
Body fluids can be divided into intracellular and extracellular fluid, depending on their location relative to the cell membrane (see Fig. 1-1).1 Approximately two-thirds of the total body fluid in an adult are contained inside the estimated 100 trillion cells of the body. The fluid in these cells, despite individual differences in constituents, is collectively designated intracellular fluid. The one-third of fluid outside the cells is referred to as extracellular fluid. Extracellular fluid is divided into interstitial fluid and plasma (intravascular fluid) by the capillary membrane (see Fig. 1-1).1
Interstitial fluid is present in the spaces between cells. An estimated 99% of this fluid is held in the gel structure of the interstitial space. Plasma is the noncellular portion of blood. The average plasma volume is 3 L, a little over half of the blood volume of 5 L. Plasma is in dynamic equilibrium with the interstitial fluid through pores in the capillaries; the interstitial fluid serving as a reservoir from which water and electrolytes can be mobilized into the circulation. Loss of plasma volume from the intravascular space is minimized by colloid osmotic pressure exerted by the plasma proteins.
Other extracellular fluid that may be considered as part of the interstitial fluid includes cerebrospinal fluid, gastrointestinal fluid (because it is mostly resorbed), and fluid in potential spaces (pleural space, pericardial space, peritoneal cavity, synovial cavities). Excess amounts of fluid in the interstitial space manifest as peripheral edema.
The normal daily intake of water (drink and internal product of food metabolism) by an adult averages 2.5 L, of which about 1.5 L is excreted as urine, 100 mL is lost in sweat, and 100 mL is present in feces. All gases that are inhaled become saturated with water vapor (47 mm Hg at 37°C). This water vapor is subsequently exhaled, accounting for an average daily water loss through the lungs of 300 to 400 mL. The water content of inhaled gases decreases with decreases in ambient air temperature such that more endogenous water is required to achieve a saturated water vapor pressure at body temperature. As a result, insensible water loss from the lungs is greatest in cold environments and least in warm temperatures. The remaining 400 mL is lost by diffusion through the skin. This is insensible water loss, not perceived as sweat. Insensible water loss is limited by the mostly impermeable layer of the skin (cornified squamous epithelium). When the cornified layer is removed or interrupted, as after burn injury, the loss of water through the skin is greatly increased.
Blood Volume
Blood contains extracellular fluid, the plasma, and intracellular fluid, mostly held in erythrocytes. The body has multiple systems to maintain intravascular fluid volume, including renin-angiotensin system, and arginine vasopressin (antidiuretic hormone) that increase fluid reabsorption in the kidney and evoke changes in the renal tubules that lead to restoration of intravascular fluid volume (see Chapter 17).
The average blood volume of an adult is 5 L, comprising about 3 L of plasma and 2 L of erythrocytes. These volumes vary with age, weight, and gender. For example, in nonobese individuals, the blood volume varies in direct proportion to the body weight, averaging 70 mL/kg for lean men and women. The greater the ratio of fat to body weight, however, the less is the blood volume in milliliter per kilogram because adipose tissue has a decreased vascular supply. The hematocrit or packed cell volume is approximately the erythrocyte fraction of blood volume. The normal hematocrit is about 45% for men and postmenopausal women and about 38% for menstruating women, with a range of approximately ± 5%.
Constituents of Body Fluid Compartments
The constituents of plasma, interstitial fluid, and intracellular fluid are identical, but the quantity of each substance varies among the compartments (Fig. 1-2).2 The most striking differences are the low protein content in interstitial fluid compared with intracellular fluid and plasma and the fact that sodium and chloride ions are largely extracellular, whereas most of the potassium ions (approximately 90%) are intracellular. This unequal distribution of ions results in establishment of a potential (voltage) difference across cell membranes.

The constituents of extracellular fluid are carefully regulated by the kidneys so that cells are bathed in a fluid containing the proper concentrations of electrolytes and nutrients. The normal amount of sodium and potassium in the body is about 58 mEq/kg and 45mEq/kg, respectively (note that normal serum level of sodium is 137 to 142 mEq/L and potassium is 3.5 to 5.5 mEq/L, reflecting the intracellular and extracellular predominance of each electrolyte). Trauma is associated with progressive loss of potassium through the kidneys due in large part to the increased secretion of vasopressin and in variable part (depending on the type of surgery) to the role of nasogastric suctioning and direct potassium loss. For example, a patient undergoing surgery excretes about 100 mEq of potassium in the first 48 hours postoperatively and, after this period, about 25 mEq daily. Plasma potassium concentrations are not good indicators of total body potassium content because most potassium is intracellular. There is a correlation, however, between the potassium and hydrogen ion content of plasma; the two are increasing and decreasing together.
Osmosis
Osmosis is the movement of water (solvent molecules) across a semipermeable membrane from a compartment in which the nondiffusible solute (ion) concentration is lower to a compartment in which the solute concentration is higher (Fig. 1-3).3 The lipid bilayer that surrounds all cells is freely permeable to water but is impermeable to ions. As a result, water rapidly moves across the cell membrane to establish osmotic equilibration, which happens almost instantly.

Cells control their size by controlling intracellular osmotic pressure. The maintenance of a normal cell volume and pressure depends on sodium–potassium adenosine triphosphatase (ATPase) (sodium–potassium exchange pump), which maintains the intracellular–extracellular ionic balance by removing three sodium ions from the cell for every two potassium ions brought into the cell. The sodium–potassium pump also maintains the transmembrane electrical potential and the sodium and potassium concentration gradients that power many cellular processes, including neural conduction.
The osmotic pressure exerted by nondiffusible particles in a solution is determined by the number of particles in the solution (degree of ionization) and not the type of particles (molecular weight) (see Fig. 1-3).3 Thus a 1-mol solution of glucose or albumin and 0.5-mol solution of sodium chloride exert the same osmotic pressure, because the sodium chloride exists as independent sodium and chloride ions, each having a concentration of 0.5 mol. Osmole is the unit used to express osmotic pressure in solutes, but the denominator for osmolality is kilogram of water. Osmolarity is the correct terminology when osmole concentrations are expressed in liters of body fluid (e.g., plasma) rather than kilogram of water (osmolality). Because it is much easier to express body fluids in liters of fluid rather than kilograms of free water, almost all physiology calculations are based on osmolarity. Plasma osmolarity is important in evaluating dehydration, overhydration, and electrolyte abnormalities.
Normal plasma has an osmolarity of about 290 mOsm/L. All but about 20 mOsm of the 290 mOsm in each liter of plasma are contributed by sodium ions and their accompanying anions, principally chloride and bicarbonate. Proteins normally contribute <1 mOsm/L. The major nonelectrolytes of plasma are glucose and urea, and these substances can contribute significantly to plasma osmolarity when hyperglycemia or uremia is present, as suggested by the standard calculation of plasma osmolarity:
Plasma osmolarity = 2 (Na+) + 0.055 (glucose) + 0.36 (blood urea nitrogen).
Tonicity of Fluids
Packed erythrocytes must be suspended in isotonic solutions to avoid damaging the cells (e.g., Fig. 1-4).4 A 0.9% solution of sodium chloride is isotonic and remains so because there is no net movement of the osmotically active particles in the solution into cells, and the particles are not metabolized. A solution of 5% glucose in water is initially isotonic when infused, but glucose is metabolized, so the net effect is that of infusing a hypotonic solution. Lactated Ringer solution plus 5% glucose is initially hypertonic (about 560 mOsm/L), but as glucose is metabolized, the solution becomes less hypertonic.

Fluid Management
The goal of fluid management is to maintain normovolemia and thus hemodynamic stability. Crystalloids consist of water; electrolytes; and, occasionally, glucose that freely distribute along a concentration gradient between the two extracellular spaces. After 20 to 30 minutes, an estimated 75% to 80% of an isotonic saline or a lactate-containing solution will have distributed outside the confines of the circulation, thus limiting the efficacy of these solutions in treating hypovolemia. Indeed, the ability of crystalloids to restore perfusion in the microcirculation is doubtful.5
Hypotonic intravenous fluids equilibrate with extracellular fluid, causing it to become hypotonic with respect to intracellular fluid. When this occurs, osmosis rapidly increases intracellular water, causing cellular swelling. Increased intracellular fluid volume is particularly undesirable in patients with intracranial mass lesions or increased intracranial pressure. Protection from excessive fluid accumulation in the interstitium (extravascular lung water) is mediated by lymphatic flow, which can increase as much as 10-fold.
Hypertonic saline solutions (7.5% sodium chloride) have been useful for rapid intravascular fluid repletion during resuscitation as during hemorrhagic and septic shock. Hypertonic saline solutions compare favorably with mannitol for lowering intracranial pressure.6 The primary effect of hypertonic saline solutions (increase systemic blood pressure and decrease intracranial pressure) most likely reflects increased intravascular fluid volume because of fluid shifts and movement of water away from uninjured regions of the brain. The use of hypertonic saline solutions is viewed as short-term treatment as hypertonicity and hypernatremia are likely with sustained administration. Furthermore, patients with hypotension due to traumatic brain injury who received prehospital resuscitation with hypertonic saline solutions have similar neurologic outcomes to those treated with conventional fluids when assessed 6 months after the initial injury.7
Dehydration
Loss of water by gastrointestinal or renal routes or by diaphoresis (excessive sweating) is associated with an initial deficit in extracellular fluid volume. At the same instant, intracellular water passes to the extracellular fluid compartment by osmosis, thus keeping the osmolarity in both compartments equal despite decreased absolute volume (dehydration) of both compartments. The ratio of extracellular fluid to intracellular fluid is greater in infants than adults, but the absolute volume of extracellular fluid is obviously less, explaining why dehydration develops more rapidly and is often more severe in the very young. Clinical signs of dehydration are likely when about 5% to 10% (severe dehydration) of total body fluids have been lost in a brief period of time. Physiologic mechanisms can usually compensate for acute loss of 15% to 25% of the intravascular fluid volume, whereas a greater loss places the patient at risk for hemodynamic decompensation.
Cell Structure and Function
The basic living unit of the body is the cell. It is estimated that the entire body consists of 100 trillion or more cells, of which (amazingly) about 25 trillion are red blood cells.4 Each organ is a mass of cells held together by intracellular supporting structures. A common characteristic of all cells is dependence on oxygen to combine with nutrients (carbohydrates, lipids, proteins) to release energy necessary for cellular function. Almost every cell is within 25 to 50 µm of a capillary, assuring prompt diffusion of oxygen to cells. All cells exist in nearly the same composition of extracellular fluid (milieu interieur or interior milieu, the extracellular fluid environment), and the organs of the body (lungs, kidneys, gastrointestinal tract) function to maintain a constant composition (homeostasis) of extracellular fluid.
Cell Anatomy
The principal components of cells include the nucleus (except for mature red blood cells), and the cytoplasm, which contains structures known as organelles (Fig. 1-5).8 The nucleus is separated from the cytoplasm by a nuclear membrane, and the cytoplasm is separated from surrounding fluids by a cell (plasma) membrane. The membranes around the cell, the nucleus, and organelles are lipid bilayers.

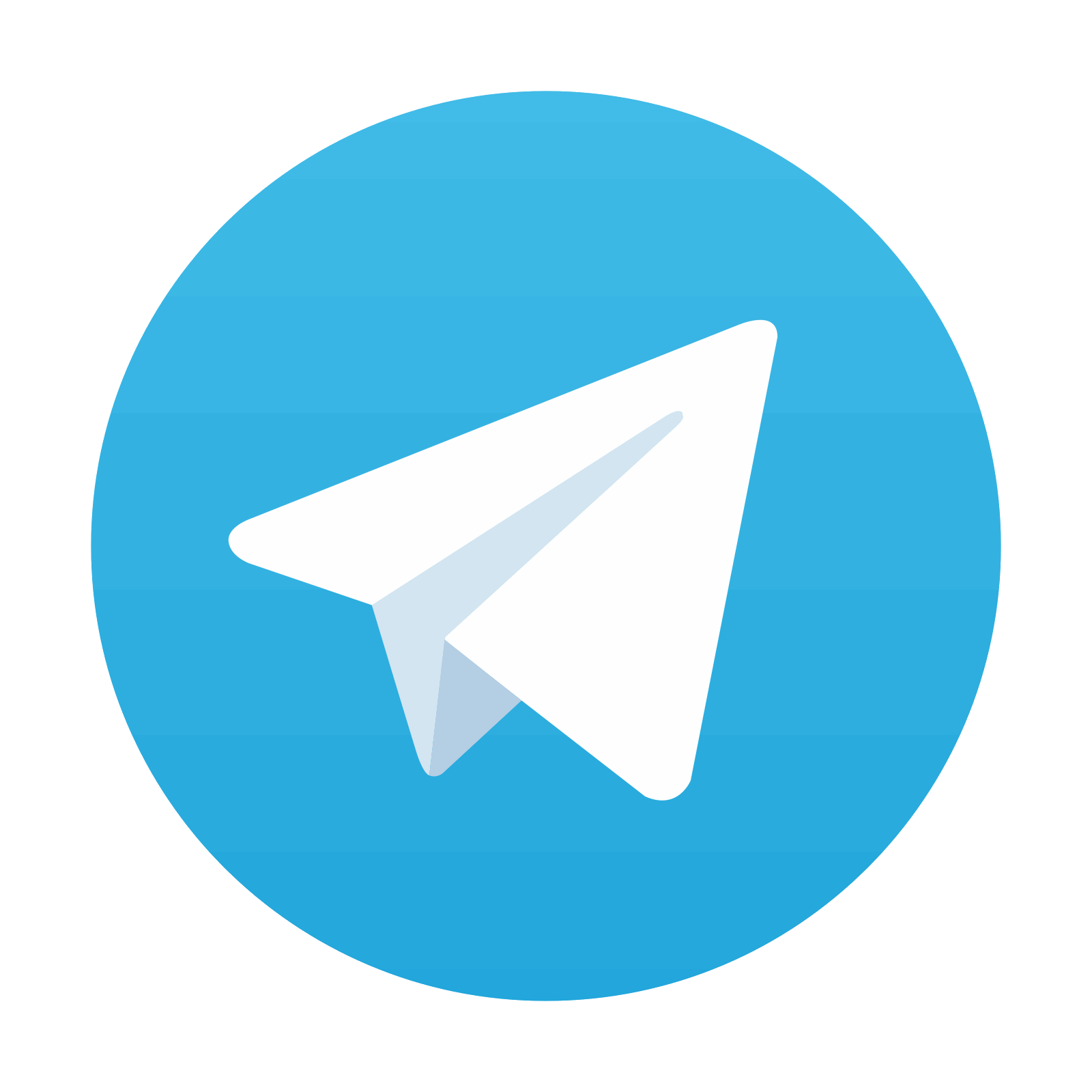
Stay updated, free articles. Join our Telegram channel

Full access? Get Clinical Tree
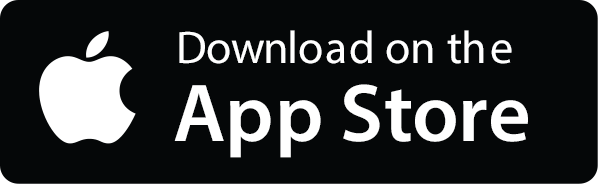
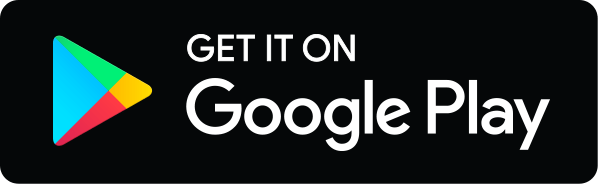