al. MicroRNAs in cerebrospinal fluid identify glioblastoma and metastatic brain cancers and reflect disease activity. Neuro-oncology 2012;14(6):689–700)
Circulating Tumor Cell Detection
Over the past 5–10 years, it has become possible to isolate circulating tumor cells (CTCs) in whole blood. Cells are obtained by using markers to detect epithelial cell adhesion molecules that are present on tumor cells, but not expressed on normal blood cells. The same technology used in whole blood may be used with CSF, and live tumor cells can be isolated this way [14, 15]. The clinical significance of asymptomatic CTCs in cerebrospinal fluid, and the reliability of following CTC counts as a marker of response to therapy as an adjunct or in lieu of cytology, remains to be determined. It is possible that CTC technology will allow considerably earlier detection of CNS involvement. Earlier detection and initiation of CNS-directed therapies may positively impact survival outcomes in brain and leptomeningeal metastasis.
Neuroimaging
Contrast-enhanced magnetic resonance imaging (MRI) is the diagnostic test of choice for identification and characterization of brain tumors (Fig. 22.1a, b through 22.8a–e, Figs. 22.10a–c, 22.11, 22.12a, b). Among other advantages, increases in field strength from 1.5 to 3 T scanners for clinical use have resulted in greater distinction between contrast-enhancing and nonenhancing regions, improved spatial resolution, and more clear differentiation of tissue types (spectral resolution). Perfusion-weighted imaging and assessment of relative cerebral blood volume to identify areas of hyperperfusion make it possible to more accurately discriminate between neoplastic and inflammatory brain lesions or subacute infarction (Fig. 22.12a, b).
