14 Botulinum Toxin Injections in Myofascial Pain Disorders
This chapter will discuss the uses of botulinum toxin for pain management in three of the myofascial pain disorders: cervical dystonia, myofascial pain syndrome and the piriformis syndrome. Although the uses for botulinum toxin that are licensed by the Food and Drug Administration (FDA) do not include pain management, it has been used by a range of medical specialists to address pain control of various etiologies. The purpose of this chapter is to provide clinical insights for clinicians regarding the use of botulinum toxin in three disorders that involve myofascial pain, based on the authors’ experience and literature review. As with all medications and procedures, clinicians must obtain the necessary training and knowledge to achieve the most effective and safe outcome. Accordingly, one should know essential features about this toxin before treating patients for pain. These vital elements include: mechanism of action1,2; concept of median lethal dose (LD50); dosing and administration3; basic neuromuscular anatomy and physiology; conjunctive therapy with botulinum toxin for optimal treatments;4,5 and contraindications, of using this toxin. Because it is beyond the scope of this chapter to address all of the topics just listed, the authors urge the reader to visit other informational sources listed at the end of this chapter.
Botulinum Toxin and Its Clinical Use
Justinus Kerner, a German physician and poet, provided the first accurate and complete description of the clinical symptoms of food-borne botulism. He described 230 patients in the 1820s who suffered all the muscular and autonomic symptoms including gastrointestinal disturbances, dry eyes, dry skin, and weakness associated with the ingestion of contaminated meats.6 Kerner termed this condition “sausage poison” and “fatty poison”. He went on to perform animal experiments and experiments on himself. He administered botulinum toxin extracted from contaminated sausages to birds, cats, rabbits, frogs, flies, locusts, and snails.7 Kerner developed hypotheses from his experiments on the pathophysiology of the toxin. In his monograph he stated: “The nerve conduction is brought by the toxin into a condition in which its influence on the chemical process of life is interrupted. The capacity of nerve conduction is interrupted by the toxin in the same way as in an electrical conductor by rust.” Later in the monograph, he conceives of the idea of using the toxin for therapeutic uses.7
The term botulism was derived from the Latin term, “botulus”, which means sausage. Edward Schantz first isolated the toxin and he and Alan Scott began work on a standardized botulinum toxin preparation in the late 1960s.6 Scott first used botulinum toxin type A in monkey experiments in 1973 and he first used botulinum toxin type A in humans to treat strabismus in 1980.6 Botulinum toxin type A was approved by the Food and Drug Administration (FDA) in 1989 for the treatment of strabismus, blepharospasm, and hemifacial spasm in patients more than 12 years of age. Botulinum toxin type B received FDA approval for treatment of cervical dystonia in 2000, and botulinum toxin type A was approved by the FDA to treat severe primary axillary hyperhydrosis and moderate-to-severe glabellar frown lines in 2002.
In addition to the approved uses in the United States, there are other published uses of botulinum toxin,8 which include painful or potentially painful conditions such as: achalasia; anismus (painful); bladder detrusor hyperactivity; essential tremor; myofascial pain syndrome (painful); focal dystonias (sometimes painful); muscle spasm (often painful); piriformis syndrome (painful); spasmodic dysphonia; spasticity (sometimes painful); whiplash (painful); chronic focal painful neuropathies; migraines and other headache disorders; temporomandibular joint pain disorders; gastrointestinal dysmotility disorders; chronic low back pain.
Aside from publications, noteworthy medical organizations have commented on the effectiveness and safety of botulinum toxin. The National Institutes of Health (NIH) Consensus Development Conference published a statement in 1990 that summarized the indications and contraindications of botulinum toxin usage for the treatment of a variety of conditions.9 The NIH conference endorsed the use of the neurotoxin as safe and effective for the symptomatic treatment of adductor spasmodic dysphonia, blepharospasm, cervical dystonia, hemifacial spasm, jaw-closing oromandibular dystonia, and strabismus. The same year, the Therapeutics and Technology Assessment Subcommittee of the American Academy of Neurology further endorsed the use of botulinum toxin for the symptomatic treatment of these conditions.10
Botulinum toxin is held to be one of the deadliest poisons known to mankind, yet by harnessing this activity, it has resulted in great advances in the treatment of numerous conditions.2 The understanding of the mechanism of action of botulinum toxin had its beginnings in 1949 when Burgen’s group discovered that botulinum toxin blocks neuromuscular transmission.11 In the 1950s, researchers discovered that injecting overactive muscles with small quantities of botulinum toxin type A resulted in decreased muscle activity by blocking the release of acetylcholine (Ach) at the neuromuscular junction.11 The understanding of this mechanism of action led to the application of botulinum toxin in most clinical settings. Since then, significant advances have occurred in the understanding of the mechanisms of action of botulinum toxin other than muscle paralysis—and this is leading to additional applications.
There are seven serotypes (A, B, C1, D, E, F and G) of botulinum toxin that act by inhibiting the exocytosis of Ach from presynaptic boutons of cholinergic neurons. Botulinum toxin is synthesized as a single-chain polypeptide (~150 kDa) that is activated by proteolytic cleavage into a 100-kDa heavy chain and 50-kDa light chain linked by a disulfide bond. The heavy chain domain is involved in cellular uptake into the presynaptic terminal by binding to extracellular receptors and in the transport of the neurotoxin through the lipid bilayer. The toxin is now intracellular but is sequestered in a membranous organelle without access to its targets, which reside in the cytoplasm. It is one of the functional domains (amino) of the heavy chain of the toxin that undergoes a conformational change facilitating translocation of the light chain through the lipid bilayer into the cytoplasm. Finally, the disulfide bond between the heavy and light chain is cleaved, allowing the light chain access to the cytoplasm. The light chain is a zinc-dependent endoprotease and the targets of the protease are presynaptic proteins required for intracellular trafficking of acetylcholine vesicles into the synaptic cleft. The presynaptic proteins targeted by the protease are called SNARE proteins (soluble N-ethylmaleimide-sensitive fusion protein attachment receptor) and are critical for the exocytosis of synaptic vesicles. It is this step, the proteolytic cleavage of the SNARE proteins, that is best understood in the intoxication by botulinum neurotoxin.12
The release of Ach into the synaptic cleft requires docking of the Ach-containing vesicle to the presynaptic membrane.12 The docking of the vesicle requires various proteins that are located in the wall of the vesicle, in the presynaptic membrane or in the cytoplasm. This family of proteins (SNAREs) includes vesicle-associated membrane protein (VAMP)/synaptobrevin, syntaxin, and SNAP-25. These proteins form a complex that allows the vesicle to fuse with the motor nerve terminus membrane and release the acetylcholine into the synaptic cleft. The site of action of botulinum toxin type A is SNAP-25 (synaptosome-associated protein with a molecular weight of 25 kDa), which is a presynaptic membrane protein. Botulinum toxin type B targets VAMP (vesicle-associated membrane protein), which is located in the wall of the vesicle. Cleavage of the proteins prevents the assembly of the fusion complex and thereby blocks the docking of the vesicle and the release of the Ach leading to relaxation of the muscle cell and a state of chemical denervation.12
Of significant clinical importance is the duration of action of the toxin effect. In general terms, the clinical effects of botulinum toxin injections are delayed a day or two with the maximal effects of functional muscular weakness peaking at about 2 weeks.1,5 The therapeutic effect of botulinum toxin-induced neuromuscular blockade usually lasts 3 to 4 months and ranges 2 to 6 months following an injection.13 Several factors likely contribute to the duration of action of the toxin. The best understood factor and most often quoted is the synaptic remodeling; however, other factors such as the duration of protease activity in the nerve terminal, the rate of replacement of the cleaved SNARE proteins, and the activity of the cleavage products may also influence the rate of function recovery.6
The development of neutralizing antibodies to botulinum toxin can be a therapeutic problem for patients and physicians.14 Patients who initially respond well to treatment with botulinum toxin can become nonresponders.15 The incidence rates of neutralizing antibody development and the exact cause in individual patients remains unknown.14 More frequent injections, more “booster injections,” and higher doses are possible risk factors, thus extending the time between injections, minimizing the dose, and avoiding booster injections may decrease the development of neutralizing antibodies.15
Some authors have noted that the pain relief preceded muscle decontraction and exceeded the degree and duration expected as a consequence of its neuromuscular actions.13 These observations suggest that botulinum toxin may have antinociceptive properties independent of the muscle relaxation. These observations and the expanding body of literature examining botulinum toxin for primary headache disorders and muscle conditions including a pain component led to further investigation into botulinum toxin in pain.16
A number of in vitro experiments have provided evidence that botulinum toxin inhibits neurogenic inflammation by attenuation of the release of neurotransmitters.17 Botulinum toxin was found to inhibit substance P release from cultured embryonic dorsal root ganglion neurons and to reduce stimulated release of calcitonin gene-related peptide from cultured trigeminal ganglia neurons.18
Additional support has come from animal experiments demonstrating reduction in nociceptive behaviors in animal models of inflammatory and traumatic neuropathic pain following peripheral injections of botulinum toxin.17 In rats with induced trigeminal neuropathy, intradermal injection of botulinum toxin in the area of the infraorbital branch of the trigeminal nerve alleviated the mechanical allodynia and reduced the exaggerated neurotransmitter release.19 In another experiment by Cui and colleagues, a rat formalin model of inflammatory pain was inhibited by subcutaneous administration of botulinum toxin injection and this inhibition was associated with a reduction in neurotransmitter release from the peripheral terminals of nociceptive sensory neurons. 20 It was Cui’s study in 2002 that provided the first evidence that botulinum toxin had an effect on nociceptive sensory nerves in vivo.20 In studies by Aoki, botulinum toxin inhibited several of the neurophysiologic and neurochemical effects of formalin in the rat formalin-pain model including glutamate release, Fos-LI in the dorsal horn, and evoked-activity of WDR neurons in the spinal cord.16
The efficacy of botulinum toxin in neuropathic pain initially was suggested in small anecdotal case studies and small open-label trials.21 An open study of botulinum toxin in 13 volunteers with trigeminal neuralgia found a reduction in visual analog scale scores and surface area of pain.22 Tsai and coworkers conducted an open label, prospective pilot study using botulinum toxin injected intracarpally in five patients with primary carpal tunnel syndrome.23 Their data suggested a long-acting antinociceptive effect of botulinum toxin.
More recently, two well designed clinical trials evaluated the efficacy of botulinum toxin for chronic neuropathic pain and diabetic neuropathic pain.17,24 Ranoux and colleagues published a randomized, double-blind, placebo-controlled, parallel group study providing evidence in support of the efficacy of botulinum toxin for the pain associated with focal neuropathies such as postherpetic neuralgia and posttraumatic or postoperative neuropathy.17 The study included 29 patients with focal painful neuropathies and mechanical allodynia, and treatment consisted of a one-time intradermal administration of botulinum toxin into the painful area. Outcome measures included average spontaneous pain intensity, quantified testing of thermal and mechanical perception of pain, allodynia to brush and decreased pain threshold to cold; the measures were evaluated at baseline, 4, 12, and 24 weeks. The results indicated that botulinum toxin treatment, relative to placebo, was associated with persistent effects on spontaneous pain intensity from 2 weeks after the injection to 14 weeks.12 A recent study by Yuan and associates report the results of a double-blind, placebo-controlled, crossover trial of intradermal botulinum toxin for diabetic neuropathic pain in 18 patients.24 The authors found a significant reduction in visual analog scale of pain at 1, 4, 8, and 12 weeks after botulinum toxin injection when compared to the placebo group. Specifically, within the botulinum toxin group, 44.4% of the participants experienced a reduction of the visual analog scale greater than or equal to 3 within 3 months after the injection, in contrast to the placebo group that reported no similar response. In addition, the authors evaluated sleep quality using the Chinese version of the Pittsburgh Sleep Quality Index and found a difference in the improvement in sleep quality between the botulinum toxin treatment group and the placebo group. The difference between the groups reached significance (P < .05) only 4 weeks after the initial injection, but did not support sleep improvement with botulinum toxin at week 12, which was the endpoint of the study design.24
Both reports (Yuan and colleagues24 and Ranoux and associates17) are small studies but each support a trend of reduced pain perception beginning at 1 week postinjection and extending to 12 weeks in the Yuan and colleagues’ study and 14 weeks in the Ranoux and associates’ study.17,24 Both studies report essentially no adverse events and this is supported by the general botulinum toxin literature. Although this suggests a promising approach to the treatment of neuropathy, additional larger, well designed, multicenter clinical trials with longer periods of follow-up are necessary.
Cervical Dystonia
Cervical dystonia (CD), also known as spasmodic torticollis and torsion dystonia, is a common form of focal dystonia manifesting as involuntary contraction and twisting of the neck muscles.25,26 These features lead to abnormal postures and movements of the head. The deviation of the head can be multidirectional and is described as torticollis (the most common form of CD with patient’s head turned to one side); laterocollis (lateral flexion of the neck), anterocollis (flexion of the neck) and retrocollis (extension of the neck). It is possible that one can have a combination of these forms. The prevalence of CD was reported to be 8.9/1,000,00027 and it is recently estimated by the Dystonia Medical Research Foundation that 250,000 people suffer from CD in the United States. It is believed that 66% to 75% of the patients with CD are disabled from the pain associated with CD.27–30 CD is mostly idiopathic and about 12% of those affected have a family history.31 Idiopathic CD is the most common form of adult-onset focal dystonia slowly developed over several years in patients 30 to 50 years old.32 Cervical dystonia can be caused by any injury or inflammation of the cervical muscles or cranial nerves from various disease processes, including head, neck, and shoulder trauma33 or from taking dopaminergic block agents.34 There is evidence indicating that CD arises from basal ganglia circuit abnormalities leading to dopaminergic dysfunction, which in turn causes disinhibited thalamocortical output and dystonic postures.31,35–37
Patients with CD can have a wide spectrum of symptoms involving the head, neck, upper extremity, and other body parts with sustained painful muscle contraction, pulling, and/or stiffness.28,31,37 The severity of the pain is usually relative to the intensity of the dystonia and muscle spasms.28 Jahanshahi and colleagues reported progression of dystonic symptoms to extranuchal, but still cervical, innervated sites such as hand, arm, and oromandibular region in one third of the 72 patients with adult-onset cervical dystonia.38 Patients with CD may develop neck pain from the muscle contraction and muscle strain from correcting the abnormal posture. The chronic abnormal posture may also lead to degenerative changes in the cervical spine leading to facet pain, radiculopathy, or spinal stenosis. Headaches associated with CD are common.39 Acute posttraumatic CD is different and the symptoms include immediate local pain after trauma, followed by a significant limitation in cervical range of motion, and abnormal posture of the head and shoulder. Trapezius hypertrophy may occur as well. Those changes often result in abnormal muscle contraction and pain.28,31 The abnormal posture and pain in patients with CD are part of their functional limitation and interfere with the patients’ activities of daily living.31 Rondot and coworkers40 found that 99% of the 220 patients they studies had various functional difficulties. Dysphasia and subclinical swallowing motility disturbances were reported in those patients.41 Permanent disability from the decreased cervical range of motion, involuntary movements, and intractable pain may occur.42 The diagnosis of CD is clinical and inspection is usually enough. However, a though physical examination should be conducted to rule out “pseudodystonia” due to structural abnormalities43 and secondary dystonia.
Treatment of Cervical Dystonia with Botulinum Toxin
There are various treatment options for CD including medications,32,44–47 surgery,48–56 rehabilitation,57 deep brain stimulation,58,59 and injections. The goal of the treatments is to reduce the discomfort and pain; improve function and quality of life; and prevent complications. Other than botulinum toxin (BTX) injection, the other treatment modalities are beyond the scope of this chapter.
The care of CD patients was drastically improved since 1989 when botulinum toxin was introduced in the United States. The FDA approved the indication of botulinum toxin injection for CD treatment in 2000. It is generally considered that BTX injection is the treatment of choice for patients with CD.60–62 The effectiveness of local injections of botulinum toxin is supported by evidence-based reviews and meta-analysis.45,54,63,64 Jankovic and Schwartz65 followed 202 out of 232 patients who received botulinum toxin type A injection for medically intractable CD for at least 3 months and up to 4 years. Seventy one percent of those patients had improved symptoms and 76% had almost complete relief of pain. Hsiung and colleagues published that 63% of patients treated with botulinum toxin injection reported benefit at 5 years.66 In a 20-week multicenter double-blind, randomized, controlled study in the United States involving 80 patients, the botulinum toxin group demonstrated improvement in the total Toronto Western Spasmodic Torticollis Rating Scale (TWSTRS) score than did the placebo group at weeks 4, 8, and 12 weeks.67 The side effects of the two groups were similar except for blurred vision and weakness in the botulinum group. The Therapeutics and Technology Assessment Subcommittee of the American Academy of Neurology concluded that botulinum toxin is safe and effective for the treatment of CD.68 There are numerous studies using botulinum toxin type B for CD treatments.69–71 Pappert and Germanson compared the CD treatment with botulinum toxin type A and botulinum toxin type B in a randomized, double-blind study, and found that both types of botulinum toxin are effective and safe for the treatment of CD in botulinum toxin-naïve patients.72 There is disagreement regarding the study conclusion.73,74 In a Cochrane Reviews, Costa and associates concluded that single injections of botulinum toxin type A and type B are effective and safe for treating CD, and long-term, uncontrolled studies suggested that further injection cycles continue to work for most patients.63,75 In another Cochrane Review, these authors concluded that it was not possible to make a definitive comparison between botulinum toxin type A and type B for CD treatment.76
Because of the complexity of the neck, it is critical for the clinician to be familiar with the anatomic landmarks, the vital structure, and the muscles for a successful treatment of CD with botulinum toxin injection. Attention should be paid to the following structures/organs when injecting botulinum toxin: the brachial plexus, carotid sheath, pharynx, esophagus, and the apex of the lung. The muscles involved in various forms of CD are listed in Table 14-1. Although the use of electromyography (EMG) for botulinum toxin injection for CD management remains controversial,68 the authors recommend using EMG-guided injection whenever possible to ensure the effective targeting of the affected muscles77 and the motor end plates within those muscles78–80 to potentiate neurotoxin effects. It is essential to have the patients as relaxed as possible to eliminate the false motor action potentials on EMG. If palpation alone is used to identify affected muscles, injection into either the mid-belly or several sites of the muscle is generally recommended.
Table 14-1 Typical Muscles Involved in Various Types of CD
CD Type | Muscles Involved |
---|---|
Torticollis | |
Laterocollis | |
Retrocollis | |
Anterocollis | |
Shoulder elevation |
CD, Cervical dystonia.
Adapted from Brashear A: Botulinum toxin type A in the treatment of patients with cervical dystonia. Biologics 3:1-7, 2009.
Because of the variation of muscle involvement, there are no clear guidelines for the appropriate dose of botulinum toxin for the treatment of CD. A wide range of dosages was reported in CD treatments with botulinum toxin type A81–90 and type B.69–71 Dressler found that 20 units of botulinum toxin type A reduced the dystonic activity in the sternocleidomastoid muscle and larger doses offered minimal additional effect.91 Koller and colleagues92 noted that fixed-dose, fixed-muscle controlled studies of botulinum toxin for the clinical management of CD did not produce the same effects. Based on our experiences and the literature review, we think that, in general, patients should receive as few doses of botulinum toxin as possible as long as their symptoms are managed; the dose of botulinum toxin should be individualized; and the lowest effective dosage of botulinum toxin should be administered for treatment of CD to protect the patient from becoming immune to its therapeutic effect.93,94
Myofascial Pain Syndrome
Myofascial pain syndrome (MPS) is a painful disorder that is characterized by the presence of the palpable taut band of muscle fibers and myofascial trigger points (MTrP).95 The International Association for the Study of Pain defines the trigger point as a discrete tender point palpable in the taut muscle band.96 A myofascial pain syndrome caused by MTrPs characteristically results from either an acute episode of muscle overload or a chronic and/or repetitive muscle overload.97 In addition, there are autonomic phenomena (piloerection, localized sweating, or even regional temperature changes in the skin because of altered blood flow), concomitant disorders, psychosocial factors, and psychiatric presentations including depression and anxiety.98,99 There are different opinions about what MPS represents. Some scholars think that it should cover all the regional pain caused by any soft tissue pathology. Thus, MPS may be considered as a primary local or original pain syndrome or a secondary syndrome caused by other disorders.100,101
Research studies indicate that the clinical characteristics of an MTrP can be explained by hypercontracted muscle fibers located at and produced by a region of muscle with multiple dysfunctional motor end plates (neuromuscular junctions). The dysfunction is a markedly excessive continuous release of the normal synaptic transmitter, Ach. The noise-like potentials and spikes that are strongly associated with MTrPs102–104 were first interpreted as coming from muscle spindles.103 However, electromyographic studies clearly identify these noise-like and spike potentials as motor endplate potentials of skeletal muscle fibers.105 Electromyographers generally recognize these end-plate potentials as normal.105–108 However, physiologists have distinguished these potentials from normal miniature end-plate potentials and have shown that they represent a pathologic increase in spontaneous release of Ach.109–112 The end-plate noise component can result from mechanical strain of the neuromuscular junction caused by stresses applied to the nerve terminal112 or produced by muscle overload. The end-plate noise component of end-plate potentials appears to be present before the needle examination and is commonly caused by stressful activity of the muscle, especially in latent MTrPs that cause no clinical pain complaint. The end-plate spikes, however, are often induced by the presence of the needle113 and are more likely to appear in more active MTrPs.
Histologically, MTrPs show large, darkly stained, round myofibers in cross section in canine,114 equine115 and in human116,117 studies. Longitudinal sections of myofibers several hundred microns in length of canine muscle show hypercontracted fibers (also called “contraction knots”). The integrated hypothesis for the pathophysiology of MTrPs attributes these contraction knots to the observed depolarization of the post-junctional membrane that continuously releases calcium from the sarcoplasmic reticulum. This hypothesis identifies contraction knots as limiting circulation because the strong contraction of the sarcomeres is sustained within the hypercontracted fiber, whereas local energy consumption is increased. The resulting energy crisis should exhibit severe local hypoxia, demonstrated in the MTrPs.118 Increased tension of involved muscle fibers accounts for the palpable taut band consistently associated with an MTrP. The energy crisis and local hypoxia that was observed to extend for several millimeters could account for the release of substances that sensitize local nociceptors, causing the local and referred pain characteristic of MTrPs.97 An interesting study examined rabbit muscle after a marker (iron deposit) was placed at precisely the location where an active trigger point was identified by twitch response, taut band, and spontaneous electrical activity. Small C nerve fibers (most likely nerves that carry pain information) were found in the immediate vicinity.107 Taken together, these data support the idea that MTrPs are related to abnormal motor end-plate activity and subsequent hypercontraction of the associated myofibers.119
MTrPs are identified on physical examination by palpating a localized tender spot in a nodular portion of a taut band of muscle fibers. Pressure (usually with the examiner’s fingertip) over a trigger point elicits pain at that area and may also elicit pain at a distance from the point under the fingertip. This is known as referred pain. Another important feature of the trigger point is that the elicited pain mirrors the patient’s experience. Insertion of a needle, snapping palpation, or even a brisk tap with the fingertip directly over the MTrP may elicit a brief muscle contraction detectable by the examiner. This brisk contraction of muscle fibers of the taut band is termed a local twitch response.97 There is a burst of electrical activity within the muscle band that has the twitch response (end-plate noise), and such activity is not observed in the other muscle bands. This type of end-plate noise is found more prevalently in myofascial trigger points than in sites that are outside the MTrP but still within the end-plate zone.120 Animal studies102,121,122 and a human study123 have shown that this response is propagated as a spinal reflex. This response is a valuable indicator that the needle being injected into an MTrP has effectively reached at least one necessary target in the MTrP. Demonstration of a local twitch is additional confirmation of the diagnosis. In addition, passive stretch range of motion of the muscle is limited by pain, and both maximum contraction in the shortened position and maximum voluntary contraction are likely to be inhibited or to be associated with pain.
If abnormal end-plate activity is responsible for MTrPs, then a powerful rationale exists for the use of neuromuscular blocking agents, such as botulinum toxin, in the treatment of myofascial pain syndrome.124 Injection of muscles with botulinum toxin can be appropriate therapy for myofascial pain caused by MTrP but is unlikely to be so for treatment of myofascial pain of unspecified origin, when that term is used in the general sense.125 Myofascial pain with muscle tenderness versus specific MTrPs responsible for the clinical pain is an ambiguous diagnosis. The tender spots may be due to fibromyalgia, bursitis, or one of many other diagnoses that do not justify injection with this product.
At present, no routine laboratory test or routine imaging test is available to confirm the presence of MTrPs. Usually two objective tests can be used to confirm the presence of MTrPs. One requires electrodiagnostic technique and the other uses ultrasound imaging. Both animal and human research studies have shown that MTrPs are characterized by electrically active loci that exhibit end-plate noise and often spikes.107
Treatment of Myofascial Pain Syndrome with Botulinum Toxin
The previous chapter in the second edition included a Medline search conducted in 1997 for the headings “botulinum toxin,” “myofascial pain,” and “pain” for the period 1966 to September 1997 and resulted in 18 references.126 An updated Medline search for the period September 1997 to April 2009 was conducted using the headings “botulinum toxin” and “myofascial pain syndrome” and resulted in 45 references. Of these, 21 studies were in English and included “pain” or “myofascial pain” within the article title.127–147
A few words of caution before considering using botulinum toxin in the treatment of a patient with myofascial pain: Recall that the approved indications for the use of botulinum toxin in the United States are for six conditions: strabismus, blepharospasm, hemifacial spasm, cervical dystonia, primary axillary hyperhidrosis, and moderate-to-severe glabellar frown lines.6 Use of botulinum toxin for myofascial pain is off-label and should be considered only for patients with conditions that failed conservative treatment or for patients judged inappropriate for conservative treatment.145
Two studies were designed to compare trigger point injections in the management of patients with myofascial pain and headaches.127,135 The studies included a total of 74 patients that were randomly assigned to one of three groups: dry needling, lidocaine injection, or botulinum toxin injection. Venancio and colleagues assessed their subjects during a 12-week period based on levels of pain intensity, frequency and duration, local post-injection sensitivity, obtainment time and duration of relief, and the use of rescue medication.127 In contrast, Kamanli and coworkers’ assessment measures included cervical range of motion, trigger point pain pressure threshold, pain scores, visual analog scales for pain, fatigue, and work disability at entry and at 4 weeks.135
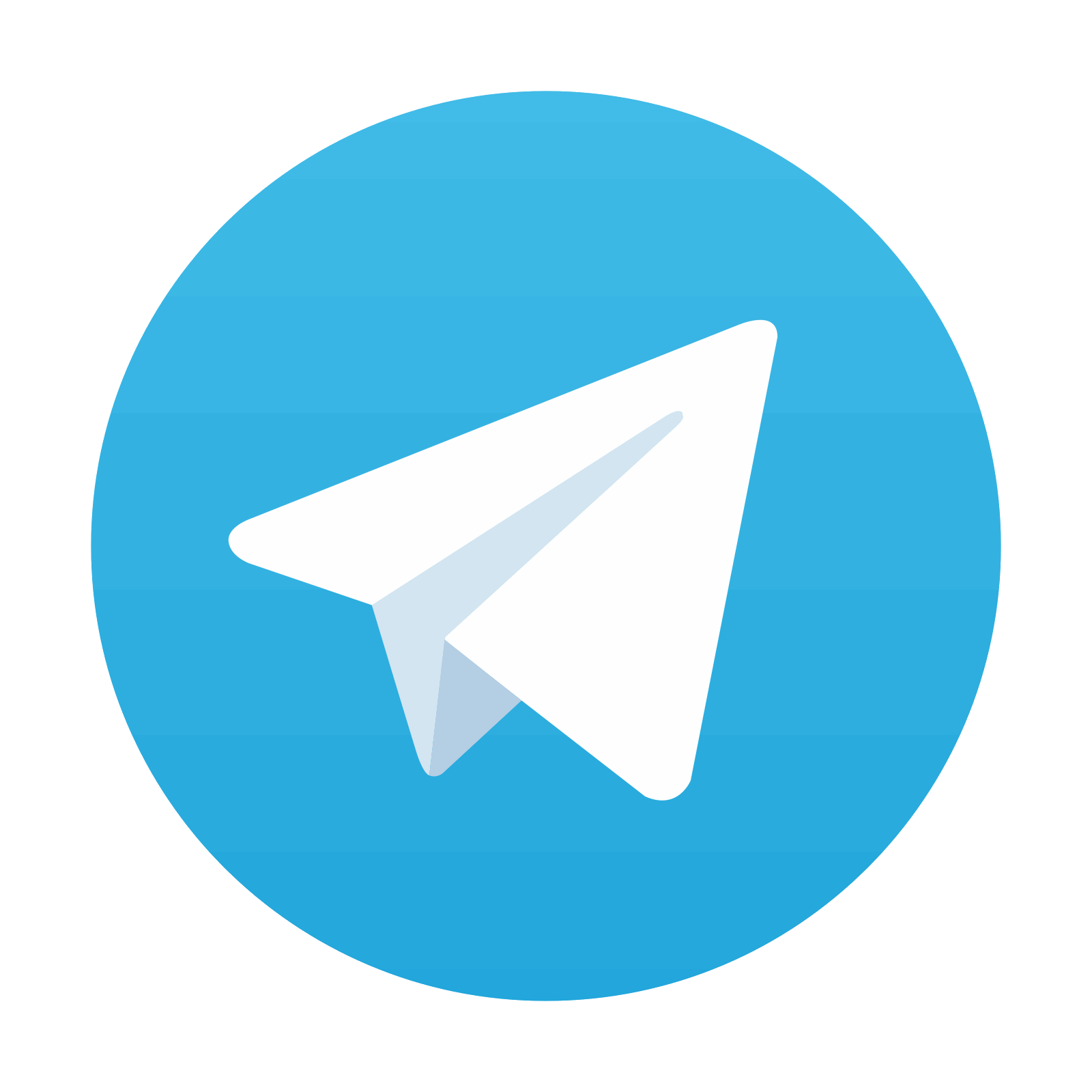
Stay updated, free articles. Join our Telegram channel

Full access? Get Clinical Tree
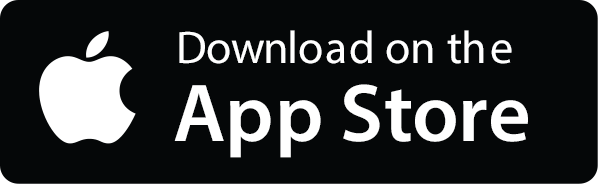
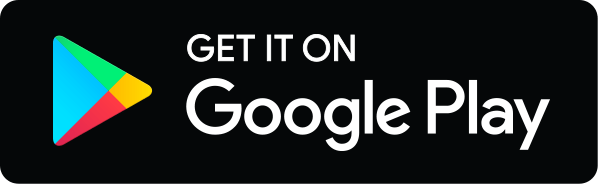