Suspected ischemic stroke is one of the most common indications for neurological imaging in the ED. Initial imaging includes NECT and/or MRI. Stroke MRI protocols may be completed in 10 minutes if resources permit, including perfusion imaging. CT findings of acute stroke may include “hyperdense MCA sign” or “dot sign”, which is due to thrombus within the proximal MCA or Sylvian MCA branch, “insular ribbon sign” due to obscuration of the insular cortex due to edema, and parenchymal hypodensity. Most commonly however, NECT will be normal in the initial stages of ischemic stroke. Later findings include the development of hypodensity, gyral swelling and sulcal effacement. Hemorrhagic transformation is most common after 1-2 days.
Many centers include CTA or CT Perfusion (CTP) immediately following NECT. CTA allows assessment of stenoses, dissections, vasculitis, or intracranial large vessel thrombus. CTP assesses cerebral blood flow and identifies the core infarct and surrounding tissue-at-risk (penumbra).
DWI is the most sensitive MR sequence for acute ischemia. Findings of “restricted diffusion” are a bright signal on DWI with a corresponding dark signal on ADC. Findings become positive within minutes of ischemia and remain positive for 10 days. MRA can be obtained as an alternative to CTA.
MR perfusion-weighted imaging (PWI) can be obtained with contrast analogous to CTP. In combination with DWI, mismatches between DWI infarct and PWI ischemic tissue can be used to determine if any salvageable penumbra tissue exists.
An ischemic stroke results from brain hypoperfusion due to thrombus, emboli, dissection, stenosis, or hypotension. Patients with ischemic stroke may present with the sudden onset of focal neurologic deficits such as motor weakness, sensory deficits, or speech difficulties. Current NINDS guidelines recommend that the ED physician complete the initial assessment and NECT within 25 minutes of patient arrival to maximize potential benefit for reperfusion therapy.
Figure 2.1 ▪ Ischemic Stroke.






A: NECT shows subtle loss of the left insular ribbon (region between the lentiform nuclei and Sylvian fissure), sulcal effacement, and mild edema that blurs the gray-white interface. Findings suggest acute MCA infarction. B: NECT shows hyperdensity in the region of the left middle cerebral artery (“hyperdense MCA sign”) (arrow). C: Axial CTA confirms the thrombus occluding the proximal (M1) segment of the left MCA with little collateral flow distally (arrow). D: Conventional angiogram of left carotid injection shows cut-off of middle cerebral artery on left corresponding to the CTA (arrow). Normal filling is seen in the ACA. E: Angiogram of left carotid injection following intra-arterial tPA and MERCI thrombus removal device intervention shows restoration of blood flow into the left MCA vessels. F: Schematic diagram of cerebrovascular territories.
Figure 2.2 ▪ CT Perfusion Obtained Immediately after NECT.




A: Mean transit time (MTT) map at level of basal ganglia shows prolonged transit through the right MCA territory indicating sluggish blood flow in this region. B: CT Perfusion cerebral blood flow (CBF) map shows decreased blood flow in corresponding MCA territory. C: CT Perfusion cerebral blood volume (CBV) map shows markedly decreased delivery of blood volume to this region. D: CT Perfusion image based on calculations indicates area of infarct (red) and penumbra (green). The penumbra is the only region that has potential for salvage.
The most recent American Heart Association guidelines recommend that IV tPA may be given up to 4.5 hours after the onset of ischemic stroke. It is crucial to obtain imaging studies prior to the administration of thrombolytics as acute hemorrhage must be ruled out prior to treatment. The more rapidly that studies can be obtained, the sooner treatment decisions can be made, a critical element of emergent stroke care as the earlier the treatment is begun the higher the odds ratio is for positive clinical outcome.
Edema related to an infarct typically involves gray and white matter. If edema only involves white matter, consider alternative diagnoses such as tumor.
Diffusion MRI becomes positive within minutes of acute ischemic stroke, but it usually takes at least 4.5 hours for FLAIR sequences to demonstrate hyperintensity.
A dense MCA sign corresponds to a significant acute clot in the proximal cerebral vasculature. Patients with this radiographic finding may benefit from neurointerventional procedures to aid in clot lysis or mechanical clot removal in order to reperfuse ischemic brain tissue.
Figure 2.3 ▪ Ischemic Stroke.



A: 80-year-old female NECT shows hyperdensity in right MCA (hyperdense MCA sign) (arrow). B: Axial CTA MIP shows cutoff of right MCA indicating thrombus. C: DWI MRI shows restricted diffusion in the right MCA territory involving the basal ganglia, right frontal, and right temporal lobes indicating acute infarction.
Hemorrhagic strokes are acute bleeding events in the brain and may result in the rapid development of neurological impairment, respiratory failure, and death. In suspected acute stroke, NECT of the brain or MRI is necessary for treatment planning. MRI with gradient-based sequences is superior to CT for detection of hemorrhage, but is less often used in most centers due to its time, expense, and availability.
Acute parenchymal hemorrhage on CT will be hyperdense (bright). MRI of hyperacute (less than 12 hours) blood will be T1 isointense, T2 hyperintense, and hypointense on T2*/GRE. Common locations for hypertensive hemorrhage are the basal ganglia, thalamus, pons, and cerebellum. Occasionally blood can seep into the adjacent ventricular system. Hemorrhages in other locations suggest an alternate etiology, such as vascular malformation (AVM, cavernous malformation), tumor, or amyloid angiopath.
Hemorrhagic stroke accounts for about 10-15% of all strokes, with a higher mortality rate when compared to ischemic stroke. It is important to ask about usage of any anti-platelet agents or warfarin when obtaining history. Patients with acute ICH may have additional clinical symptoms that go along with focal neurological deficits such as headache, seizures, persistent nausea and vomiting, altered mental status, and cardiopulmonary failure. The mechanism of ICH is thought to be leakage from small intracerebral arteries damaged by long-standing high blood pressure. Other causes include cerebral amyloidosis, iatrogenic anticoagulation, bleeding diathesis, and use of cocaine. In addition to the focally injured area, there is additional pressure produced by the mass effect of the hematoma and bleeding that subsequently may lead to rapid increases in intracranial pressure. Decompressive craniotomy may be required in some instances.
Morbidity and mortality rates are higher for hemorrhagic strokes than ischemic strokes.
Acute respiratory failure and need for emergent airway management are much more common with acute hemorrhagic stroke than with ischemic stroke.
NECT is the first-line imaging for the detection of acute hemorrhagic stroke.
Atraumatic subarachnoid hemorrhage (SAH) usually results from a ruptured aneurysm, 90% of which arise from the circle of Willis. NECT is the initial choice for imaging patients with suspected SAH. Blood appears as a hyperdensity within the basilar cisterns and sulci but may also be commonly seen along the sylvian fissure and interpenduncular fossa. MRI is nearly as sensitive as CT for hyperacute (less than 12 hours) SAH detection. After 24 hours, MRI is superior to CT for detection of blood products as the sensitivity of CT for SAH detection drops to 93% by 24 hours and 50% at 7 days as the SAH is cleared by the CSF circulation. FLAIR demonstrates SAH as a bright signal along the cerebral sulci or within the basilar cisterns and T2*/GRE sequences show areas of a dark signal within the sulci.
The location of SAH may suggest where the aneurysm is located. Blood in the anterior interhemispheric fissure suggests aneurysm of the ACOM (anterior communicating) artery. Sylvian fissure blood correlates with the middle cerebral artery (MCA), and blood located in the posterior fossa correlates with a posterior circulation aneurysm (basilar artery or PICA).
Figure 2.6 ▪ ACOM Aneurysm with SAH.



A: NECT shows dilation of temporal horns with blood in the left side. Subarachnoid blood is within the quadrigeminal and ambient cisterns (arrow). A round gray object is seen in the interhemispheric fissure representing a large ACOM aneurysm (arrowhead). B: Magnified frontal angiogram of carotid injection shows saccular aneurysm arising from the anterior communicating artery. C: (In a different patient): Axial NECT shows small amount of SAH in the left Sylvian fissure.
Figure 2.7 ▪ Atraumatic Subarachnoid Hemorrhage.


A: NECT (different patient from images 8a-8d) shows extensive SAH throughout the basilar cisterns, prepontine cistern, and anterior interhemispheric fissure. B: NECT shows small focus of SAH in the interpeduncular fossa. Angiography was negative. Patient was diagnosed with a perimesencephalic nonaneurysmal SAH.
An entity called perimesencephalic nonaneurysmal SAH exists in which SAH is present in the prepontine cistern, interpeduncular fossa, or ambient cisterns; however, angiography will fail to reveal an aneurysm. The source is thought to be venous and the risk of re-bleeding is exceedingly low with a benign clinical course.
Patients with SAH often present with sudden onset of severe headache “thunderclap headache” accompanied by possible syncope, nausea, vomiting, photophobia, and focal neurological deficits. Meningeal irritation or neck stiffness may be a sign of a ruptured aneurysm. Grading of SAH depends on the patient’s neurological state, with a higher mortality rate associated with more profound disability on presentation. Treatment is based upon the availability of interventional neuroradiology/endovascular neurosurgery. Some studies have shown lower morbidity and mortality with coil embolization versus surgical clipping. SAH can cause hydrocephalus by obstructing the arachnoid villi and interrupting the normal flow of CSF. After several days, SAH can also cause dangerous vasospasm of the cerebral vessels.
If there is high clinical suspicion for SAH in the face of a negative CT scan, a lumbar puncture must be performed to assess for RBC’s and xanthrochromia in order to rule out hemorrhage. There is emerging literature to suggest a NECT plus CT angiography may become a suitable approach to rule out SAH, but this method has not been proven to date.
Emergent NECT is the initial preferred imaging modality for patients with suspected SAH with nearly 100% sensitivity if performed within 12 hours.
If NECT reveals SAH, CTA or cerebral angiography is the next step. If this is normal, a repeat CTA in 7-14 days is recommended to re-assess for a bleeding source. If the 2nd CTA is negative, an MRI is recommended to assess for vascular malformations of the brain, brainstem, or spinal cord.
If NECT reveals no SAH, lumbar puncture is the next step. If lumbar puncture reveals xanthochromia or elevated red-cell count, CTA or cerebral angiography is indicated.
An aneurysm is a focal outpouching of a cerebral artery at an area of focal weakness that is contained by the media and adventitia. These intracranial aneurysms affect only part of the vessel circumference and are also called “berry” or “saccular” aneurysms. Fusiform aneurysms are long areas of dilation of a vessel. Mycotic aneurysms are an entity due to infection (bacterial or fungal). They are often found in the distal intracranial vasculature near branch-points. They lack a true vessel wall and are technically pseudoaneurysms. Because of this, they are at a high risk of rupture.
The initial method for evaluation of intracranial aneurysms is CTA or MRA. CTA offers greater spatial resolution compared to MRA. If CTA is equivocal and concern remains high, catheter angiography is needed as it offers resolution unmatched by CT or MRI. Aneurysms can produce hyperintense or hypointense signals on MRI studies depending on the specific flow characteristics and pulse sequences (T1, T2, etc.) used. The typical aneurysm lumen with rapid flow is visible as a well-delineated mass that shows high-velocity signal loss on T1- and T2-weighted images. Signal heterogeneity may be observed if turbulent flow in the aneurysm is present. Most ruptured aneurysms are in the 4-8 mm size range. An aneurysm greater than 25 mm in size is termed a “giant” aneurysm. Digital subtraction angiography is the gold standard for diagnosis as this modality affords the most accurate assessment of aneurysm size, configuration (saccular or fusiform), and neck evaluation (narrow or broad). Coil embolization treatment can be performed during the same procedure.
Most unruptured aneurysms are asymptomatic and are often incidentally discovered. If symptomatic, unruptured aneurysms are often discovered due to the mass effect they exert on surrounding nerves or structures. Rupture of an aneurysm is the cause of 80-90% of atraumatic subarachnoid hemorrhage.
Figure 2.8 ▪ MCA Aneurysm.





A: A 77-year-old-male presenting with right CN III palsy. T2 MR shows a large round lesion in the right parasellar region. B: T1+C MR, shows pulsation artifact and enhancement within the lesion, confirming an aneurysm. The pulsation artifact is due to the movement of blood. C: Axial CTA shows the large aneurysm that is actually arising from the ICA terminus. On this image, the aneurysm is seen displacing the PCOM artery. The 3rd cranial nerve runs adjacent to the PCOM, and is being impinged upon by the aneurysm causing a 3rd nerve palsy. D: Frontal angiogram from right carotid artery injection demonstrates a large aneurysm sac filling with contrast confirming the findings seen on CT/MRI. E: Frontal angiogram following endovascular coil embolization of the large aneurysm with minimal residual filling of the neck of the aneurysm.
Figure 2.9 ▪ Fusiform Basilar Artery Aneurysm.


A: NECT in a 39-year-old male presenting with severe hypertension shows a markedly enlarged basilar artery anterior to the pons (size is over twice that of internal carotid arteries). B: Sagittal CTA shows long segment fusiform aneurysm of the basilar artery without rupture.
A classic symptom of an unruptured aneurysm is a PCOM aneurysm causing painful third nerve palsy with a “blown pupil.” The oculomotor nerve (CN III) passes directly lateral to the PCOM and may be compressed by an enlarging aneurysm. The PCOM branches off the ICA as it enters the brain. The junction of the PCOM and the ICA is the most common site for a PCOM aneurysm. Coil embolization or surgical clipping are the treatments of unruptured aneurysms.
Unruptured aneurysms vary in their risk of rupture based on their size and location. The larger the aneurysm, the higher the risk of rupture, with anterior circulation aneurysms having a lower 5-year risk of rupture than posterior circulation aneurysms.
Patients may report headache on the same side as the aneurysm. Painful compression of the third nerve resulting in ipsilateral 3rd nerve palsy is a common symptom and may be the only deficit in patients harboring a PCOM aneurysm.
CTA or MRA is the preferred initial imaging. Angiography remains the gold standard. Due to the invasive nature and risks of conventional angiography, noninvasive imaging is pursued first.
CTA and MRA can reliably detect aneurysms 3 mm or larger with 90% sensitivity.
15-20% of patients will have multiple aneursysms on imaging studies.
Figure 2.10 ▪ MCA Aneurysm.



A: Axial T1 MRI without contrast demonstrates a large mass near the inferior left basal ganglia containing areas of bright signal (thrombus or slow-moving blood). The horizontal pulsation artifact indicates that this is an aneurysm as a tumor would not cause a MRI pulsation artifact. B: Axial T2 image shows mixed bright and dark T2 signal within this large aneurysm representing areas of flowing blood (dark signal) and slow-moving blood or thrombus (brighter signal). C: Frontal angiogram demonstrates a giant aneurysm arising from the ICA terminus/MCA.
Arteriovenous malformations (AVM) are masses of abnormal blood vessels characterized by direct connection of arteries to veins without the normal intervening capillary network. If an AVM has not hemorrhaged, the appearance on CT is that of enlarged vessels with a density that may be only slightly higher than the adjacent brain tissue. The vessels may have the appearance of a high-density mass, with calcification present 15-20% of the time. When associated with hemorrhage, an AVM will present as a high-density mass within the brain on NECT, and acute hyperdense blood may obscure other diagnostic features. On MRI, dilated arteries and enlarged draining veins from an AVM are dark in signal on T2WI. These will enhance with contrast (CTA or MRA). The nidus may have the appearance of a “bag of worms” due to the cluster of serpentine vessels. Digital subtraction angiography remains the gold standard of diagnosis and pre-treatment planning as it allows exquisite detail of the location and number of feeding vessels, flow dynamics, and the drainage pattern.
AVMs may present with headache, acute neurologic dysfunction, and signs and symptoms as a consequence of acute intracranial hemorrhage. Slowly progressive neurological deficits may also occur and are thought to relate to siphoning of blood flow away from the adjacent brain tissue (“steal phenomenon”). Neurological deficits may be explained alternatively by the mass effect of an enlarging AVM or venous hypertension in the draining veins. Neurologic findings are rare in the absence of seizure or hemorrhage in patients with cerebral AVMs, but are more common in brainstem and deeply situated AVMs. If parenchymal hemorrhage is present, physical findings are indistinguishable from those due to intracranial hemorrhage of other causes.
Figure 2.11 ▪ Arteriovenous Malformation.


A: NECT shows a large parenchymal hemorrhage in the lateral left basal ganglia. A coarsely calcified mass is present in the left basal ganglia medial to the hemorrhage. The mass represents thrombosed chronically calcified vessels. Blood is present within the ventricles (notice hematocrit level in the occipital horns. B: Lateral cerebral angiogram of carotid injection shows a tangle of vessels arising from the middle cerebral artery territory representing the AVM.
Figure 2.12 ▪ Arteriovenous Malformation.


A: NECT in a 3-year-old male shows dystrophic calcification in the left frontal lobe from prior hemorrhage or infection. There is a poorly characterized lesion in the right parietal lobe consisting of tubular structures better seen on subsequent MRI. B: T2WI shows enlarged and tortuous abnormal vessels predominantly in and around the right parietal lobe, but also in each frontal lobe.
MRI with MRA will provide a more detailed evaluation of the brain and vasculature than CTA alone. In cases of hemorrhage, repeat imaging several weeks after the acute bleeding has subsided provides the best imaging evaluation.
Young adults with spontaneous intracranial hemorrhage should trigger a search for an AVM. Treatment can entail embolization, surgical resection, or radiation.
Peak presentation is in 3rd and 4th decades of life.
Cerebral venous sinus thrombosis results from a blood clot of the dural venous sinuses that drain blood from the brain. NECT may demonstrate hyperdensity within the dural sinuses or cortical veins, termed the “cord sign.” MRI initially demonstrates T1 isointense signal within the sinuses but in the subacute phase of illness (2-3 days after onset) T1 hyperintense signal is present. CECT or MRI may show the “empty delta sign,” which consists of enhancement of the outer venous walls, but no central enhancement due to thrombus. CTV or MRV is the preferred evaluation for sinus thrombosis. If equivocal, conventional angiography provides definitive evaluation. As time progresses, increased venous hypertension can cause intraparenchymal hemorrhage or infarction due to back pressure. Superior sagittal sinus thrombosis maybe associated with involvement of the parasagittal frontal and parietal white matter. Internal cerebral vein or straight sinus involvement can affect the thalami or basal ganglia. Asymmetric hypoplasia of the transverse sinuses is often present and can mimic stenosis if the source images are not examined carefully. Arachnoid granulations and acute extra-axial hematomas near the transverse sinus can mimic areas of thrombus. Patients with elevated hematocrit can also show falsely dense sinuses on NECT.
Ninety percent of people with dural sinus thrombosis complain of headache, while about half have stroke-like symptoms. Some patients may have memory loss or aphasia. Unless it is contraindicated, patients with dural sinus thrombosis should be anticoagulated in order to prevent extension of the blood clot and formation of new thrombus. If symptoms continue to progress despite anticoagulation, endovascular thrombolysis may be considered. The use of D-Dimer as a laboratory adjunct may be helpful in the diagnosis of acute dural sinus thrombosis. Signs of increased ICP such as papilledema and elevated opening pressure on LP may also be present.
Figure 2.13 ▪ Transverse Sinus Thrombosis.


A: NECT shows hyperdense structure in the region of the right transverse sinus (“cord sign”) (arrow). B: Coronal CTA in the venous phase shows filling of the superior sagittal and left transverse sinuses but only enhancement of the outer wall of the right transverse sinus (“empty delta sign”) (arrow).
Dural sinus thrombosis presents in a clinical manner similar to idiopathic intracranial hypertension, making radiographic imaging important.
CTV or MRV is the imaging modality most likely to definitively identify the thrombus.
One percent of strokes are due to dural sinus thrombosis. They are often misdiagnosed or missed because they can be hard to diagnose with imaging and are often not considered clinically.
Imaging pitfalls include congenital asymmetric size of venous sinuses, arachnoid granulations, and elevated hematocrit levels mimicking thrombus.
Cavernous sinus thrombosis is a rare complication of orbital, sinus, or facial infection. CTA or CECT of the brain may demonstrate an abnormally convex margin to the cavernous sinus that should normally be flat or concave. Engorgement or dilation of the superior ophthalmic vein is often seen as the pressure extends back into the draining veins. There may be narrowing of the internal carotid artery as it passes through the cavernous sinus. Orbital MRI with fat-saturation best demonstrates the anatomy of this region. MR also provides important information about the adjacent soft tissues, sinuses, orbits, and intracranial structures. Cranial nerves III, IV, V1, and V2, and VI run through the cavernous sinus. Catheter angiography may be required for final diagnosis.
Patients with cavernous sinus thrombosis often have fever, severe headache or facial pain, usually unilateral and localized to retro-orbital and frontal regions. Later developments may include ophthalmoplegia, proptosis, and diminished or absent facial sensation. Decreased level of consciousness, confusion, seizures, and focal neurologic deficits are signs of spread to the CNS. Patients may also have anisocoria or mydriasis, papilledema, and vision loss. Complications include meningoencephalitis, brain abscess, stroke, blindness, and pituitary insufficiency. Imaging is important to assess for other diagnoses that can present in a similar manner such as idiopathic orbital inflammatory syndrome/orbital pseudotumor, Wegener’s, neurosarcoidosis, sinusitis with orbital extension, tumors, or masses. Treatment includes IV antibiotics with possible surgical intervention. Secondary treatment may include corticosteroids for cranial nerve dysfunction; anticoagulation is controversial because most patients respond to antibiotics, and adverse effects of anticoagulation may exceed benefits of the therapy.
Figure 2.17 ▪ Cavernous Sinus Thrombosis.


A: Coronal CTA in a 70-year-old female with proptosis and bruit over the eye shows marked enlargement of both superior ophthalmic veins (these are commonly enlarged in both cavernous sinus thrombosis and carotid-cavernous fistula). B: Sagittal CTA shows the engorged superior ophthalmic vein.
Look for asymmetric engorgement of the cavernous sinus on the ipsilateral side of involvement on contrast-enhanced images as a sign of cavernous sinus thrombosis.
Proptosis, ptosis, chemosis, and cranial nerve palsy beginning in one eye and progressing to the other eye suggests the diagnosis.
Dilation of the superior ophthalmic veins is often seen ipsilateral to the thrombosed sinus.
CT and MR venography are not preferred over CTA or CECT for the diagnosis of cavernous sinus thrombosis even though the involved structures are part of the venous vasculature.
Moyamoya disease is a vaso-occlusive disease involving the distal internal carotid arteries and the circle of Willis and its branches. The term moyamoya disease should be reserved for an idiopathic, sometimes familial condition, which leads to characteristic intracranial vascular changes. An extensive collateral network develops from hypertrophied lenticulostriate, dural, and meningeal arteries. This collateral network of tiny vessels creates the classic angiographic picture of contrast disappearing into a cloud of tiny vessels (English translation of the Japanese word “moyamoya” is “puff of smoke”).
CTA or MRA may show the hypertrophied lenticulostriate vessels and stenotic anterior cerebral circulation but traditional catheter angiography is needed for ultimate diagnosis and pre-operative planning. Axial MRI images may show small flow voids insinuating around the basal ganglia and midbrain due to the collateral vessels. Contrasted imaging and FLAIR may show the “ivy sign” (diffuse leptomeningeal enhancement representing engorgement of the pial vasculature) due to slow flow in meningeal collaterals. Diffusion-weighted imaging (DWI) is helpful to determine acute versus chronic infarcts as these patients have often had prior insults.
Moyamoya disease, although initially described in the Japanese population, can be seen in any ethnicity. However, this condition is the most common cause of stroke in Asian children. It is believed to be due to a genetic defect in chromosomes 3p, 17q, and possibly others. Other causes of acquired moyamoya pattern are sickle cell disease, Down syndrome, brain irradiation, neurofibromatosis, tuberous sclerosis, vasculitis (inflammatory or infectious), and connective tissue disorders. If the patient is non-Asian, non-genetic etiologies should be considered. Children often present with TIA or ischemic stroke, while adults more commonly present with subarachnoid or parenchymal hemorrhage. Recognition of disease early in the course with prompt institution of therapy is critical to achieve the best outcomes in patients. Definitive treatment may include revascularization bypass surgery.
Figure 2.18 ▪ Moyamoya Disease.

Cerebral angiogram injection of carotid artery in a 27-year-old female shows terminal occlusion of the ICA with a “puff of smoke” appearance of the contrast as it dissipates into small lenticulostriate vessels (arrow). Note the enlarged meningeal and pial collaterals supplying the anterior and middle cerebral vessels peripherally (arrowheads).
Figure 2.19 ▪ Moyamoya Disease.

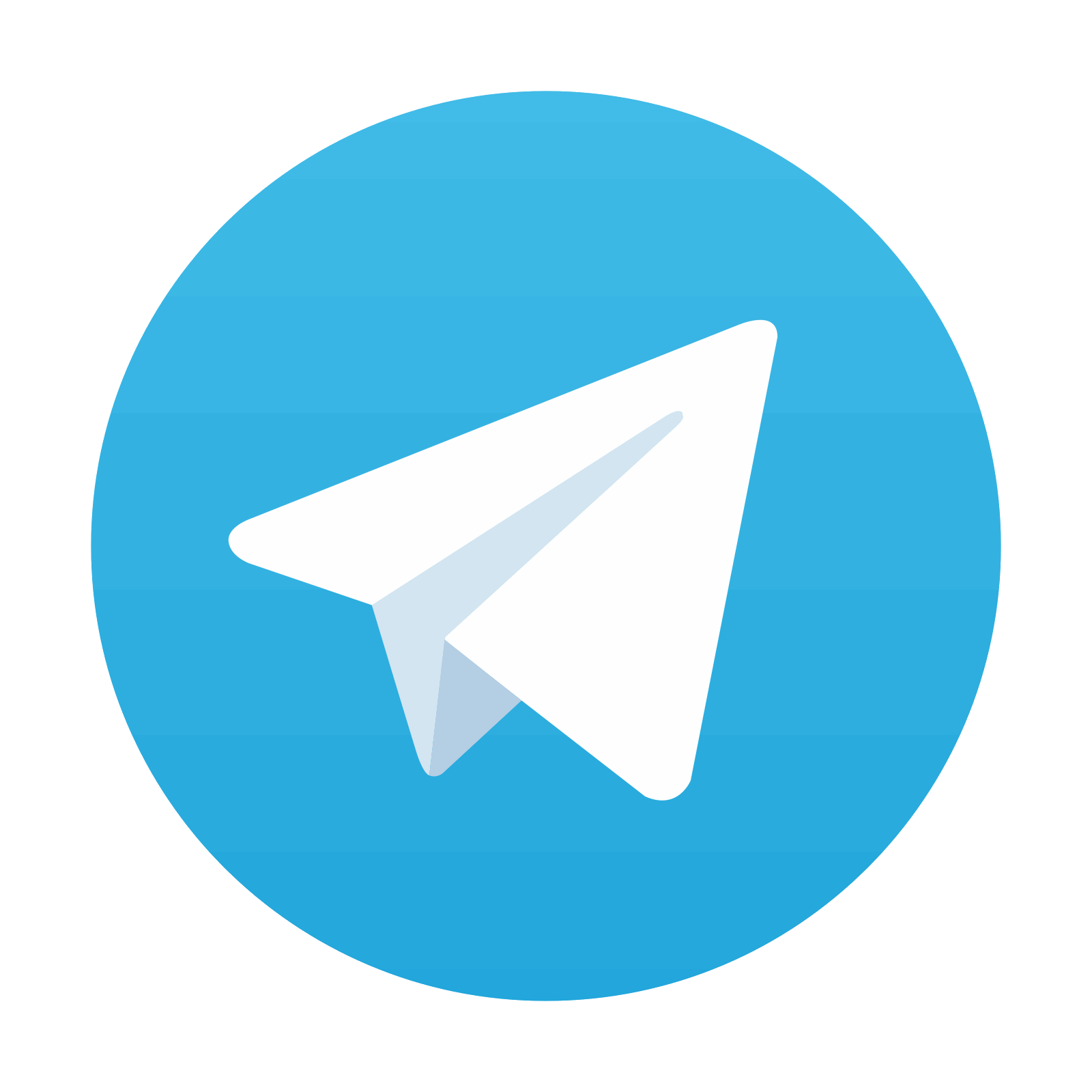
Stay updated, free articles. Join our Telegram channel

Full access? Get Clinical Tree
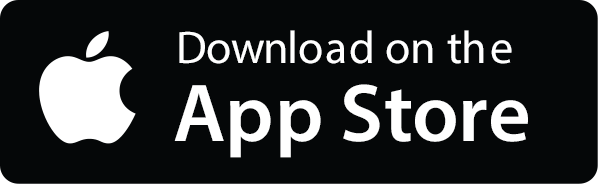
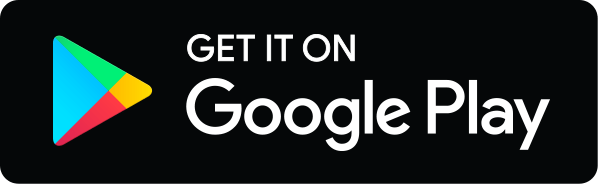
