Spatial resolution is the ability to distinguish two closely situated objects as separate. Spatial resolution includes axial resolution (the ability to distinguish two objects at different depths along the path of the ultrasound beam) and lateral resolution (the ability to distinguish two objects that are side by side perpendicular to the ultrasound beam). Higher transducer frequencies increase spatial resolution but penetrate poorly into the tissues. Lower transducer frequencies penetrate deeper into the tissues at the expense of lower spatial resolution. Spatial resolution and beam penetration have to be balanced when choosing the transducer frequency.
Examples: A high-frequency (6–13 MHz) ultrasound transducer is used to image superficial structures such as the brachial plexus in the interscalene groove or supraclavicular fossa. A lower-frequency transducer (5–10 MHz) is suitable for slightly deeper structures such as the brachial plexus in the infraclavicular fossa, and a low-frequency transducer (2–5 MHz) is used to image deep structures such as the lumbar paravertebral region or the sciatic nerve. High-frequency (6–13 MHz) linear transducers with a small footprint (25–26 mm) are particularly suited for regional blocks in young children.
Scans can be performed in the transverse (axial) or longitudinal plane. During a transverse scan, the transducer is oriented at right angles to the long axis of the target, producing a cross-sectional display of the structures (Fig. 1–1A). During a longitudinal (sagittal) scan, the transducer is oriented parallel to the long axis of the target (eg, a blood vessel or nerve) (Fig. 1–1B). During USGRA, ultrasound scans are most commonly performed in the transverse plane in order to easily visualize the nerves, the adjacent structures, and the circumferential spread of the local anesthetic.
The ultrasound image must be correctly oriented in order to accurately identify the anatomical relationships of the various structures on the display monitor. Ultrasound transducers have an orientation marker (eg, a groove or a ridge) on one side of the transducer, which corresponds to a marker on the monitor (eg, a dot or logo) (Fig. 1–2). There are no accepted standards on how to orient a transducer, but it is common to have the orientation marker on the transducer directed cephalad when performing a longitudinal scan, and directed towards the right side of the patient when performing a transverse scan (Fig. 1–3). In this way, the monitor “marker” should be at the upper-left corner of the screen representing the cephalad end during a longitudinal scan, or the right side of the patient during a transverse scan (Fig. 1–3). The top of the monitor represents superficial structures, and the bottom of the monitor deep structures.
The image should be optimized by adjusting the depth, focal zone, and gain. Imaging depth affects temporal resolution (the ability to accurately depict moving structures) and should be reduced to the smallest field of view (FOV) that is practical. The focal zone should be positioned at the region of interest to increase lateral resolution at that site. Reducing the total number of focal zones also improves temporal resolution. Finally, the time gain compensation (TGC) and overall gain should be adjusted to produce an image with appropriate brightness. The TGC is usually adjusted with the near field gain turned down and the far field gain turned up in steady progression to adjust for beam attenuation with depth.
Certain terms are frequently used to describe the sonographic appearance of musculoskeletal structures (Fig. 1–4):
Isoechoic: The structure is of the same brightness or echogenicity as the surrounding tissues.
Hyperechoic: The structure is bright.
Hypoechoic: The structure is dark but not completely black.
Anechoic: The structure has no echoes and appears completely black.
Contrast resolution is the ability to distinguish subtle differences in echogenicity between two adjacent structures.
During USGRA, the block needle can be visualized in its short axis (out-of-plane approach) (Fig. 1–5) or long axis (in-plane approach) (Fig. 1–6). In the out-of-plane approach, the needle is initially outside the plane of imaging and therefore not visible. The needle only becomes visible when it crosses the plane of imaging and is seen as an echogenic dot on the monitor (Fig. 1–5). It is important to note that this echogenic dot may not represent the tip of the needle because it is a short-axis view. In the in-plane approach the needle is inserted along the plane of imaging and therefore both the shaft and tip of the needle are visible on the monitor (Fig. 1–6).
Both approaches are commonly used, and there are no data showing that one is better than the other. Pros and cons for both methods have been debated. Proponents of the out-of-plane approach have had great success with this method and claim that it causes less needle-related trauma and pain because the needle is advanced through a shorter distance to the target. However, critics of the out-of-plane approach express concerns that the inability to reliably visualize the needle and using tissue movement as a surrogate marker to locate the needle tip during a procedure can lead to complications. The needle is better visualized in the in-plane approach, but this requires good hand–eye coordination, and reverberation artifacts from the shaft of the needle can be problematic. Moreover, there are claims that the in-plane approach also causes more discomfort in awake patients because longer needle insertion paths are required.
Having an adequate FOV during USGRA is important because it not only allows one to visualize the “target,” but also the neighboring structures (eg, blood vessel, pleura, etc.) that one wishes to avoid injury to. Linear array transducers have a narrow FOV, whereas curved array transducers have a divergent ultrasound beam resulting in a wider FOV (Fig. 1–7).
Needles are best visualized when imaged perpendicular to the ultrasound beam. Needles at steep angles required for deep blocks may not be easily visualized with linear array transducers. Linear array transducers are best suited for superficial blocks (eg, axillary or interscalene brachial plexus block, femoral nerve block). Curved array transducers are more suitable for deep blocks (eg, sciatic nerve block, lumbar plexus block, and central neuraxial blocks). However, curved array transducers have reduced lateral resolution at depth due to the diverging ultrasound beam.
Other factors can also influence needle visibility. The needle is better visualized in its long axis than in its short axis, and its visibility decreases linearly with smaller needle diameters. The needle tip is better visualized when in its long axis for shallow angles of insertion (less than 30 degrees), and in its short axis when the angle of insertion is steep (greater than 60 degrees). This is also true when the needle is inserted with its bevel facing the ultrasound transducer. To overcome the effect of angle on needle visibility, some high-end ultrasound machines allow the operator to steer the ultrasound beam (beam steering) towards the needle during steep insertions. However, this requires experience, and decreases in needle visibility can still occur. Needle visibility is also enhanced in the presence of a medium-sized guide wire. Priming a needle with saline or air, insulating it, or inserting a stylet prior to insertion does not improve visibility.
We believe that the anesthesiologist’s skill in aligning the needle along the plane of imaging is by far the most important variable influencing needle visibility because minor deviations of even a few millimeters from this plane can result in an inability to visualize the needle. Even with experience, needle tip visibility is a problem when performing blocks at depth, in areas that are rich in fatty tissue, and in the elderly. Under such circumstances gently jiggling (rapid in-and-out movement) the needle and observing tissue movement or performing a test injection of saline or 5% dextrose (1–2 mL) and observing tissue distention can help locate the position of the needle tip. The preference is for 5% dextrose for the latter when nerve stimulation is used because it does not increase the electric current required to elicit a motor response.
Anisotropy, or angular dependence, is a term used to describe the change in echogenicity of a structure with a change in the angle of insonation of the incident ultrasound beam (Fig. 1–8). It is frequently observed during scanning of nerves, muscles, and tendons. This occurs because the amplitude of the echoes returning to the transducer varies with the angle of insonation. Nerves are best visualized when the incident beam is at right angles; small changes in the angle away from the perpendicular can significantly reduce their echogenicity. Therefore, during USGRA the transducer should be tilted from side to side to minimize anisotropy and optimize visualization of the nerve. Although poorly understood, different nerves also exhibit differences in anisotropy; this may be related to the internal architecture of the nerve.
Peripheral nerves consist of hypoechoic nerve fascicles surrounded by hyperechoic connective tissue and have a “honeycomb” appearance in the transverse axis (Fig. 1–9). They have a fibrillar appearance in the longitudinal axis with fine parallel hyperechoic lines separated by fine hypoechoic lines. Generally, nerves appear hyperechoic, but the appearance can vary depending on the surrounding structures. For example, nerves appear hyperechoic when surrounded by hypoechoic muscle, but can appear hypoechoic when surrounded by hyperechoic fat. The echogenicity of a nerve may also vary depending on the location where it is scanned; for example, the brachial plexus nerves appear hypoechoic at the interscalene groove, but are hyperechoic at the infraclavicular fossa and axilla. The exact reason for this is not clear, but may be related to the relative proportion of neural and connective tissue within the nerve. The ratio of neural to non-neural tissue content within the epineurium of the nerve increases from 1:1 in the interscalene/supraclavicular fossa to 1:2 in the mid-infraclavicular/paracoracoid regions. Nerve motion can also be demonstrated on dynamic ultrasound imaging.
Tendons are hyperechoic with a fibrillar pattern on longitudinal scans. Tendons are more hyperechoic than nerves and move more than adjacent nerves when the corresponding muscle is contracted or passively stretched.
Muscle fiber bundles are hypoechoic. The separating and surrounding connective tissue perimysium and epimysium are hyperechoic (Fig. 1–9). Muscle fibers converge to become tendons or aponeuroses.
Subcutaneous fat lobules appear as round to oval hypoechoic nodules that are separated by fine hyperechoic septa. They are slightly compressible and appear similar on transverse and longitudinal scans.
Bone reflects most of the ultrasound beam. Therefore, the bone surface appears hyperechoic on ultrasound with posterior acoustic shadowing, and possibly posterior reverberation, distal to it (Fig. 1–10).
FIGURE 1–10
Echogenicity of bone, pleura and lung at the intercostal space. Note the acoustic shadow deep to the rib.

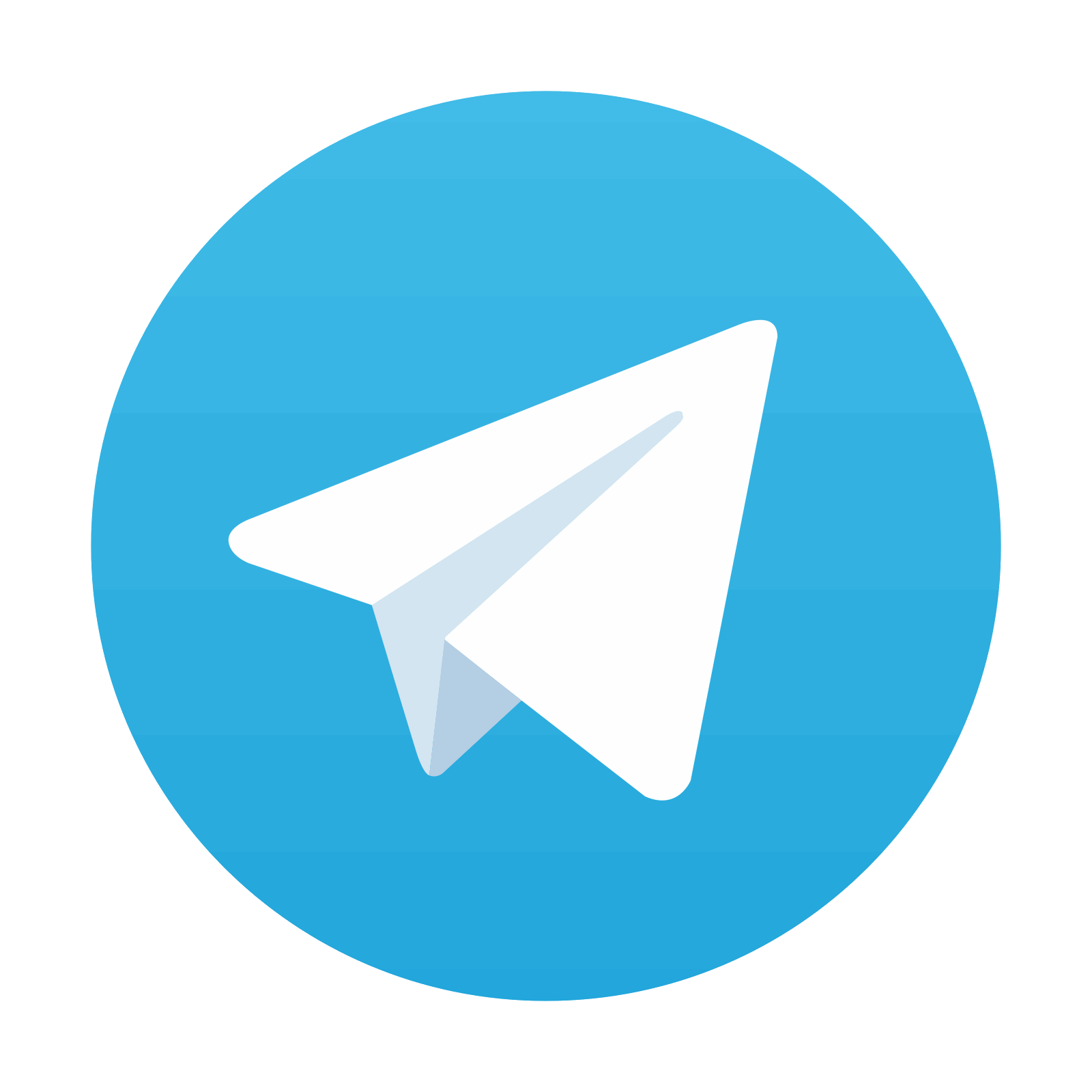
Stay updated, free articles. Join our Telegram channel

Full access? Get Clinical Tree
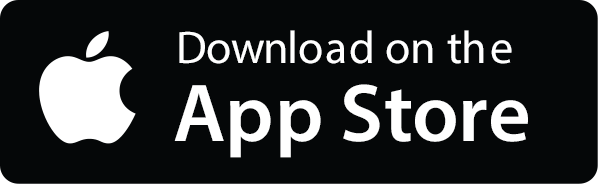
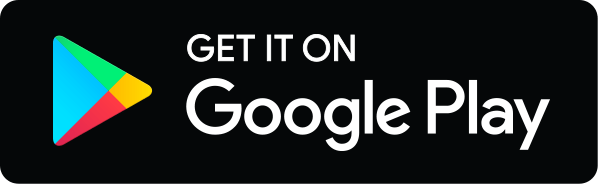
