Abstract
The blood–brain barrier (BBB) provides a complex network of cells and supporting structures that maintain separation of brain parenchyma and cellular material. This chapter discusses the mechanism and functionality of the BBB and the effects of general anesthetics on BBB function. BBB permeability may be increased or decreased by anesthetics, inflammatory mediators and secondary messengers. Irrespective of mechanisms, altered BBB permeability leads to a number of homeostatic adaptations that may in turn translate into poor neurologic postoperative outcomes. Importantly, there are also some anesthetics with possible “antiinflammatory effects” that lead to BBB “tightening.” These may be neuroprotective. We will present and discuss this dual role of anesthetics on the BBB. In addition, we will provide a succinct description of the molecular changes to endothelial cells, as well as changes in inflammatory mediators that are responsible for the effects of anesthesia toward clinical or experimental end points.
Keywords
Blood–brain barrier, Clinical anesthesia, Inflammation, Neuroprotection, Physiology
Outline
Introduction 51
Permeability at the Blood–Brain Barrier 51
Cellular and Molecular Effects of Anesthetics on the Blood–Brain Barrier 52
Anesthesia and Nitric Oxide Signaling 52
Anesthesia Effects on Tight Junctions 52
Anesthesia Effects on Endothelial Cells 53
Anesthesia and Neuroinflammation 53
Clinical and Experimental Implications of Anesthetics on the Blood–Brain Barrier 54
Anesthetic Neuroprotection in Perioperative Neurological Injury 54
Antiinflammatory Effects of General Anesthetics in the Treatment of Refractory Status Epilepticus 55
Antiinflammatory Considerations in Barbiturate Induced Coma for Traumatic Brain Injury 55
Conclusion 56
References 56
Introduction
The blood–brain barrier (BBB) maintains the brain parenchyma and blood components in separate compartments. In addition, by allowing glucose transport it helps fuel neuronal function. Maintenance of the integrity of this closed compartment comprises a dynamic combination of vascular, cellular, molecular, and ionic factors. Structurally, this barrier is composed of endothelial cells supported mainly by astrocytes and pericytes. BBB endothelial cells also have a transport function that acts to maintain a constant parenchymal milieu. Endothelial cells transport amino acids, participate to ionic homeostasis, and allow a controlled exchange of solute and water. Importantly, a variety of traumatic and nontraumatic inflammatory insults to the BBB may lead to a loss of the closed compartment and consequences of such.
The anesthesiologist must be aware that interventions such as cardiopulmonary bypass, cerebral arteriography, and osmotic BBB opening have all been linked to impairment of cerebral homeostasis in patients. We will focus our text to the effects of anesthetics on the BBB and clinical implications of the same.
Permeability at the Blood–Brain Barrier
Structurally, the brain microvasculature is lined with endothelial cells that are secured together by tight junctions (TJs). These TJs provide a means to regulate movement of substances into and out of the brain. The lipid bilayer of these endothelial cells allows movement and determines permeability across the BBB. Substances do not cross through the alternative paracellular route.
Translated into clinical practice, this unique structure means that the BBB does not allow a majority of CNS drugs to enter the brain parenchyma. Interestingly anesthetics are an important exception in that they freely exert CNS effects, and it has been proven that the lipophilicity of these agents drives them to cross the BBB. The log octanol/water partition coefficient has a significant role in predicting how and if compounds will cross the BBB.
Typically this coefficient is determined using an aqueous substance (water) and a hydrophobic substance (octanol). Compounds with a high log (P) favor hydrophobic compartments and will cross a lipid bilayer while compounds with a low log (P) will tend to stay in hydrophilic compartments (e.g., serum) and will not cross the BBB. As a general rule, compounds with a log (P) > 0 will cross the BBB rapidly with the major limiting factor being supply of the drug. On the other hand, compounds with a log (P) < −1 are limited in their ability to cross the BBB. Importantly, log (P) is a velocity, and therefore a higher value is necessary for a clinically relevant effect. It is indeed in actuality a pharmacokinetic property that allows anesthetic agents to move from blood to brain.
Also, compounds with similar log (P)s can have differing ability to cross the BBB. To cross the BBB efficiently, a number of conditions must be met: (1) Larger compounds (greater than 400 Da) require some additional transport mechanism to cross the BBB. These are too large to pass through TJs or directly across the lipid bilayer. (2) Many drugs have changes in ionization states that affect the ability of a drug to cross the BBB. (3) Hyperthermia enhances while hypothermia impedes BBB permeability. (4) Highly regulated mechanisms of transport by BBB endothelial cells. This type of facilitated transport is dependent on membrane receptors and is the major mechanism of transport regulation across the BBB (most notably glucose).
Cellular and Molecular Effects of Anesthetics on the Blood–Brain Barrier
Anesthetic agents may interact in several different ways with the BBB. This is because the same anesthetic agent establishes a different relationship with the target, signaling pathway, and pathology involved. Both direct and indirect effects of anesthetic agents on the molecular components of BBB integrity may affect these signaling pathways. At the cellular level several changes may be important such as tight and adherens junctions, vasodilation, endothelial cell survival, and neuroinflammation. Therefore, anesthetic action at the BBB depends upon the extent to which the agent modulates these pathways of signal transmission and in addition the systemic cerebral milieu in which it is administered.
Anesthesia and Nitric Oxide Signaling
Anesthetics have a varied effect on nitric oxide (NO) signaling pathways. NO is a signaling molecule and potent vasodilator that has been effective in ischemic preconditioning and, at low levels, shown to induce neuroprotection mediated by the BBB. NO has important downstream effects such as vascular regulation, mediation of neuroinflammation, and regulation of endothelial cell survival.
Volatile anesthetics result in potent cerebral vasodilation that in turn can induce endothelial stretching, increasing the gap between neighboring endothelial cells, and hence BBB permeability. These agents act in part via modulation of NO signaling (via nitric oxide synthase iNOS) and regulation of ATP-sensitive K + channels in vascular smooth muscle cells.
Let us look at isoflurane as a specific example. Isoflurane can induce nitric oxide synthase (iNOS)-dependent cerebral vasodilation and also neuroprotection via NO downstream effects. But as both the agents, on the flip side, NO and isoflurane are known to stimulate prostanoid production, this may potentially result in increased inflammation. Some other studies have also noted the proapoptotic effects of NO donors in vitro that lead to disruption of the cerebral endothelial cell monolayer and increased leukocyte adhesion in the presence of NO. Some of these effects may be attenuated by propofol administration.
Volatile anesthetics are important regulators of cardiovascular output, vascular tone, and cerebral blood flow. This may be a mechanism of BBB perturbation (via endothelial stretching) and a potential experimental confounder when assessing other molecular mechanisms influencing permeability.
Anesthesia Effects on Tight Junctions
Animal models have shown isoflurane results in an increased edema in comparison to other anesthetics such as sevoflurane or pentobarbital. While vasodilation-induced cell starching and separation may play a role, other mechanisms are important in determining the direct effects on TJs. TJs are redistributed following ischemia, inflammation, and TBI. Sevoflurane compared to isoflurane, may alter the balance between TJ proteins that are modulated by anesthetics specifically by greater induction of zonula occludens-1 (ZO-1) as compared to occludin. This coincides with a reduction in cerebral edema in sevoflurane-treated animals. The other important TJ protein, occludin, is regulated in part by glutamate signaling, which is altered by certain anesthetics. Glutamatergic signaling alters the phosphorylation of certain occludin residues, resulting in its redistribution and a consequent increase in BBB permeability. The clinically important implication for this mechanism is the reversal of this increased permeability via NMDA antagonists including the commonly used ketamine. An increase in circulating amino acids levels results in elevated plasma and cerebrospinal fluid (CSF) glutamate. This presents another possible mechanism by which anesthetic administration may modulate BBB TJs via glutamatergic signaling. As a direct effect, isoflurane downregulates the expression of occludin in human brain vascular endothelial cell cultures in a hypoxia inducible factor-1α (HIF-1α)–dependent manner.
In addition to its role in occludin expression, HIF-1α acts in biphasic manner with BB integrity to both protect against and exacerbate damaging pathways of cerebral ischemia and consequently plays a significant role in mediating cerebral water content. Propofol and isoflurane have both been implicated in modulation of this signaling pathway, which regulates expression of vascular endothelial growth factor, aquaporin-1, aquaporin-4, and matrix metalloprotease expression, among many others. Importantly, for the traumatized brain, HIF-1α has a biphasic expression, with a trough at 24 h postreperfusion that corresponds to a decrease in BBB permeability. While the protective effects of early HIF-1α inhibition with respect to increased BBB integrity have been confirmed by several studies, biological reality is likely to be far more complex. The reader is referred to the bibliography to find more reading of the HIF pathways, which is beyond the scope of inclusion in this text.
Anesthesia Effects on Endothelial Cells
Endothelial cell survival, structure, and expression of adherens or TJ proteins represent another set of pathways by which anesthetics, particularly isoflurane and propofol, alter the integrity of the BBB. In addition to its aforementioned roles, isoflurane posttreatment upregulates the activity of sphingosine kinase 1 (SphK1), which catalyzes production of sphingosine 1 phosphate (S1P). One such downstream signaling receptor of S1P is S1P1, signaling of which has been shown to decrease BBB permeability by inducing changes in expression of junction proteins such as VE-cadherins and altering cytoskeletal structure that decreases space between neighboring endothelial cells via GTPases Rho/Rac. Endothelial cell proliferation and migration is mediated via another such S1P receptor, S1P3. Furthermore, S1P3 alters calcium signaling and induces vasoconstriction in vascular smooth muscle, while S1P1 activation upregulates endothelial nitric oxide synthase activity, resulting in vasodilation. Isoflurane activates the antiapoptotic Akt pathway, increasing endothelial cell survival and improving cerebral edema via SphK1. The long-term protective effects of isoflurane postconditioning appear to depend upon the model of cerebral injury (e.g., hemorrhagic versus ischemic stroke), outcomes measured, and anesthetic protocol. This reemphasizes that clinical context remains of paramount importance in that the effects of anesthetics such as isoflurane in different experimental neurological pathologies are different, where the mechanism of early brain injury may be similar, but the sequelae distinct.
Anesthesia and Neuroinflammation
Neuroinflammation plays a significant role in the damage that follows a cerebral insult by mediating acute increases in permeability leading to delayed cell death. The role of inflammation via NFκB, IL-1β, TNF-α, and other reactive oxygen species in oxidative stress, endothelial dysfunction, and apoptosis has been described in many studies with potential clinical implications including cerebral edema, neuronal death, and short- and long-term cognitive impairment. Significantly, anesthesia has mostly antiinflammatory or rather minimal proinflammatory effects in healthy animals. Pretreatment or postconditioning with isoflurane reduces expression of the proinflammatory cytokines NFκB, IL-1β, and IL-6, while ketamine consistently demonstrates similar antiinflammatory properties, decreasing expression of NFκB, COX-2, iNOS, TNF-α, IL-1β, and other important inflammatory signaling molecules. Propofol exhibits similar antiinflammatory effects in endothelial cells in vitro through inhibition of NFκB, iNOS, and IL-1β. Extending beyond endothelial cells, astrocytes (an integral part of the BBB) exposed to midazolam and corticosterone challenge produced pregnenolone and progesterone, both of which are cytoprotective. Clinical effects of delayed cognitive dysfunction that are not immediately evident during acute-phase reactions may be secondary to the role of neuroinflammation acting in conjunction with delayed apoptotic signaling. This also may translate into timed administration of specific anesthetics that may protect the BBB and thus reduce cerebral edema and neuronal death in common inflammatory states such as infection, malignancies, etc.
Accumulating evidence of the effect of surgery on neuroinflammation and neurodegeneration suggests a potentially important role in anesthetic selection for vulnerable populations such as children, the elderly, and those with preexisting neurologic conditions such as Alzheimer disease. Induction of neuroinflammation by anesthetics is of particular concern in pediatric populations due to the documented inhibitory effect of proinflammatory cytokines on long-term potentiation. Indeed, consistent administration of sevoflurane or isoflurane to young mice results in cognitive impairment, although the causal mechanisms have yet to be fully elucidated. In young mice, sevoflurane administration upregulates the production inflammatory cytokines IL-6 and TNF-α, possibly as a downstream result of NFκB induction. Moderate induction of neuroinflammation by sevoflurane may have a particularly significant effect during periods of neurodevelopment due to the documented inhibitory effect of proinflammatory cytokines on long-term potentiation. This would support the clinical findings that repeated exposures to anesthesia during childhood can raise the risk for cognitive impairment as well as experimental results identifying neurodegeneration in neonatal rats exposed to isoflurane. In light of the evidence in favor of an antiinflammatory role of volatile anesthetics under pathologic conditions, it is unclear whether this is related to an age-dependent difference or to experimental variability in models (e.g., TBI vs healthy animals) or dosage protocol. The much talked about general anesthesia compared to spinal anesthesia (GAS trial) will examine outcomes at 2 and 5 years of age after exposure of the infant brain to volatile anesthesia. This will help establish whether general anesthesia in infancy has any effect on neurodevelopmental outcome. Outcomes at 2 years of age were accessed using the Bayley Scales of Infant and Toddler Development III during this multicenter, randomized controlled trial. These outcomes that have since been published found no evidence that just less than 1 h of sevoflurane anesthesia in infancy increases the risk of adverse neurodevelopmental outcome at 2 years of age compared with awake-regional anesthesia.
In summary, anesthetic agents differentially regulate several pathways directly and indirectly involved in BBB integrity including TJ formation, endothelial cell survival, vasodilation, reactive oxygen species production and signaling, and neuroinflammation. The varied effects of volatile anesthetics on inflammation and permeability underscore the potential role of variability in administration protocols and the presence and type of underlying neuropathology. These are important factors to account for in interpreting the above highlighted data by the bedside clinician. It is safe to conclude at this moment of time that the bulk of available data suggest a possible role for anesthetic agents in pre- or postconditioning of the BBB, though some other studies have also identified potentially detrimental effects including increased BBB permeability and neuroinflammation.
Clinical and Experimental Implications of Anesthetics on the Blood–Brain Barrier
The proceeding text has shown us that the interpretation of clinical and animal research to the operating room, and intensive care units is guarded to say the least. The clinician would specifically like to know if anesthetic neuroprotection exists in common clinical scenarios.
The following section highlights some such common clinical scenarios, such as perioperative neurological injury in cardiac surgery, the use of general anesthetics to treat refractory status epilepticus (SE), and high-dose barbiturate therapy to treat refractory intracranial hypertension.
Anesthetic Neuroprotection in Perioperative Neurological Injury
Perioperative neurological injury is one of the most serious adverse complications of general anesthesia. Manifestations are on a diverse spectrum ranging from dense coma and brain death to the relatively subtle (though importantly very disabling) postoperative cognitive dysfunction (POCD). Thus, perioperative brain damage is a major concern after cardiac surgery and is one such surgical insult where both cerebral ischemia and the POCD have a significantly higher incidence compared to noncardiac surgery.
While the etiology of perioperative neurological injury is incompletely understood, it is believed that in addition to ischemia due to hypoperfusion and cerebral microembolization, followed by reperfusion injury, cardiac surgery induces a systemic inflammatory response that may act in concert to disrupt the BBB and cause cerebral edema and inflammation, thereby leading to neurocognitive dysfunction. This is supported by animal models demonstrating that cardiopulmonary bypass leads to opening of the BBB. Importantly, more recent studies have also corroborated these results in patients undergoing cardiac surgery. Reinsfelt et al. demonstrated a pronounced cerebral inflammatory response in patients following “on-pump” surgical aortic valve replacement. Their results show increased levels of proinflammatory cytokines in CSF as well as BBB disruption and glial cell injury as assessed using biomarkers of BBB dysfunction and astrocytic damage in CSF. Further, using MRI-DTI and FLAIR, others found subclinical BBB disruption in both on- and off-pump cardiac surgery patients.
Nussmeier et al. conducted a randomized, clinical trial in patients undergoing cardiac surgery with normothermic cardiopulmonary bypass, in which they compared the infusion of thiopental with fentanyl in control subjects. They concluded that thiopental offers neuroprotection in patients undergoing cardiopulmonary bypass. However, in a previous randomized trial in patients undergoing coronary artery bypass grafting comparing thiopental to placebo, Zaidan et al. reported that, contrary to the Nussmeier et al. results, there was no significant difference in either the incidence of neurological deficits or rate of recovery among the two groups. It was concluded that thiopental does not offer neuroprotection in patients undergoing coronary artery surgery. While many now regard thiopental as “an agent of the past,” this example highlights the difficulty in ascertaining a clear outcome signal in these types of studies. The heterogeneity of the population and the methods used are the biggest problems when comparing these and other studies.
Similarly a review of numerous other clinical trials investigating the neuroprotective properties of other anesthetics has led to ambiguous conclusions. As a result, the neuroprotective statuses of both lidocaine and ketamine are currently still controversial. Propofol does not appear to have significant neuroprotective properties in patients undergoing cardiac surgery.
It is important to note that the follow-up period for a number of these studies was quite short (5–10 days postsurgery), which may have contributed to the significant findings. Thus, the question of whether any anesthetic neuroprotection is observed for a long term still remains. One randomized trial investigating the influence of propofol versus desflurane on the long-term incidence of POCD in patients undergoing coronary artery bypass surgery found that although desflurane was associated with decreased incidences of POCD early in recovery (4–7 days postsurgery), there was no significant difference in incidence between the two groups at 3 months postsurgery. This result is in agreement with others who also looked at long-term follow-up and found no evidence for neuroprotection on POCD incidence.
Antiinflammatory Effects of General Anesthetics in the Treatment of Refractory Status Epilepticus
SE refers to the state in which a patient has continuous or rapidly repeating seizures most commonly generalized tonic–clonic. To treat SE, benzodiazepines are used as first-line therapy (first 30 min) and intravenous antiepileptic drugs, such as phenytoin, are used as second-line therapy (30–120 min). If SE does not respond to either first- or second-line therapy, it is considered refractory SE and requires the application of general anesthetics.
While the neuronal molecular mechanisms by which general anesthetics work as antiepileptic drugs are widely accepted—propofol and thiopental are γ-aminobutyric acid (GABA) receptor agonists and ketamine is an NMDA receptor antagonist—these anesthetic drugs also have important immunomodulatory effects that partially overlap with those of corticosteroids. Thiopental, propofol, and ketamine exert potent antiinflammatory effects that are mediated through inhibition of the activation of NFκB, a transcription factor essential for the expression of proinflammatory cytokines, in the experimental setting. Studies in patients have also corroborated the antiinflammatory effects of ketamine. Sevoflurane lacks the antiinflammatory effects of general anesthetics and thus may actually have an epileptogenic effect.
BBB breach has been shown to decrease seizure threshold and promote seizure development in both animals and humans. While general anesthetics have direct antiepileptic neuronal effects, they may be more effective in treating refractory SE because of their antiinflammatory properties that prevent BBB disruption and promote the repair of the cerebrovasculature.
Antiinflammatory Considerations in Barbiturate Induced Coma for Traumatic Brain Injury
Increased intracranial pressure (ICP) or intracranial hypertension occurs commonly in 25–40% of patients with severe traumatic brain injury usually as a consequence of both vasogenic and cytotoxic edema. Vasogenic edema is secondary to BBB disruption and results in the extravasation of proteins and fluid from the cerebrovasculature into the extracellular space. On the other hand, cytotoxic edema—the accumulation of intracellular fluid—is believed to occur as a result of loss of the cell’s ability to regulate ionic gradients. The Monroe–Kellie doctrine determines that due to the rigidity of the skull, cerebral edema causes a significant increase in ICP and cerebral ischemia once the compensatory mechanisms are overwhelmed. However, despite aggressive management strategies, refractory intracranial hypertension may persist in approximately 10–15% of patients with severe TBI.
High-dose barbiturates are recognized as an effective therapy for controlling refractory intracranial hypertension. One randomized, multicenter trial found that inducing barbiturate coma in such patients resulted in double the chance of achieving ICP control. High-dose barbiturates, when used in hemodynamically stable patients (to account for the effects on mean arterial pressure), are believed to lower ICP through the suppression of cerebral metabolism, which reduces cerebral blood volume and ICP due to the coupling of cerebral blood flow to regional metabolic demands.
Thiopental exerts potent antiinflammatory effects through the inhibition of NFκB activation. Importantly, it has been demonstrated that it is the thio-group of thiopental that is of functional importance for this inhibitory effect, as the oxibarbiturate analogs of thiobarbiturates (such as pentobarbital vs thiopental) fail to inhibit NFκB in equimolar amounts. Considering that vasogenic edema is secondary to BBB disruption, thiopental may thus help to decrease cerebral edema by promoting the repair of the BBB through its antiinflammatory effects—although it is important to note that it is actually cytotoxic edema that is believed to be the more insidious of the two types of edema involved in intracranial hypertension.
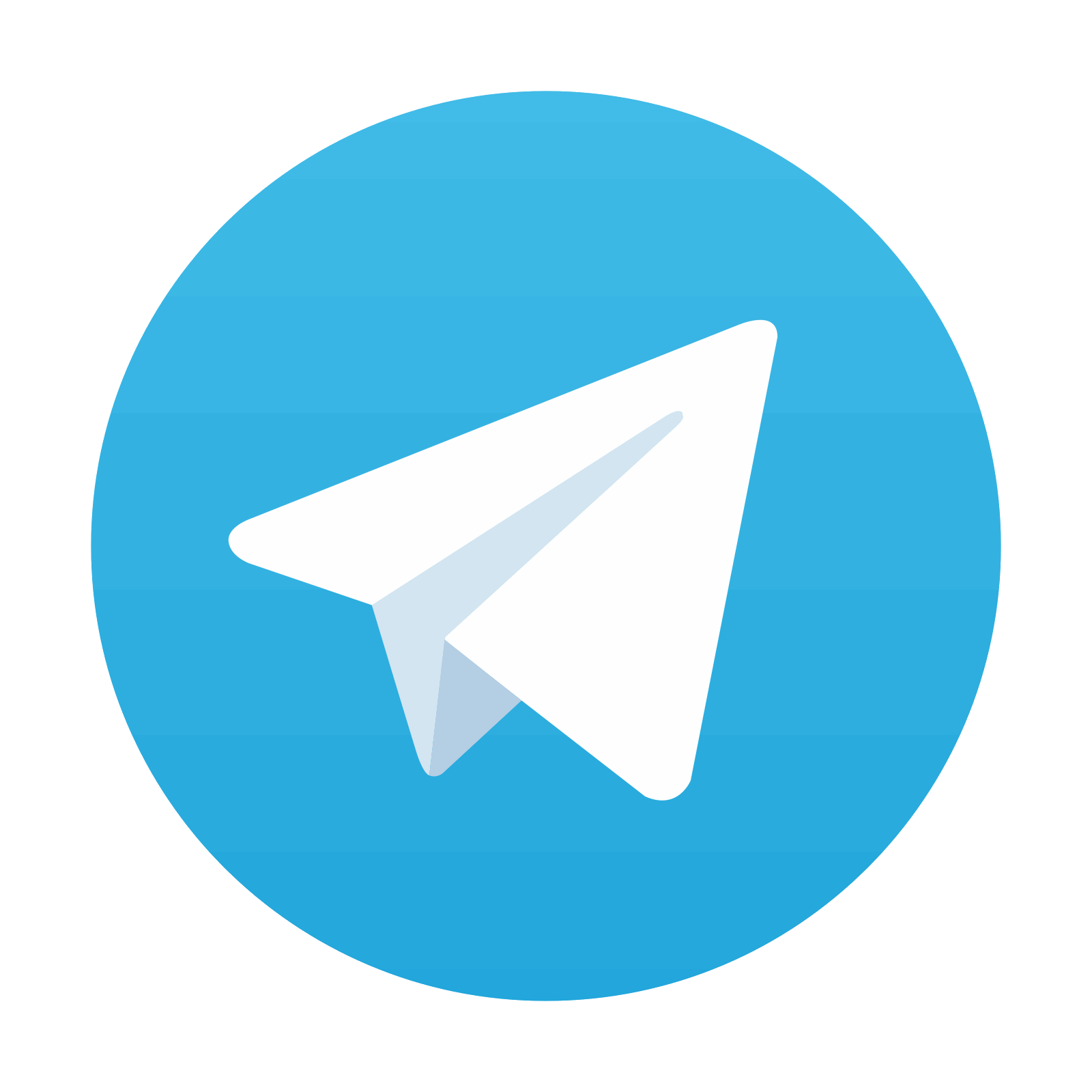
Stay updated, free articles. Join our Telegram channel

Full access? Get Clinical Tree
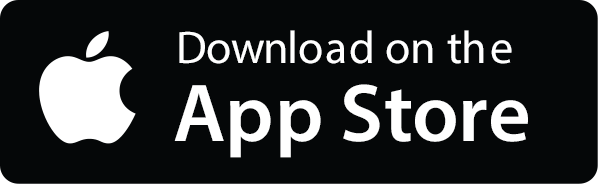
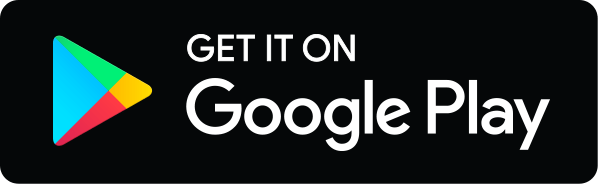