Biological Agents of Mass Destruction
Angeline A. Lazarus
Asha Devereaux
Lawrence C. Mohr Jr
Overview
The use of biological agents in warfare has been recorded throughout history. The first reported biological attack occurred in 1346 when the Tartar army used catapults to throw plague-infected corpses into the city of Kaffa. During the French and Indian War, British forces supplied blankets laden with smallpox to Native Americans supportive of the French. This caused a widespread epidemic of smallpox, leading to the surrender of Fort Carillon by Native American defenders and subsequent outbreaks of smallpox among tribes in the Ohio region [1]. In World War II, a Japanese plane reportedly dispersed rice and fleas infected with the plague organism over the city of Chu Hsien, China. An epidemic of bubonic plague developed in the Chu Hsien region shortly after this event [1].
In 1972, the United States and 161 other nations signed the Convention on the Prohibition of the Development and Stockpiling of Biological and Toxin Weapons. This international treaty prohibits the production of biological weapons and mandates the destruction of existing stockpiles. However, in 1979, there was an accidental release of aerosolized anthrax from the Institute of Microbiology and Virology at Sverdlovsk in the former Soviet Union. This resulted in an outbreak of inhalational anthrax and at least 66 deaths among the local civilian population [1].
In 1999, the Centers for Disease Control and Prevention (CDC) was designated as the lead agency in the United States for planning the public health response to a bioterrorism attack. Several reports published about that same time indicated that the risk of biological terrorism was increasing and that the use of biological agents, as both large-scale and small-scale weapons, was being actively explored by many nations and terrorist groups [2,3,4,5,6]. The concern expressed in these reports was realized after the attack on the World Trade Center in the fall of 2001, and when 22 cases of anthrax occurred in the United States as a result of anthrax spores being sent through the U.S. mail. There were 5 deaths among the 22 patients with anthrax [7,8]. These attacks demonstrated significant vulnerabilities of the United States to bioterrorism and the need for healthcare providers to be prepared to deal with bioterrorism attacks in their respective communities.
In 2002, the CDC published the Public Health Assessment of Potential Biological Terrorism Agents [9]. In this publication, potential bioterrorism agents were placed in one of three categories for the planning of public health preparedness. The agents in each category are summarized in Table 213.1. Category A agents have the greatest potential for the production of mass casualties and a major adverse public health impact. Category B agents have some potential for large-scale dissemination and mass casualties, but would be expected to cause less illness and death than Category A agents. Category C agents are those that do not pose a high bioterrorism threat at the present time, but could emerge as a future threat. This chapter focuses on Category A agents that have the greatest ability to cause mass casualties and significant loss of life. The Category B agent, ricin, is also discussed because of its unique potential to be used as a clandestine agent of terrorism.
Smallpox
The last case of endemic smallpox occurred in Somalia in 1977. In 1980, the World Health Organization (WHO) declared that the disease was eradicated. However, in recent years there has been renewed concern about the variola virus, the causative agent of smallpox, primarily due to the potential of the variola virus to be used as a biological weapon of mass destruction and the possibility for such a weapon to cause a major smallpox epidemic among infected populations. As a result of this concern, the WHO has restricted the number of laboratories officially authorized to serve as repositories for the variola virus to two: the CDC in Atlanta, Georgia, and the Vektor Institute in Novosibirsk, Russia [10].
Although smallpox has been officially declared to be eradicated, there is a possibility for its reemergence. In the nineteenth century, a major epidemic of smallpox appeared in the icy Sakha Republic in Russia, resulting in significant mortality. In the event of unusual thawing or flooding in that region, there is concern that infected corpses might be a potential source for the reemergence of smallpox. Although no live variola viruses have been isolated in the Sakha region, there is historical evidence of smallpox virus survival in interred and exhumed individuals from the eighteenth century [11].
With the increasing concern of bioterrorism and the possibility for the variola virus to be weaponized, the U.S. Military began smallpox vaccination of its troops on December 13, 2002 [12]. Much has been learned regarding the indications, contraindications, and efficacy of the vaccine since this mass immunization process began. Considerable thought has also been given to the dire consequences of a smallpox attack and the preparations necessary to manage a large-scale epidemic resulting from such an attack [13]. This section focuses on those aspects of smallpox infection that are most relevant to the critical care physician.
Virology
The causative agent of smallpox, the variola virus, is a member of the Poxviridae family, subfamily Chordopoxvirinae, and genus Orthopoxvirus. This genus also includes vaccinia (used in the smallpox vaccine), monkeypox virus, camelpox, and cowpox. The variola virus, like other members of the Poxviridae family, is a large, enveloped, DNA virus. Poxviridae viruses
are the only viruses that can replicate in the cytoplasm of cells without involvement of the cell nucleus. The variola virus has a brick-shaped morphology, measures 260 by 150 nm, and has one of the largest viral genomes known. Its large genome makes it difficult to genetically engineer or synthesize the virus in the laboratory. Humans are the only known reservoir for the variola virus, although monkeys are susceptible to infection [14]. The variola virus is very stable and maintains infectivity for long periods of time outside the human host [15]. There are two strains of variola, variola major and variola minor. Variola major is more virulent with a mortality rate between 20% and 50% in infected individuals. Variola minor causes a similar illness, but the mortality is less than 1% [16].
are the only viruses that can replicate in the cytoplasm of cells without involvement of the cell nucleus. The variola virus has a brick-shaped morphology, measures 260 by 150 nm, and has one of the largest viral genomes known. Its large genome makes it difficult to genetically engineer or synthesize the virus in the laboratory. Humans are the only known reservoir for the variola virus, although monkeys are susceptible to infection [14]. The variola virus is very stable and maintains infectivity for long periods of time outside the human host [15]. There are two strains of variola, variola major and variola minor. Variola major is more virulent with a mortality rate between 20% and 50% in infected individuals. Variola minor causes a similar illness, but the mortality is less than 1% [16].
Table 213.1 Bioterrorism Agents and Threat Categories | |||||||||
---|---|---|---|---|---|---|---|---|---|
|
Transmission and Pathogenesis
Transmission of variola occurs from person to person by respiratory droplet nuclei dispersion. Transmission is enhanced by infected individuals who have a cough. Although infrequent, infection has also been known to occur following contact with infected clothing, bedding, or other contaminated fomites [17,18].
Following inhalation, the variola virus seeds the mucus membranes of the upper and lower respiratory tract and then migrates to regional lymph nodes, where viral replication occurs. Viral replication in regional lymph nodes leads to viremia, which results in systemic dissemination of the virus to other organs including the liver, spleen, skin, lung, brain, and bone marrow, where it continues to replicate. Clinical symptoms typically develop after an incubation period of 7 to 17 days.
Clinical Manifestations
Following the initial infection period of 1 to 4 days in which viremia occurs, the clinical manifestations of smallpox appear in a series of distinct phases [18]. These phases that are uniquely characteristic of smallpox are summarized here.
Incubation Phase
The incubation phase of smallpox lasts for 7 to 17 days after infection. During this phase, the virus replicates in regional lymph nodes of the upper and lower respiratory tract. During the incubation phase, infected individuals will most likely be asymptomatic but may have minimal symptoms, such as low-grade temperature elevation or a mild, erythematous rash. Smallpox is not contagious during the incubation phase.
Prodrome Phase
Approximately 7 to 17 days after exposure and initial infection, viremia develops and the variola virus spreads systematically to mucous membranes of the oropharynx, lungs, liver, spleen, bone marrow, and dermal layer of skin. The prodrome phase is characterized by the abrupt onset of high fever (greater than 40°C), headache, nausea, vomiting, and backache. These symptoms are sometimes accompanied by abdominal pain and delirium [19]. These prodromal symptoms typically last for 2 to 4 days. Smallpox may be contagious during the prodrome phase.
Eruption Phase
The eruption phase occurs 2 to 4 days after the onset of prodromal symptoms. Enanthema of the tongue, mouth, and oropharynx precedes the eruption phase by about 1 day. The eruption phase usually begins as small, red maculopapular lesions approximately 2 to 3 mm in diameter. The lesions first appear on the face, hands, and forearms. Lower extremity lesions appear shortly thereafter. The fever usually fades as the skin lesions appear. Symptoms of the prodrome phase may continue and patients can appear very ill. During the next 2 days, the skin lesions become distinctly papular and spread centrally to the trunk. Lesions also appear on the mucous membranes of the oropharynx, and oropharyngeal sections become highly infectious. Smallpox is most contagious during the eruption phase. Healthcare workers, family members, and other close contacts are at greatest risk of contracting smallpox from an infected individual during this phase.
Vesicular Phase
In 2 to 3 days after the eruption of skin lesions, the papular lesions begin to appear vesicular. The vesicles are filled with a thick, opaque fluid and typically range from 2 to 5 mm in diameter. The vesicles are most abundant on the face and extremities. The vesicular phase usually lasts for 2 to 3 days. Humoral antibodies become detectable during this period. Smallpox is contagious during the vesicular phase.
Pustular Phase
The vesicular lesions become pustules approximately 4 to 7 days after the onset of rash. The pustules are sharply raised, firm to the touch, and may have a depressed center and become umbilicated after several days. The pustular phase lasts for 5 to 8 days. Smallpox is contagious during the pustular phase.
Crust Phase
The umbilicated pustules eventually desiccate and become crusted scabs. During this time, there may be a fever spike that may indicate the presence of a superimposed bacterial infection. The crust phase lasts for 5 to 7 days. The crusts contain virus particles and smallpox remains contagious during this phase.
Desquamation Phase
Approximately 2 weeks after the eruption of the rash, desquamation begins. During this phase, the crusts separate from the skin and begin to fall off. Crusts on the palms and soles persist the longest and typically desquamate last. Virus particles are found in the fallen-off crusts and patients are infectious until all crusts separate and fall off. The desquamation phase typically lasts for several weeks. After the crusts fall off the skin, lesions heal and form depressed, depigmented scars.
There are several important characteristics of the smallpox skin lesions that can help to distinguish smallpox from varicella infections (chickenpox). The sequential appearance of the various types of skin lesions described previously is one important characteristic. The distribution of the skin lesions is also characteristic. Smallpox lesions appear first on the face and hands, then on the upper and lower extremities and, over the course of approximately 1 week, eventually spread to the trunk. In all phases of smallpox, there is a predominance of skin lesions on the face and extremities. Another important characteristic of smallpox is that skin lesions are mostly of the same type and same stage of development throughout each clinical phase. The synchronous and centrifugal nature of the smallpox skin lesions is the hallmark of this disease. In contrast, the skin lesions associated with varicella infections are greatest on the trunk, spare the hands and soles, and are at multiple stages at any given time, with papules, vesicles, and crusts all present simultaneously [18].
The mortality rate from the usual variety of smallpox is 3% in vaccinated individuals and 30% in those who are unvaccinated [20]. Death from smallpox is presumed to be secondary to a systemic inflammatory response syndrome caused by overwhelming quantities of immune complexes and soluble variola antigen. Smallpox-associated systemic inflammatory response syndrome results in severe hypotension that usually occurs in the second week of illness. Respiratory complications, including pneumonia and bronchitis, are common [18]. Due to fever and fluid shifts during the vesicular stage of the rash, severe intravascular volume and electrolyte imbalance may occur, which can lead to the development of renal failure. Encephalitis (less than 1% affected) and bacteremia may arise, with the risk of each increasing with the severity of the disease and contributing to mortality. Osteomyelitis, arthritis, and orchitis are other rare manifestations.
Table 213.2 Distinguishing Clinical Features in Smallpox and Chickenpox | |||||||||||||||||||||
---|---|---|---|---|---|---|---|---|---|---|---|---|---|---|---|---|---|---|---|---|---|
|
There are two atypical manifestations of smallpox that have very high mortality rates [17]. Hemorrhagic smallpox occurs in less than 3% of infected individuals. The hemorrhagic form is characterized by a short incubation period and an erythematous skin eruption that later becomes petechial and hemorrhagic, similar to the lesions seen in meningococcemia. Most individuals with the hemorrhagic form of smallpox die in 5 to 6 days after onset of the rash. The malignant form, or “flat smallpox,” is characterized by a fine-grained, reddish, nonpustular, and confluent rash, occasionally with hemorrhage. The malignant form occurs in 2% to 5% of infected individuals. Patients with the malignant form have severe systemic illness and most die within several days. Pulmonary edema occurs frequently in both hemorrhagic and malignant smallpox and contributes to the high mortality rates [20].
The primary long-term sequela of smallpox is the “pockmarks” that affect the skin. These are pitted lesions that permanently scar the face due to infection of sebaceous glands. Panophthalmitis, viral keratitis, and corneal ulcers can cause permanent blindness in 1% of infected individuals. Infection with smallpox results in lifelong immunity [20].
Diagnosis
The differential diagnosis of papulovesicular lesions that can be confused with smallpox includes chickenpox (varicella), shingles (varicella-zoster), disseminated herpes simplex, monkeypox, drug eruptions, generalized vaccinia, eczema vaccinatum, impetigo, bullous pemphigoid, erythema multiforme, molluscum contagiosum, and secondary syphilis. Severe chickenpox (varicella) is the most common eruption that can be confused with smallpox. Table 213.2 delineates clinical features that can help to distinguish chickenpox from smallpox.
Confirmation of smallpox can be performed by the analysis of skin scrapings, vesicular fluid, and oropharyngeal swabs. Specimens should be collected using respiratory and contact isolation procedures, ideally by previously vaccinated personnel. Specimen collection techniques and guidelines are available from public health departments, the CDC, and the WHO [18]. If smallpox is suspected, the local public health department should be notified immediately. Public health departments can provide valuable assistance in collecting specimens and getting them to an appropriate laboratory for analysis. The brick-shaped variola virus is distinguished from varicella-zoster by electron microscopy. However, polymerase chain reaction (PCR) assays are the mainstay of diagnosis at the present time. Serologic testing is not useful in differentiating the variola virus from other orthopoxviruses. Laboratory specimens
should only be manipulated and processed at laboratories with Biosafety Level 4 facilities [17]. Again, local public health departments can assist in getting specimens to an appropriate laboratory.
should only be manipulated and processed at laboratories with Biosafety Level 4 facilities [17]. Again, local public health departments can assist in getting specimens to an appropriate laboratory.
Infection Control
Although the primary transmission of smallpox is via respiratory droplet nuclei, infected clothing and bedding can also transmit disease [11]. Individuals with smallpox are most infectious within the first 7 to 10 days of the rash, but the disease is contagious until all crusted lesions have fallen off [17]. Secondary cases occur in family members or healthcare workers who are exposed to an infectious individual. If a new outbreak were to occur, it is anticipated that the rate of transmission may be as high as 10 new cases for every infected person. All individuals who have direct contact with the index case should be quarantined for 17 days in respiratory isolation. Home quarantine will be necessary in mass casualty situations. Healthcare workers caring for infected individuals should be vaccinated and use strict airborne and contact isolation procedures. Infected patients should be placed in respiratory isolation and managed in a negative-pressure isolation room, if possible. Patients should remain isolated until all crusted lesions have fallen off. Patients should also be vaccinated if the disease is in the early stage. If performed early, vaccination may significantly decrease the severity of smallpox symptoms [18].
Treatment
There is no U.S. Food and Drug Administration (FDA)-approved drug for the treatment of smallpox. At the present time, treatment is primarily supportive. Supportive care includes maintaining general hygiene, appropriate antibacterial therapy for secondary skin infections, daily eye irrigation for severe cases, and ensuring that the patient receives adequate nutrition and hydration. Topical treatment with idoxuridine can be considered for the treatment of corneal lesions.
Animal studies have suggested that cidofovir has activity against orthopoxviruses, including variola. Cidofovir given at the time of, or immediately following, exposure has the potential to prevent cowpox, vaccinia, and monkeypox. Aerosolized cidofovir has been shown to protect mice against intranasal challenge with the cowpox virus [21]. Additional animal studies are being conducted with other antiviral agents. A new class of potent antipoxviral drugs (ST-246 and lipid-soluble cidofovir CMX001) has been developed and stockpiled [22]. It has been demonstrated that vaccinia immune globulin decreases pulmonary viral loads and pneumonitis in animals with vaccinia or cowpox. However, there is no evidence that the use of vaccinia immune globulin offers any survival or therapeutic benefit in patients infected with smallpox.
Immunization
Smallpox eradication was possible due to a successful worldwide vaccination program with live vaccine viruses. Vaccination continues to be the mainstay of smallpox prevention. First-generation live virus vaccines (Dryvax, APSV, Lancy-Vaxina, L-IVP) were administered by puncturing the skin of the upper arm with a bifurcated needle to induce a robust humoral immunity. Many side effects, reactions, and contraindications resulted from the use of these vaccines. Second-generation vaccines produced in the last 5 to 10 years still contain replication-competent viruses produced in tissue culture and elicit an immunity level similar to first-generation vaccines. A lyophilized preparation of live vaccinia virus (Dryvax, Wyeth Laboratories, Lancaster, PA) that contains polymyxin B, streptomycin, tetracycline, and neomycin was used in the 2004–2005 U.S. vaccination program. Third-generation vaccine formulations have utilized attenuated vaccinia strains (LC16m8, MVA, NYVAC, DVVL) with the hope of an improved safety profile [23]. There has been considerable discussion regarding the efficacy of pre-exposure mass vaccination to protect the public against smallpox in the event of a bioterrorism attack. At the present time, the CDC recommends voluntary vaccination for those likely to be exposed to smallpox and “ring vaccination” in the event of a smallpox outbreak [24,25].
Within 1 week of primary vaccination, the Jennerian pustule develops a gray-white loculated pustule with central umbilication. This marks a “major reaction” and implies successful vaccination. The Jennerian pustule will then crust and darken and remain for approximately 3 weeks following immunization. Successful revaccination is marked by mild induration at the inoculation site. A repeat vaccination attempt is suggested for any equivocal responses. Due to the shortage of vaccine supply in early 2002, dilution studies showed that the vaccinia virus diluted to a titer as low as 107.0 plaque-forming units (pfu) per mL (approximately 10,000 pfu per dose) will result in vesicle formation in 97% of inoculated individuals [26]. Dilutions to a titer of 106.5 pfu per millimeter were only effective in 70% of those immunized. Lower doses decrease success to as low as 15% [27]. A successful primary vaccination offers full immunity for 5 to 10 years in 95% of those immunized. Successful revaccination is likely to be effective for 10 to 20 years.
Site of vaccination: Outer aspect of upper arm over the insertion of the deltoid muscle.
Preparation of skin: None, unless the site is obviously dirty. Use water to cleanse the site because the use of a disinfectant can kill the virus.
Withdrawal of vaccine from the ampule: A cool, sterile bifurcated needle is inserted into the reconstituted vaccine ampule. A droplet is sufficient for vaccination and is contained within the fork of the needle. Never dip the same needle back into the ampule to avoid contamination.
Application: The needle is held at 90 degrees perpendicular to the skin; the needle then touches the skin to release the droplet. For primary vaccination, three strokes are made in a 5-mm area. For revaccination, 15 up/down, perpendicular strokes of the needle are rapidly made in the area of 5 mm diameter (through the drop of vaccine deposited on the skin). The strokes should be sufficiently vigorous so that a trace of blood appears at the site. If blood does not appear, the procedure (three strokes) should be repeated with the same needle.
Dressing: Although the WHO does not recommend a dressing, the CDC recommends a loose sterile gauze dressing covered by a semipermeable dressing to prevent transmission of the virus. Absorb the excess blood and vaccine with gauze, and dispose the gauze in a biohazard receptacle.
Unused Vaccine: Unused vaccine is good for 90 days after reconstitution and should be refrigerated without any special light precautions.
The most common adverse reactions following smallpox vaccination are tenderness and erythema at the injection site and secondary bacterial infections. Fever, malaise, local lymphadenopathy, erythema multiforme, Stevens–Johnson syndrome, urticaria, exanthems, contact dermatitis, and erythematous papules have been reported [29]. Inadvertent autoinoculation of another body site, generalized vaccinia (vesicles or pustules appearing on normal skin distant from the vaccination site), eczema vaccinatum, vaccinia keratitis, and progressive vaccinia
have been reported in primary vaccinations. Postvaccinia encephalitis is a very rare complication. Myopericarditis was reported in 200 cases from the recent military vaccination program, at a rate of 117 cases per million vaccinees [12,30]. The cause of postvaccination myopericarditis is not well understood but is probably immunologically mediated and not from direct viral infection of the myocardium. As a result, the CDC has recommended that routine vaccination should not be given to anyone with known previous cardiac disease or three or more risk factors for coronary artery disease [18,31]. The reported rate of cardiac mortality is 1.1 deaths per million primary vaccines. A review of approximately 39,000 people vaccinated against smallpox (36% primary vaccinations and 64% revaccinations) reported the following adverse reactions: encephalitis in 1 individual, myopericarditis in 21 individuals, generalized vaccinia in 2 individuals, inadvertent inoculation in 7 individuals, and ocular vaccinia in 3 individuals [32].
have been reported in primary vaccinations. Postvaccinia encephalitis is a very rare complication. Myopericarditis was reported in 200 cases from the recent military vaccination program, at a rate of 117 cases per million vaccinees [12,30]. The cause of postvaccination myopericarditis is not well understood but is probably immunologically mediated and not from direct viral infection of the myocardium. As a result, the CDC has recommended that routine vaccination should not be given to anyone with known previous cardiac disease or three or more risk factors for coronary artery disease [18,31]. The reported rate of cardiac mortality is 1.1 deaths per million primary vaccines. A review of approximately 39,000 people vaccinated against smallpox (36% primary vaccinations and 64% revaccinations) reported the following adverse reactions: encephalitis in 1 individual, myopericarditis in 21 individuals, generalized vaccinia in 2 individuals, inadvertent inoculation in 7 individuals, and ocular vaccinia in 3 individuals [32].
Contraindications to smallpox vaccination are infants less than 1 year of age, immune suppression, eczema, exfoliative skin conditions, pemphigus, cardiac disease as previously described, allergy to any component of the vaccine, and pregnant or breastfeeding women. Individuals who are taking, or have taken, high-dose corticosteroids should not be vaccinated within 1 month of completing corticosteroid therapy. Although testing for human immunodeficiency virus (HIV) is not mandatory prior to smallpox vaccination, the Advisory Committee on Immunization Practices has recommended that HIV testing be readily available to all individuals considering smallpox vaccination [25]. Individuals with a contraindication to vaccination should avoid people who have been recently vaccinated, due to possible transmission of vaccinia from viral shedding at the vaccination site. A small number of deaths (12/68) in the 1960s were attributed to unvaccinated persons exposed to recently vaccinated friends or family members [33].
Healthcare workers must be aware of the possibility for the nosocomial transmission of vaccinia during the hospitalization of a recently vaccinated patient. Nosocomial infection can result in mortality up to 11%. Direct carriage of the virus on the hands, nasal mucosa, fomites, contaminated equipment, and laundry has been implicated in the transmission of vaccinia [34]. Risk of the nosocomial transmission can be mitigated by several simple precautions. Semipermeable dressings should be applied to the site of a recent vaccination and changed frequently if there is evidence of the accumulation of purulent material. Gloves should be worn during dressing changes and meticulous handwashing with antimicrobial soap should be performed by all healthcare providers, both before and after contact with a recently vaccinated patient. Contaminated dressings should be disposed of in a biohazard container. Care should be taken to avoid contact of the vaccination site with material or equipment that could transmit the virus to other individuals. Clothing, towels, and other cloth materials that have contact with the site can be decontaminated by routine laundering with hot water. If at all possible, healthcare workers who are responsible for dressing changes should be vaccinated against smallpox, but nonvaccinated individuals are acceptable as long as appropriate precautions are observed [25]. Sexual transmission of vaccinia virus from a recently immunized active duty military member to a civilian has been recently reported following immunization [34].
Treatment of adverse effects following smallpox vaccination include supportive therapy; administration of vaccinia immune globulin; cidofovir; and an antiviral ophthalmic ointment, such as trifluridine or vidarabine, for eye involvement. Vaccinia immune globulin is available from the CDC, although the supply is limited. Cidofovir is available at no cost from the CDC under investigational use if a patient fails to respond to vaccinia immune globulin, the patient is near death, or all inventories of vaccinia immune globulin are depleted. The dose of cidofovir is 5 mg per kg, given intravenously, during 60 minutes as a single dose [18].
Due to the adverse effects of vaccinia, the federal government contracted with Acambis (Cambridge, England) and Baxter Healthcare (Cambridge, MA) to purchase a cell culture-derived smallpox vaccine that has demonstrated 94% efficacy in phase II clinical trials. Further research on the development of safer smallpox vaccines is currently in progress. In August 2007, the ACAM2000 vaccine was licensed by the FDA for administration to people at high risk of smallpox or other orthopoxvirus diseases. Over 200 million doses of the vaccine have been purchased by the U.S. Strategic National Stockpile (covers approximately 62% of the population), but it is not available for commercial use. Clinical trials show similar efficacy and side effects profile to the Dryvax vaccination, but ACAM200 cannot be diluted [18,22].
In the event of an international release of variola virus, the priority of vaccination is as follows [24,25,35]:
Group 1: Individuals directly exposed to the release.
Group 2: Individuals with face-to-face household contact with a directly exposed individual.
Group 3: Personnel directly involved in the evaluation, care, or transport of infected patients.
Group 4: Laboratory personnel responsible for handling and processing specimens, and others who may be exposed to infectious materials.
Anthrax
In the fall of 2001, 22 cases of anthrax with 5 deaths occurred in the United States as a result of anthrax spores in envelopes sent through the U.S. mail. Early recognition and treatment of anthrax by astute clinicians was responsible for preventing additional deaths [7,8]. Anthrax is thought to be the most likely biological agent to be used in a bioterrorism attack. Identification of a single case should prompt notification of local, state, and national public health authorities [36]. The CDC has rapid response teams with specialized expertise, training, and equipment that can be deployed immediately to assist local authorities in the event of a bioterrorism attack [8]. Cases of anthrax in animals have been reported to occur sporadically in North America. In 2006, an outbreak was reported in Canada that affected over 900 animals. Two cases of cutaneous anthrax and one case of inhalational anthrax in humans were reported in the United States from occupational exposure associated with drum making using animal hides from West Africa [37].
Microbiology
Bacillus anthracis (from the Greek word for coal, anthrakis) is a large, Gram-positive, aerobic, spore-forming, nonmotile bacillus. B. anthracis is found in the soil of many regions of the world, where it exists in the endospore form. Its virulence is determined by two plasmids. One plasmid involves the synthesis of a poly-D-glutamic acid capsule that inhibits phagocytosis of vegetative bacilli and the other contains genes for the synthesis of exotoxins. The exotoxins are known as protective antigen, edema factor, and lethal factor. The protective antigen is a binding protein that is necessary for entry into the host cell and combines with both edema factor and lethal factor to produce “edema toxin” and “lethal toxin” [38]. Edema toxin converts adenosine triphosphate to cyclic adenosine monophosphate (cAMP), resulting in high intracellular cAMP levels that impair water homeostasis and thereby cause cellular edema. Lethal toxin stimulates the overproduction of cytokines, primarily tumor necrosis factor-α and interleukin-1-β that cause
macrophage lysis. The sudden release of inflammatory mediators appears to be responsible for the marked clinical toxicity of the bacteremic form of anthrax.
macrophage lysis. The sudden release of inflammatory mediators appears to be responsible for the marked clinical toxicity of the bacteremic form of anthrax.
Clinical Manifestations
There are three forms of anthrax infection. The clinical characteristics of each form are determined by the route of entry of the anthrax spores. Cutaneous anthrax is the most common naturally occurring form, comprising approximately 95% of cases reported. Spores enter the body through breaks in the skin and begin low-level germination within days, resulting in soft tissue or mucosal edema and localized necrosis. Initially, a painless, pruritic macule appears, followed by vesiculation, ulceration, and a black, “coal-like” painless eschar, from which anthrax gets its name. The eschar sloughs within 2 to 3 weeks of onset [39]. Abscess formation occurs only with superinfection. Endospores phagocytosed by macrophages are often transported to regional lymph nodes causing painful lymphadenopathy and lymphangitis. Infrequently, cutaneous anthrax may spread hematogenously with significant morbidity and death in a small number of individuals. Cutaneous anthrax has been reported to cause microangiopathic hemolytic anemia, renal dysfunction, and coagulopathy [40].
Gastrointestinal and oropharyngeal anthrax usually occur following the ingestion of contaminated meat. This is a rare manifestation of anthrax, with most cases occurring in Africa. Mucosal ulcers, edema, and regional lymphadenopathy are initial manifestations. In the oropharyngeal form, pseudomembranes are seen in the oropharynx and upper airway obstruction can develop. In the gastrointestinal form, a necrotizing infection progresses from the esophagus to the cecum. Fever, nausea, vomiting, abdominal pain, gastrointestinal bleeding, and bloody diarrhea are typical symptoms. Anemia, electrolyte abnormalities, and hypovolemic shock may follow. Massive ascites that is occasionally purulent has been reported. Death results from intestinal perforation or septicemia [20].
The third form of anthrax infection is inhalational anthrax. Anthrax spores are 1 to 1.5 μm in size and easily deposit in the alveoli following inhalation. There, the endospores are phagocytosed by the pulmonary macrophages and transported via lymphatics to the mediastinal lymph nodes, where they may remain dormant as “vegetative cells” for approximately 10 to 60 days or longer. Once germination in the lymph nodes is complete, bacterial replication occurs. The replicating bacteria release edema and lethal toxins that produce a hemorrhagic mediastinitis.
In some patients, the initial symptoms are relatively mild and nonspecific, resembling an upper respiratory tract infection. Fever, chills, fatigue, nonproductive cough, nausea, dyspnea, chest pain, and myalgias are common presenting complaints (Table 213.3) [36,37]. These symptoms typically last for 2 to 3 days and then progress to a more severe, fulminant illness. However, some patients present with fulminant illness without any prodromal symptoms. Dyspnea and shock characterize the fulminant phase of inhalational anthrax. The number of spores inhaled, age of the patient, and the underlying immune status most likely affect the clinical course of the disease [41]. Chest radiographs show mediastinal widening and pleural effusions that may be massive (Fig. 213.1). B. anthracis bacilli, bacillary fragments, and anthrax antigens can be identified by immunohistochemistry testing of the pleural fluid [42]. Although parenchymal infiltrates are not prominent, a focal hemorrhagic necrotizing pneumonitis, resembling the Ghon complex of tuberculosis, was noted in 11 of 42 autopsy patients from the accidental release of anthrax in Sverdlovsk, USSR, in 1979. Almost 50% of patients with inhalational anthrax develop hemorrhagic meningitis as a result of the hematogenous spread of B. anthracis. Massive bacteremia, with up to 107 to 108 bacteria per mL of blood, causes overwhelming septic shock and death within hours after the onset of symptoms. According to the Defense Intelligence Agency, the lethal dose to kill 50% of persons exposed (LD50) to weapons-grade anthrax is 2,500 to 55,000 spores [43]. However, as few as one to three spores may be sufficient to cause infection [36].
Table 213.3 Clinical Features of Inhalational Anthrax (U.S. Outbreak 2001, n = 10) | ||||||||||||||||||||||||||||||
---|---|---|---|---|---|---|---|---|---|---|---|---|---|---|---|---|---|---|---|---|---|---|---|---|---|---|---|---|---|---|
|
Diagnosis
A high index of suspicion is necessary to make the diagnosis of anthrax when patients present with a severe flulike illnesses. Laboratory findings from the U.S. outbreak in 2001 showed that patients had a mild neutrophil-predominant leukocytosis,
in the range of 7,500 to 13,300 per μL. Peak white blood cell count during illness ranged from 11,900 to 49,600 per mm3. Elevated transaminases, hyponatremia, and hypoxemia were also noted [7,37,41,43]. One hundred percent of these patients had an abnormal chest radiograph with mediastinal widening, pleural effusions, consolidation, and infiltrates predominating. The presence of mediastinal widening that may require computed tomography scanning to elucidate should be considered diagnostic of anthrax until proven otherwise [44,45,46]. Hemorrhagic necrotizing lymphadenitis and mediastinitis are pathognomonic of anthrax, but these are autopsy findings of these conditions [19]. B. anthracis is easily cultured from blood, cerebral spinal fluid, ascites, and vesicular fluid with standard microbiology techniques. The laboratory should be notified when the diagnosis of anthrax is being considered, as many hospital laboratories will not further characterize Bacillus species unless requested. Biosafety Level 2 conditions apply for workers handling specimens because most clinical specimens have spores in the vegetative state that are not easily transmitted [36]. The presence of large Gram-positive rods in short chains that are positive on India ink staining is considered presumptive of B. anthracis, until the results of cultures and other confirmatory tests are obtained. Confirmatory testing can be performed by the CDC Laboratory Response Network. Rapid detection tests based on immunohistochemistry, and PCR techniques are available via the Laboratory Response Network [37]. Nasal swabs are not recommended because they are not reliable for making the diagnosis of anthrax. Following the 2001 anthrax attack, there were negative nasal swab results in patients with fatal inhalational anthrax [43]. In June 2004, the FDA approved the Anthrax Quick ELISA test (Immunetics, Inc., Boston, MA) that detects antibodies to the protective antigen of B. anthracis exotoxin. The test can be completed in less than 1 hour and is available to hospital and commercial laboratories by the manufacturer [37].
in the range of 7,500 to 13,300 per μL. Peak white blood cell count during illness ranged from 11,900 to 49,600 per mm3. Elevated transaminases, hyponatremia, and hypoxemia were also noted [7,37,41,43]. One hundred percent of these patients had an abnormal chest radiograph with mediastinal widening, pleural effusions, consolidation, and infiltrates predominating. The presence of mediastinal widening that may require computed tomography scanning to elucidate should be considered diagnostic of anthrax until proven otherwise [44,45,46]. Hemorrhagic necrotizing lymphadenitis and mediastinitis are pathognomonic of anthrax, but these are autopsy findings of these conditions [19]. B. anthracis is easily cultured from blood, cerebral spinal fluid, ascites, and vesicular fluid with standard microbiology techniques. The laboratory should be notified when the diagnosis of anthrax is being considered, as many hospital laboratories will not further characterize Bacillus species unless requested. Biosafety Level 2 conditions apply for workers handling specimens because most clinical specimens have spores in the vegetative state that are not easily transmitted [36]. The presence of large Gram-positive rods in short chains that are positive on India ink staining is considered presumptive of B. anthracis, until the results of cultures and other confirmatory tests are obtained. Confirmatory testing can be performed by the CDC Laboratory Response Network. Rapid detection tests based on immunohistochemistry, and PCR techniques are available via the Laboratory Response Network [37]. Nasal swabs are not recommended because they are not reliable for making the diagnosis of anthrax. Following the 2001 anthrax attack, there were negative nasal swab results in patients with fatal inhalational anthrax [43]. In June 2004, the FDA approved the Anthrax Quick ELISA test (Immunetics, Inc., Boston, MA) that detects antibodies to the protective antigen of B. anthracis exotoxin. The test can be completed in less than 1 hour and is available to hospital and commercial laboratories by the manufacturer [37].
Treatment
Due to the fulminant course of inhalational anthrax, prompt initiation of therapy is essential for survival. Ciprofloxacin (400 mg) or doxycycline (100 mg) given intravenously every 12 hours with one to two other antibiotics that have predicted efficacy against anthrax is currently recommended. Additional antibiotics that are effective against anthrax include rifampin, vancomycin, imipenem, chloramphenicol, penicillin, ampicillin, clindamycin, and clarithromycin. Two survivors of anthrax during the U.S. outbreak received parenteral ciprofloxacin, clindamycin, and rifampin. The addition of clindamycin may attenuate toxin production [44]. There are limited data regarding treatment of pregnant women for anthrax. However, the limited information that is available suggests that the use of ciprofloxacin during pregnancy is unlikely to be associated with a high risk for structural birth defects [47]. Therefore, ciprofloxacin should be given to pregnant women for the treatment of inhalational anthrax unless otherwise contraindicated. Doxycycline is relatively contraindicated in pregnancy and should only be considered if ciprofloxacin is unavailable or absolutely contraindicated. Therapy with ciprofloxacin or doxycycline should continue for 60 days. Patients can be switched to oral therapy with ciprofloxacin (500 mg twice daily) or doxycycline (100 mg twice daily) after fulminant symptoms have resolved and they are stable. The use of systemic corticosteroids has been suggested for meningitis, severe edema, and airway compromise. Parenteral ciprofloxacin and another antibiotic with good central nervous system penetration, such as rifampin, should be part of the initial treatment regimen for anthrax meningitis. Cutaneous anthrax with systemic involvement, significant edema, and lesions of the head and neck should be treated similarly. Uncomplicated cutaneous anthrax can be treated with oral ciprofloxacin or doxycycline for 7 to 10 days, but, due to the possibility of concomitant inhalational exposure, a 60-day course is recommended [36,37].
A review of anthrax cases in adults from 1900 to 2004 noted that fulminant inhalational anthrax is often fatal despite advances in medical care. Early diagnosis and initiation of therapy during the prodromal phase improved survival and are pivotal for decreasing mortality in inhalational anthrax [48]. Similarly, a review of anthrax cases in children from 1900 to 2005 shows that early diagnosis and treatment of all forms of anthrax are critical for improved survival in children [49].
Prophylaxis
All patients exposed to anthrax should receive prophylaxis with oral ciprofloxacin (500 mg twice daily), levofloxacin (500 mg daily), or doxycycline (100 mg twice daily) for 60 days, regardless of laboratory test results. Nasal swabs can confirm exposure to anthrax, but cannot exclude it. High-dose penicillin or ampicillin may be an acceptable alternative for 60 days in patients who are allergic or intolerant to the recommended antibiotics [36,37]. More than 5,000 people received postexposure prophylaxis following the 2001 U.S. outbreak, but only about half completed the 60-day course. The main reasons for discontinuing therapy were gastrointestinal or neurologic side effects (75%) or a low perceived risk (25%).
The anthrax vaccine (AVA-Biothrax) manufactured by BioPort Corporation in Lansing, Michigan, is the only licensed human anthrax vaccine in the United States. The vaccine consists of supernatant material from cultures of a toxigenic, nonencapsulated strain of B. anthracis. A six-dose series has been used by the U.S. Military. The anthrax vaccine is not available to the general public at the present time. Although efficacy data are limited to goat hair mill workers from the 1950s to 1974, fully vaccinated individuals did not contract anthrax as compared to those who did not participate in the vaccine program [50]. Approximately 95% of vaccinated individuals seroconvert after the third dose of vaccine. Data regarding vaccine safety from more than 1 million doses administered to members of the U.S. Military reveal that the adverse events were without any significant pattern or association. The vaccine is generally considered to be safe by the FDA [50]. A review by the U.S. Army Medical Research Institute of Infectious Diseases reported a 1% (101/10,722) incidence of systemic symptoms, most commonly headache. Local or injection site reactions occurred in 3.6% [36]. A study comparing four subcutaneous injections of anthrax vaccine adsorbed (AVA) with three and four intramuscular injections of AVA showed similar immunoprotection at 7 months with less adverse effects at the injection site. Following an aerosolized B. anthracis attack, postexposure prophylactic vaccination and antibiotic therapy remain the most effective and least expensive strategies [51].
Tularemia
Tularemia is a zoonosis found in a wide range of animals, primarily small mammals such as rodents and rabbits. In 1922, tularemia was reported to cause fatal illness in humans [52]. In the late 1920s, tularemia was recognized as a threat to laboratory workers. Tularemia is caused by Francisella tularensis, an intracellular, nonspore-forming, aerobic Gram-negative coccobacillus. In the mid-twentieth century, both the United States and the former Soviet Union developed biological weapons that could disperse F. tularensis [53]. Biological weapons have now been banned and are no longer in production. However, there is concern that F. tularensis could be used as an agent
of bioterrorism. In a 1970 report, the WHO estimated that 50 kg of aerosolized F. tularensis dispersed over a metropolitan area of 5 million people could cause 19,000 deaths and 250,000 incapacitating illnesses [19,54]. The impact of such an attack would probably linger for several weeks to months due to disease relapses [54].
of bioterrorism. In a 1970 report, the WHO estimated that 50 kg of aerosolized F. tularensis dispersed over a metropolitan area of 5 million people could cause 19,000 deaths and 250,000 incapacitating illnesses [19,54]. The impact of such an attack would probably linger for several weeks to months due to disease relapses [54].
Microbiology
F. tularensis is a nonsporulating, nonmotile, Gram-negative coccobacillus. It is a hardy organism, which makes it well suited for use as an agent of bioterrorism. It can survive in moist soil, water, and animal carcasses for many weeks. However, chlorination of water prevents its spread through water contamination. F. tularensis can be aerosolized and inhalation of aerosolized organisms poses a threat to those exposed. The most common isolate, and the most virulent form, is F. tularensis biovar tularensis (Group A). Inoculation or inhalation of as few as 10 organisms may cause clinical disease [55,56,57]. F. tularensis biovar palaearctica is found mostly outside of the United States, most notably in Europe. Transmission of F. tularensis to humans occurs predominantly through tick and fleabites, handling of infected animals, ingestion of contaminated food and water, and inhalation of the aerosolized organism. There is no human-to-human transmission of F. tularensis. As a biological weapon, the organism would most likely be dispersed as an aerosol and cause mass casualties from an acute febrile illness that may progress to severe pneumonia [19].
Epidemiology
Tularemia occurs worldwide but is rare in Africa, Central, South America, and the United Kingdom, with highest incidence in Russia and Scandinavian countries [57,58,59]. In the United States, tularemia cases are reported most often from the south central and western states (Arkansas, Illinois, Missouri, Oklahoma, Tennessee, Texas, Utah, Virginia, and Wyoming). The predominant mode of transmission to humans in the United States is by tick bites, and most cases are reported in spring and summer. Hunters and trappers exposed to animal reservoirs are at high risk for exposure [57,58,59]. In Europe and Japan, mosquito bites and the handling of infected animals appear to cause the disease. A large outbreak of tularemia in 2003 along with small summer outbreaks between 1995 and 2005 in Sweden suggests environmental sources clustering around recreational areas [60]. Tularemia epidemics may have a seasonal presentation. F. tularensis var tularensis, often seen in summer, is tick-borne, while F. palaearctica, seen in fall and winter, is commonly transmitted from contaminated water, rodents, or aquatic animals. An outbreak of tularemia in Martha’s Vineyard, Massachusetts, during the summer of 2000 was associated with lawn mowing and brush cutting [61,62]. A water-borne outbreak resulting in 21 cases of oropharyngeal and 5 cases of glandular tularemia was reported in Georgia [63].
Pathogenesis
F. tularensis enters the human host via the eye, respiratory tract, gastrointestinal tract, or a break in the skin. The virulence of the organism depends on its ability to replicate within the macrophage. On entering the macrophage, the organism proliferates. This is followed by apoptosis of the macrophage and the release of a larger number of organisms, leading to involvement of the local lymph nodes and bacteremia. Once bacteremia develops, F. tularensis infects the lungs, pleura, spleen, liver, and kidney. The host defense against F. tularensis is reported to be T-cell independent in the first 3 days and T-cell dependent after 3 days of infection. Initially, a focal suppurative necrosis with polymorphonuclear cells, macrophages, epithelioid cells, and lymphocytes are noted. The predominant protective mechanism in containing the disease comes from cell-mediated immunity. On histopathology, granulomas with necrosis may be seen in infected organs. Following inhalational exposure, hemorrhagic airway inflammation progressing to bronchopneumonia, pleuritis, and pleural effusion have been reported [55,56,57]. The mucosal immunopathogenesis of F. tularensis in animal models has shown that the antibodies may provide both prophylactic and therapeutic protection against pulmonary infection when there is active cell-mediated immunity [64].
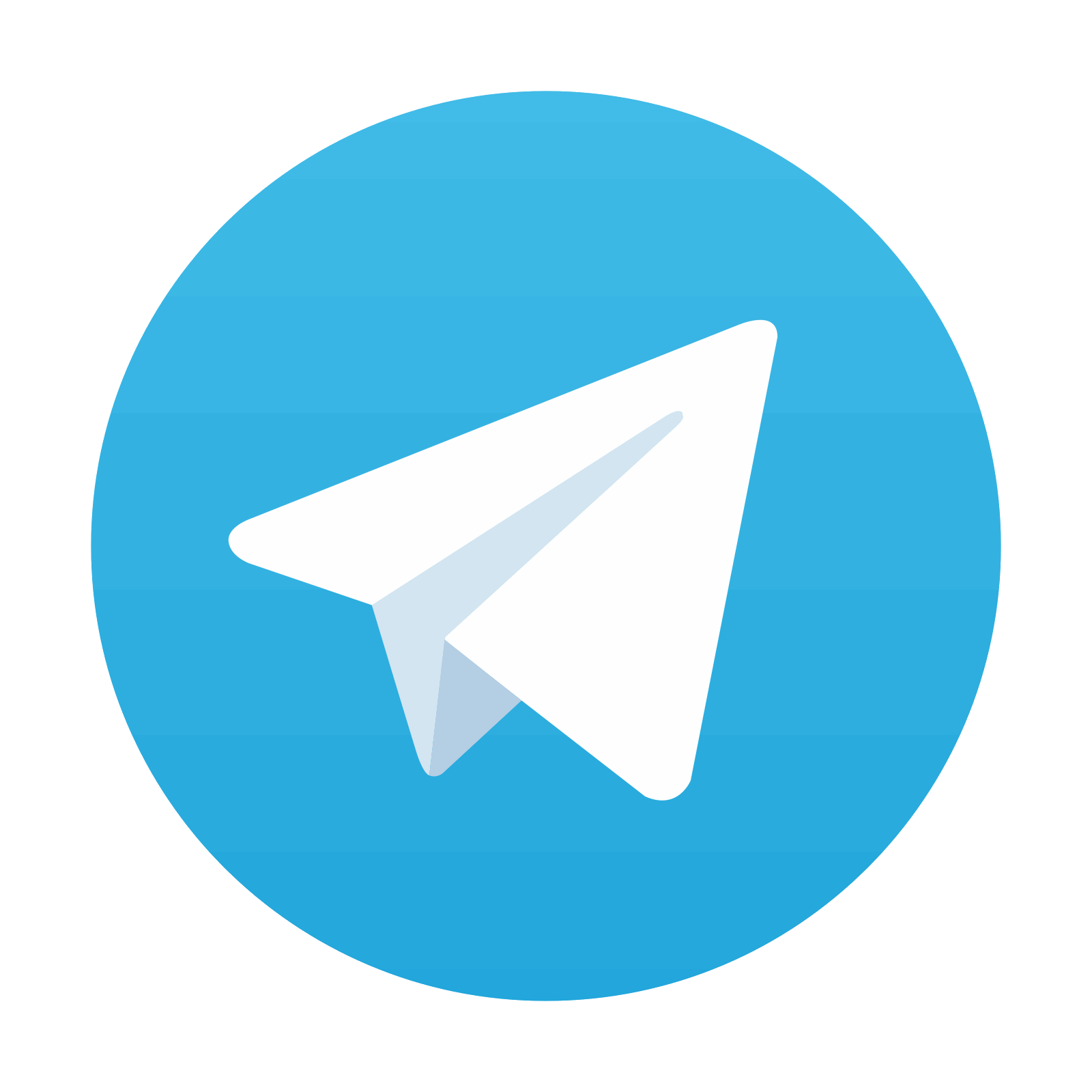
Stay updated, free articles. Join our Telegram channel

Full access? Get Clinical Tree
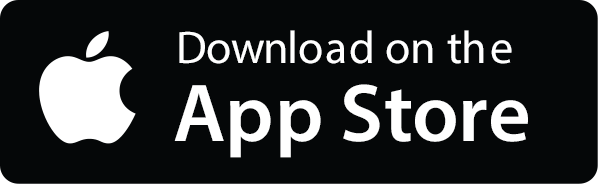
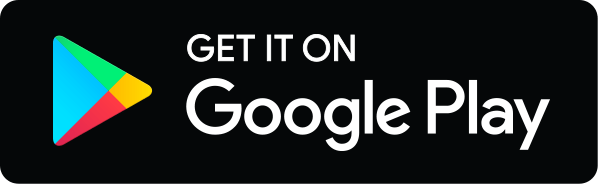