Pearls
- •
Cardiovascular assessment and monitoring in the pediatric intensive care unit require careful integration of physical findings, laboratory studies, and electronic data to make appropriate therapeutic decisions.
- •
Noninvasive monitoring includes physical examination, chest radiography, echocardiography, blood pressure monitoring, and pulse oximetry. Invasive monitoring includes intravascular and intracardiac monitoring, cardiac output measurements (thermodilution or Fick method), and laboratory studies.
- •
It is important to appreciate the quantity of therapy needed to achieve and sustain adequate systemic oxygen delivery and perfusion pressure if the clinician is to understand the patient’s overall condition, discern the patient’s trajectory, and anticipate associated consequences of current management choices.
- •
Management of patients with single-ventricle physiology (such as the neonate with hypoplastic left heart syndrome) poses several unique challenges to the cardiac intensivist, including optimization of pulmonary-to-systemic blood flow ratios for best systemic oxygen delivery.
Pediatric patients undergoing surgical treatment for congenital heart disease (CHD) or those with severe systemic illnesses such as sepsis and other causes of multiple-organ system failure commonly have impaired cardiovascular function. , In addition to treating the primary disease process, the pediatric intensivist should use strategies to reliably assess and monitor cardiovascular function, which specifically involve assessing adequacy of oxygen delivery (D o 2 ) and systemic perfusion pressure, the primary determinants of tissue oxygenation.
Cardiovascular function
The function of the heart and vasculature is to deliver oxygen (O 2 ) and other nutrients to various tissues in order to meet the metabolic demands of the organism. Mild to moderate depression of D o 2 is normally compensated by augmented O 2 extraction at the tissue level, thereby maintaining a stable level of oxygen consumption (V o 2 ). When D o 2 falls below some critical level, this compensatory mechanism fails, and a state of O 2 supply dependency exists such that any further drop in D o 2 leads to a parallel fall in V o 2 . Under a state of supply-dependent V o 2 , affected tissues and organs attempt to maintain homeostasis partly through anaerobic metabolism. Several studies suggest that the initial metabolic response to hypoxemia or decreased D o 2 differs between the newborn and older ages and varies among different vascular beds. In adults at rest, D o 2 is in great excess of V o 2 . This “O 2 surplus” means that moderate reductions of O 2 transport are generally well tolerated without compromise of V o 2 . In contrast to the adult, the metabolism of the newborn may be particularly susceptible to modest alterations in O 2 transport because of the high resting demands for O 2 , the ease with which these demands can be increased by small environmental changes, and the apparently limited reserve for augmenting cardiac output (CO) or O 2 extraction acutely. , Thus, it is crucial that cardiovascular assessment and monitoring in the pediatric intensive care unit (PICU) involve continuous and reliable evaluation of the adequacy of systemic perfusion and D o 2 to select appropriate hemodynamic support strategies.
Quantity of therapy
If one considers hemodynamic monitoring not only in terms of D o 2 and perfusion pressure but also in terms of what therapy is required to produce a given level of tissue oxygenation, one gains a much better understanding of the overall condition of the patient. It is therefore important to monitor not only D o 2 and perfusion pressure but also the quantity of therapy (QOT) needed to procure and maintain adequate tissue oxygenation. Consider two hypothetical 6-month-old infants, Destiny and Dakota, 2 hours after repair of tetralogy of Fallot. They have identical (and adequate) D o 2 and perfusion pressure, but Destiny has a left atrial (LA) pressure of 6 mm Hg and a right atrial (RA) pressure of 8 mm Hg, whereas Dakota has received volume infusion to achieve an LA pressure of 6 mm Hg and an RA pressure of 15 mm Hg. Assuming that the levels of intravascular volume provided are exactly those needed to achieve the identical D o 2 values and perfusion pressures, it is clear that the physiologies of these two patients are different. The clinician who has learned what to expect relative to the QOT in any given set of circumstances will find Dakota’s sufficient tissue oxygenation only mildly reassuring and asks: is there substantial residual right ventricular (RV) outflow tract obstruction or another problem or problems that I need to know about? Will Dakota have sufficient D o 2 in 10 hours when postbypass myocardial depression is at its worst? Might additional therapy secure adequate D o 2 at a lower filling pressure, thereby minimizing adverse effects of systemic venous hypertension?
As this example illustrates, the QOT concept is useful for three reasons:
- 1.
The QOT is, in part, a function of the patient’s overall condition and can reflect anatomic or physiologic problems that require further exploration.
- 2.
Physiologic trajectory is key, especially early in the course of certain illnesses or after cardiopulmonary bypass, when D o 2 predictably declines over the first 12 hours. Taking into account the QOT relative to tissue oxygenation at any point in time helps one better estimate the likelihood of the need for augmented support (e.g., mechanical support of the circulation) as time passes.
- 3.
Some therapies (e.g., fluid infusion to obtain high filling pressure or high airway pressure), while helpful at a point in time, can be pernicious over the longer run: high venous pressure, especially in infants, causes third spacing of fluid, and the effect of ventilator-associated lung injury on lung function can be devastating. By using high central venous or airway pressures, the intensivist is, in effect, incurring a debt to secure short-term perfusion that will have to be repaid later. Experienced clinicians always take the QOT into account in their work, which influences their level of concern about a patient and guides subsequent timing and choice in adjusting therapy.
Almost any form of therapy might be included in the QOT concept. However, this chapter focuses on medical therapies that have the most important effect on hemodynamics, including D o 2 and perfusion pressure. These therapies include inotropic/vasoactive agents, volume infusion, and airway pressures used during mechanical ventilation. The amount of inotropic and vasoactive drugs administered, assuming that they are used appropriately, seems to be a crude indicator of patient illness. Volume infusion to achieve adequate filling pressure is required even for the normal heart, but the QOT concept applies when higher-than-normal filling pressure is needed to maintain adequate CO. The consequences of high filling pressures are body edema (especially in infants), pleural and other cavity space effusions, and pulmonary edema. If high venous pressure is coupled with systemic hypotension (e.g., with a failing Fontan circulation), there may be critically reduced transtissue perfusion pressure with a potentially negative impact on cerebral and splanchnic perfusion.
With respect to mechanical ventilation, the need for high mean airway pressure (P aw ) is most commonly a reflection of lung disease, but pulmonary edema on a hydrodynamic basis may occasion the use of high P aw for optimal lung recruitment. High P aw can reduce venous return to the heart, increase pulmonary vascular resistance (PVR), and contribute to ventilator-associated lung injury.
Variables that determine tissue oxygenation
Tissue oxygenation is directly related to both D o 2 and systemic arterial blood pressure (SAP). D o 2 , the quantity of O 2 delivered to the tissues per minute, is the product of systemic blood flow (SBF), which equals CO except in patients with certain cardiac malformations, and arterial O 2 content:
DO2(mL/min)=10×CO(L/min)×CaO2(mL/100mL blood)
where CO is cardiac output or SBF (in liters per minute or liters per minute per square meter) and Ca o 2 is quantity of O 2 bound to hemoglobin plus the quantity of O 2 dissolved in the plasma in arterial blood. The O 2 content of arterial blood (mL O 2 /dL blood) equals:
CaO2=(SaO2×Hbg[g/dL]×1.36)+(PaO2×0.003)
where Sa o 2 is arterial O 2 saturation, Hgb is hemoglobin concentration (in grams per deciliter), 1.36 (constant) is the amount of O 2 bound per gram of hemoglobin (mL) at 1 atm of pressure, Pao2 is arterial partial pressure of O 2 , and 0.003 (constant) multiplied by the Pa o 2 equals amount of O 2 dissolved in plasma at 1 atm. The quantity of dissolved O 2 is generally considered to be negligible in the normal range of Pa o 2 . Hypoxia, because of poor gas exchange within the lungs (i.e., intrapulmonary shunt), or in the setting of CHD with right-to-left shunting, is an important determinant of blood O 2 content.
CO is the product of stroke volume (quantity of blood ejected per beat) and heart rate; SAP is determined by CO and systemic vascular resistance (SVR). The four primary determinants of cardiac function are preload (which determines the precontractile lengths of the myofibrils); end-systolic wall stress (function of systemic blood pressure and physical characteristics of the arterial system, ventricular wall thickness, and chamber dimension); myocardial contractility; and heart rate. These determinants of ventricular function can be altered by many factors in the intensive care setting. Preload, or end-diastolic volume, is affected by ventricular compliance (rate and extent of cardiomyocyte relaxation and cardiac connective tissue), intravascular volume, and intrathoracic pressure. Expansion of the heart resulting from transmural filling pressure, rather than the LA pressure per se, determines the force of contraction. Therefore, intrathoracic (or intrapericardial) pressure is a key determinant of preload. Ventricular hypertrophy, vasodilator and diuretic therapies, and positive pressure mechanical ventilation all adversely affect preload. Similarly, cardiac function is inversely related to afterload, or end-systolic wall stress. Anatomic obstructions and systemic or pulmonary hypertension may negatively affect ventricular systolic and diastolic function. Excessively fast or slow heart rates and inappropriately timed atrial contraction (relative to ventricular systole) may negatively affect ventricular filling or function. Finally, myocardial contractility is often negatively affected by the following factors: hypoxemia, acidosis, hypomagnesemia, hypocalcemia, hypoglycemia, hyperkalemia, cardiac surgery, sepsis, and cardiomyopathies.
Monitoring tissue oxygenation
CO can be assessed qualitatively by physical examination and other modalities and quantitatively by a variety of techniques using invasive and noninvasive devices, laboratory data, and other clinical indicators. D o 2 is easily derived if systemic blood flow can be measured, but it is only indirectly inferred if this information is lacking.
Qualitative assessment of cardiac output
Physical examination
The physical examination is often the initial and most common technique used to assess and monitor cardiovascular function. Significantly diminished CO may manifest as diminished peripheral pulses, cool or mottled extremities, and delayed capillary refill. However, certain clinical signs of low CO may be unreliable depending on the particular diagnosis. For example, in the context of cardiac lesions associated with a large arterial pulse pressure (e.g., severe aortic insufficiency and aortopulmonary shunts), peripheral pulses may be increased despite low CO and reduced systemic D o 2 . Patients in septic shock are often peripherally vasodilated and warm despite hypotension and reduced tissue D o 2 . Central cyanosis from either cardiac or respiratory causes results from arterial O 2 desaturation. In contrast, peripheral cyanosis results from vasoconstriction or low blood flow at the microcirculatory level. In some patients, cyanosis is a relatively subtle physical finding, particularly if the patient is anemic or has a dark complexion. Hydration status can be assessed by skin turgor, dryness of mucous membranes, and fullness of the anterior fontanel (in infants), but these manifestations of hydration status relate mostly to interstitial fluid and may poorly reflect intravascular volume, which must be directly measured.
Cardiac auscultation for abnormal heart sounds—including valve clicks, rubs, gallops, and murmurs—may provide the first indication of a significant functional or structural cardiac abnormality, although these sounds do not directly reflect D o 2 . Unfortunately, the lack of a heart murmur, especially in low CO states, does not necessarily rule out a significant residual cardiac lesion. The presence of crackles on pulmonary auscultation, particularly in the older pediatric patient, may signify pulmonary edema. However, crackles are nonspecific and may be caused by lung disease or fluid overload in addition to disorders of cardiac structure or function. Finally, jugular venous distension and hepatomegaly are indicative of high right-sided filling pressures often associated with RV dysfunction.
Chest radiography
Although the chest radiograph is of little value in assessing a patient’s hemodynamic profile per se, it may be helpful for assessing certain aspects related to cardiovascular status. Provided that the chest radiograph is technically adequate, the clinician can assess heart size, contour and configuration, pulmonary vascularity, pleural effusions, lung parenchyma, and abdominal situs. Some of these findings, when abnormal, may help determine the etiology of cardiovascular dysfunction. Chronically increased pulmonary arterial pressure may be indicated by enlarged pulmonary arteries in the hila that radiate toward the periphery of the lung. Conditions that increase pulmonary blood flow (PBF, or Qp) at least twice normal also increase the size of the pulmonary arteries. Increased pulmonary capillary pressure may be inferred by the presence of pulmonary edema. The edema may present as a “fluffy” hilum but may have a more diffuse granular appearance in neonates. Pleural effusions may accompany pulmonary edema, particularly in conditions associated with poorly compensated congestive heart failure. Increased pulmonary venous markings are indicative of elevated pulmonary venous pressures of any cause, although usually from decreased left ventricular compliance or obstruction in the pulmonary veins or mitral stenosis.
In the early postoperative period, the most important information obtained from the chest radiograph is (1) the positions of the endotracheal tube, chest tubes, and intracardiac lines; (2) the presence of extrapulmonary fluid or air; and (3) the presence of pulmonary edema. The cardiothoracic ratio gives a quantitative estimate of cardiac size, which is obtained by dividing the transverse measurement of the cardiac shadow in the posteroanterior view by the width of the thoracic cavity. Cardiomegaly is present if this value is greater than 0.5 in adults and 0.6 in infants. Although useful for assessing left ventricular (LV) enlargement, the cardiothoracic ratio is not as sensitive to RV enlargement. RV enlargement results in lateral and upward displacement of the cardiac apex on the posteroanterior view and filling of the retrosternal space on the lateral view. It is perhaps more important to know what the chest radiograph may not reveal; significant cardiac problems, such as constrictive pericarditis, acute fulminant myocarditis, and even acute pericardial tamponade are often associated with a normal-sized heart on a chest radiograph.
Quantitative assessment of cardiac output
Quantitative measures of CO in the ICU can be obtained by a variety of techniques, including the Fick method, thermodilution, dye dilution techniques (now rarely used), and Doppler echocardiography. Each of the first three methods applies a similar principle of dilution of an indicator: O 2 , cold, or indocyanine green dye, respectively. The change in concentration of a substance is proportional to the volume of blood in which it is being diluted. In general, thermodilution is the method most widely used in the intensive care setting. However, for conditions of low CO, the Fick method is more reliable than the thermodilution or dye dilution techniques. Conversely, the Fick method is less accurate in conditions of high systemic blood flow because of difficulty in measuring narrow arteriovenous O 2 differences in the blood.
Thermodilution technique
The thermodilution technique requires use of a specialized pulmonary artery (PA) catheter. CO is calculated by injecting a known volume of iced water or saline solution into the right atrium (proximal catheter port) and measuring the temperature change at the catheter tip in the PA. CO is calculated by the following equation:
CO=1.08×Vi(Tb-Ti)/Tb(t)dt
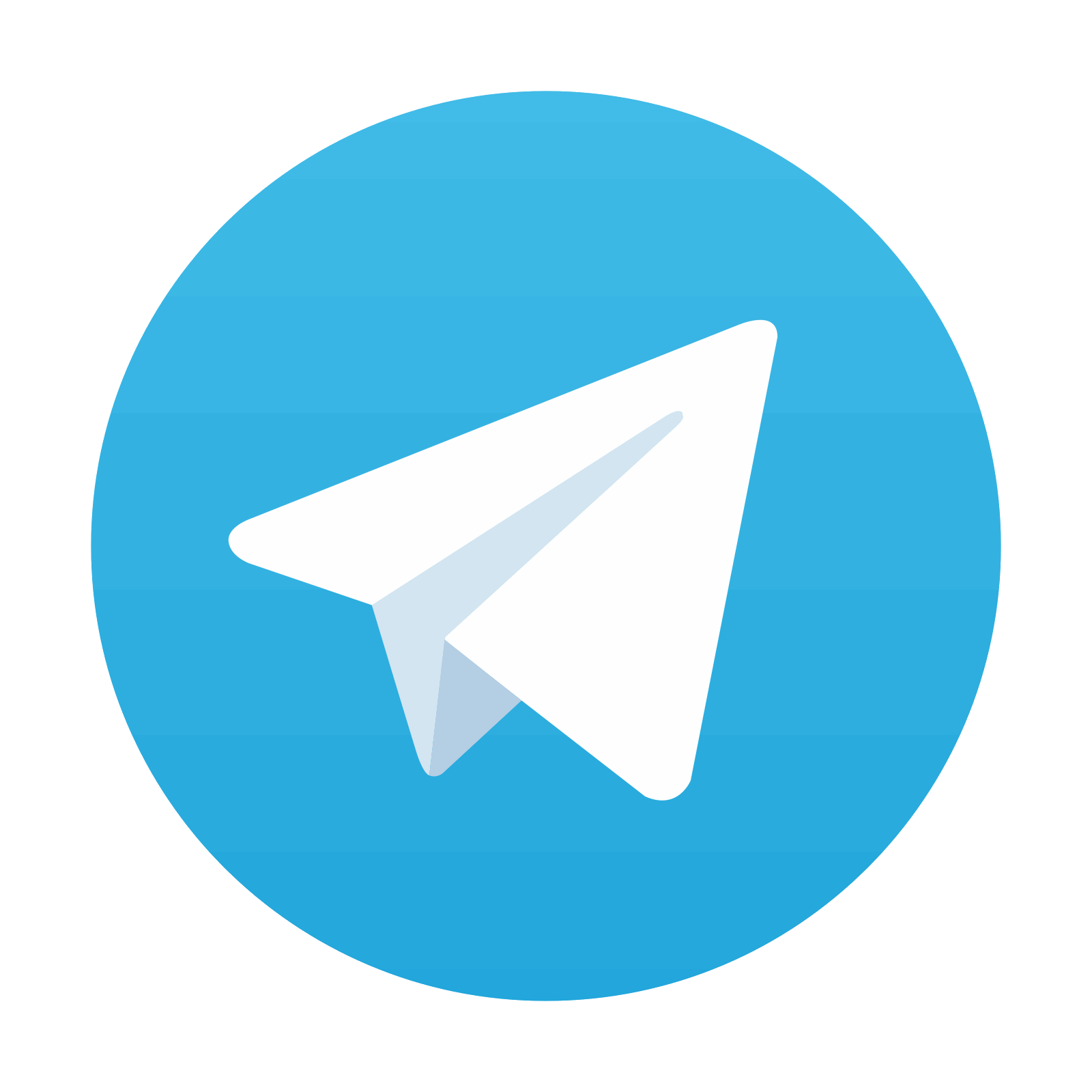
Stay updated, free articles. Join our Telegram channel

Full access? Get Clinical Tree
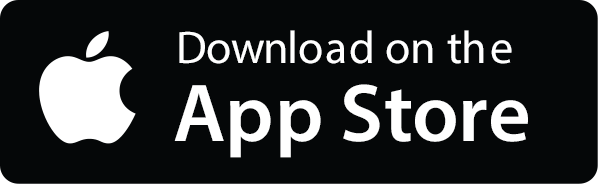
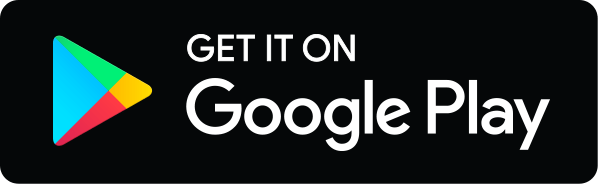
