As our knowledge increases regarding the health benefits of exercise, more and more people attempt to stay healthy and physically fit with sports-related activities. As a result, sports injuries are no longer confined to a small group of competitive athletes but affect an ever-growing segment of the population. Improper training techniques, overambitious routines, and the use of faulty equipment have led to an increase in sports injuries (especially overuse injuries) and result in pain. In the pediatric population, children engage in highly competitive sports with training schedules that put them at increased risk for injury. Elite athletes, under the pressure of commercial interests and more widely applied knowledge about exercise physiology and training methods, are driven to greater extremes in order to gain small but significant advantages over the competition. This has led to overambitious workouts with inadequate rest periods. Many athletes suffer from chronic pain and injury as a result.
This chapter presents a review of the essential elements of bone, joint, tendon, ligament, and muscle physiology to lay the foundation for understanding both acute and repetitive stress injuries that are commonly seen in athletics. We will provide treatment strategies for both acute and more chronic pain syndromes.
Bone is composed of organic proteins, matrix, and cells. The organic component of bone is 90% collagen type I and provides the tensile strength. The mineral phase of bone matrix accounts for 50% of the volume and 65% of the weight of bone and is composed of highly structured hydroxyapatite crystals and amorphous calcium phosphates. Bone cells account for only 3% of bone volume and include osteoblasts, osteoclasts, and osteocytes. Bone remodeling is ongoing throughout life and occurs predominantly at the trabecular part of the skeleton. Wolff’s law of adaptation states that mechanical remodeling of bone occurs in response to deforming strain. Both osteoclasts (responsible for bone resorption) and osteoblasts (responsible for bone formation and remodeling) are involved. The exact mechanism of how mechanical strain activates osteocytes to initiate remodeling is still unknown, but both chemical messengers (dependent on the prostaglandins PGI2 and PGE2, insulin like growth factor 1 [IGF-1], and parathyroid hormone) and piezoelectric effects are believed to be involved.1
High levels of physical activity and stress loading in athletes increase bone density. The degree of increase in bone density is proportional to the level of stress loading accomplished in the athletic activity. For example, the bone mineral density (BMD) of the distal femur is found to be highest in world-class weightlifters followed by throwers, runners, soccer players, and then swimmers. This positive effect on BMD from mechanical loading is also observed in young women skaters and may counter some of the adverse estrogen-deficient effects on bone density seen in woman who are thin and amenorrheic.
Studies on both the elderly and the young confirm that strength training at higher loads increases BMD more compared with endurance training at low weights with high repetitions.2
Diarthrodial or synovial joints are capable of large degrees of motion and, under normal circumstances, tolerate high levels of friction, shear, and wear with little deterioration throughout a normal life span. A typical knee or hip joint may withstand loads up to six times body weight on a repetitive basis for up to a million times a year.3 Synovial fluid and soft connective tissue are common to all diarthrodial joints. The soft connective tissue includes the articular cartilage, joint capsule, meniscal cartilage, and ligaments. The primary load-bearing structure of the joint is the articular cartilage. Cartilage is primarily made up of type II collagen with up to 150 proteoglycan (PG) monomers linked to a central core of hyaluronic acid (Fig. 53-1). The link between PG and hyaluronic acid is essential for the structural integrity of cartilage. These macromolecules are highly hydrophilic but, because they are confined in the semirigid collagen matrix, reach only 20% of their theoretical swell volume. The absorbent effects caused by the embedded PG are resisted by the tension that develops in the collagen matrix, and it is this balance of forces that is essential for modulating the compressibility of the structure under various loads.
FIGURE 53-1.
(A) Schematic depiction of the molecular arrangement of the PG monomer. (B) The PG aggregates along a hyaluronate chain. (Reproduced with permission from Zimmerman JR, et al. In Chapter 8: Downey JA, Myers SJ, et al, eds. The Physiological Basis of Rehabilitation Medicine. 2nd ed. Boston, MA: Butterworth-Heinemann, 1994.)

Immobilization of a joint for prolonged periods causes significant loss of PGs in the articular cartilage, which results in a loss of resistance to compression. In the recovery process after injury, both joint range of motion (ROM) and stress loading are necessary to reverse these changes.
Tendons and ligaments are made up of highly organized collagen fibers (predominantly type I) arranged in a linear fashion. Tendons transmit the force generated by muscle actions to bones and generate movement about a joint. Ligaments are capsular if they extend off the joint capsule or accessory when extending between bones. In contrast to tendons, ligaments prevent excessive movement and contribute to joint stability. Both tendons and ligaments are made up of collagen fascicles that spiral on each other with successive folds or crimps that permit stretch and buffer elongation. The blood supply to tendons and ligaments is sparse compared with joints, muscle, and bone. For example, avascular regions are found in the central region of the anterior cruciate ligament (ACL) of the knee and the supraspinatus tendon of the shoulder.
Tendons are often surrounded by synovial sheaths when there is a significant pulley action present (e.g., the digit flexor tendons of the hand). At the junction with the bone, or enthesis, small synovial bursa are often present to prevent friction (e.g., greater trochanter bursa, retrocalcaneal bursa, and subacromial bursa).
Golgi and Pancinian organs lie at the myotendinous junction and transmit information to the central nervous system (CNS) about muscle tension and pressure. Tendons demonstrate nonlinear deformation in response to stress. In the first phase, collagen fibers straighten and elastic fibers elongate. The second phase requires much greater force and is characterized by breaking of collagen cross-links and disruption of smaller collagen fibers. Finally, increased forces result in tendon rupture, and failure occurs when a tendon is stretched 5% to 8% beyond its resting length (Fig. 53-2).
FIGURE 53-2.
Load-deformation (strain) curve for ligaments and tendons. The “toe” region of the curve is within the normal physiologic range. Greater than 4% strain causes tissue damage. (Reproduced with permission from Oxford Textbook of Sports Medicine 2nd Edition edited by Harris (1998) Fig. from Chp.4.4.3 “Tendon/Ligament Basic Science” by Oakes p. 584. By permission of Oxford University Press.)

Changes in activity significantly affect tendons and ligaments. Physical training increases the weight and size of tendons and ligaments and increases the cross-links between collagen fibers. Immobilization decreases these cross-links and thus diminishes the tensile strength.
Skeletal muscle makes up approximately 40% to 45% of the total body weight. There are two major types of muscle fibers (type I and II), which were originally identified with basic histochemical staining techniques (Fig. 53-3). These staining differences correlate with underlying differences in structural, contractile, and biochemical properties. Subtypes of type I and II have been characterized as well, and Table 53-1 summarizes the differences among fiber types.
FIGURE 53-3.
Photomicrograph of vastus lateralis from a 21-year-old man stained for ATPase at pH 4.6 showing type I, type IIA, and type IIB fibers. (Reproduced with permission from Lieberman JS, et al. Skeletal muscle: structure, chemistry, and function. In: Downey JA, Myers SJ, et al, eds. The Physiological Basis of Rehabilitation Medicine. 2nd ed. Boston, MA: Butterworth-Heinemann, 1994: 88.)

Muscle Fiber Type Classification
| Type I | Type IIA | Type IIB |
Glycogen content | Low | High | High |
Mitochondria | Many | Many | Few |
Oxidative enzymes | High | Intermediate | Low |
Contraction speed | Slow | Fast | Fast |
Fatigue resistance | High | High | Low |
Aerobic capacity | High | Medium | Low |
Anaerobic capacity | Low | Medium | High |
Strength | Low | High | High |
The response of muscle to changes in levels of physical activity can be profound. There are two basic forms of muscle actions: static (or isometric), in which there is no joint movement, and dynamic, in which there is a change in the length of the muscle and joint movement occurs. Dynamic actions can be further divided into concentric, in which the muscle shortens during the increased load (triceps in a shot put hurl), and eccentric, in which the muscle lengthens during the increased load (quadriceps when landing from a jump). Eccentric actions demand a lower metabolic expenditure compared with concentric actions but carry an increased risk of muscle damage and tearing because of higher forces.
Increases in muscle mass with resistance training are primarily brought about by type II fiber hypertrophy (with a contribution from type I hypertrophy) rather than by muscle fiber proliferation. It has been found in animal models that repetitive stretching of muscle fibers alone is sufficient to cause massive increases in gene expression of contractile proteins, causing muscle hypertrophy. The resistance to fatigue that develops with endurance training results from a number of factors, including increases in capillary supply and mitochondrial content (i.e., increase in oxidative capacity) of the muscle fibers and metabolic adaptations favoring fat metabolism and glycogen sparing. It is still a contested question whether transformation of fiber types contributes to the adaptation of muscle to various training stimuli. The predominance of type I fibers in distance runners probably results from genetic influences, environmental factors such as training, and the interaction between the two. Some studies have shown changes in type IIB to type I with high-intensity interval training, but others did not show such changes or have shown only a shift from type IIB to type IIA with endurance training. In disuse atrophy, the reduction of muscle bulk can be profound, with up to 30% reduction in cross-sectional area after 1 month of immobilization. Both type I and type II fibers are reduced in size to varying degrees depending on the individual.4
Aging alone has been thought to lead to the loss of muscle mass and strength. There is a loss of both cross-sectional area and total number of muscle fibers with age, predominantly affecting type II. This loss in muscle mass coincides with loss of bone density. However, recent studies have supported the idea that many of the changes seen are not inevitable but are the result of decreased activity. The positive training effects seen on muscles in young people also occur in elderly adults. Even in the 10th decade of life, an elderly person can make significant increases in muscle mass and strength if given a progressive resistance-training program. Similar positive changes in muscle respiratory capacity seen in young people can occur with endurance training in elderly adults.5
Finally, the CNS also changes with training. This is evidenced by the marked improvement in performance that can occur with training in a specific activity over time, with much less dramatic changes in peripheral muscle strength. For example, during a 12-week period of training that included lifting boxes from the floor to the waist by knee extension, a 200% increase in weight was achieved with only a 15% increase in absolute isometric strength of the quadriceps muscle group. Also, the untrained contralateral limb will show an improvement in performance when the ipsilateral limb is repetitively trained, suggesting that the central mechanism plays a role. These changes are felt to result from neural adaptations, including synchronization of motor units in the trained activity with a more advantageous balance of agonist versus antagonist muscle activation.
After an acute injury, there is a release of molecules, creating an “inflammatory soup” at the cellular level, including nerve growth factor, bradykinin, serotonin, and prostaglandins. These molecules excite nociceptors, thereby stimulating afferent pain signaling and neurogenic inflammation. Neurogenic inflammation is the process by which active nociceptors release neurotransmitters such as substance P to induce vasodilation, leak proteins and fluids into the extracellular space near the end of the nociceptor, and stimulate immune cells which perpetuate the inflammatory soup.6
These processes are evident in acute injuries throughout the musculoskeletal system. For example, human synovial joints are richly innervated in type IVa free nerve endings. These are found in joint capsules, tendons, retinacula, fat pads, synovium, subchondral bone, and surrounding ligaments. They detect pressure and mediate proprioception during joint movement. Muscle and fascia are rich in free nerve endings which detect substance P and mediate nociception. Cadaver studies have shown that substance P and calcitonin gene-related peptide (CGRP) are increasingly expressed in degenerative knees versus healthy subjects. Anatomic studies demonstrate that periosteum is rich in myelinated and unmyelinated sensory fibers, CGRP, and sympathetic nerve fibers expressing tropomyosin receptor kinase A. Hyaline cartilage is avascular and aneural, but cartilage tears initiate local inflammation, which triggers a nociceptive response in adjacent tissue.7
The neurogenic inflammation produced after tendon injury includes molecules such as catecholamines, acetylcholine, and glutamate. In addition to its production by nerves, increased levels of glutamate in damaged tendons have been linked to local tenocyte production as well. Substance P may signal tenocytes to modulate their expression of growth factors, proteinases, and proteinase inhibitors. There are more mast cells in tendinopathic tissue as well. Neuropeptides may stimulate mast cells to degranulate and release histamine. Histamine promotes axonal stimulation and neuropeptide release, thereby perpetuating the neurogenic inflammation.8–10
A normal tendon is sparsely innervated. However, paratendinous tissue is well supplied with nerves as they run in proximity with the vascular bundle. Most of these nerves are sensory, responding to substance P and CGRP. There is evidence that tendon injury encourages paratendon nerve sprouting and penetration of repairing scar tissue within the tendon body. This is an example of the “failed healing response” that has been demonstrated in tendinopathy. The degeneration and abnormal regeneration includes an aberrant increase in microvessels and nerves, abnormal quality and accelerated remodeling of extracellular matrix (ECM), and increased local metabolism, all within damaged tendons.8–14
In a retrospective study by Sanchis-Alfonso and colleagues,11 the nerve morphologies at the patellar tendon–bone junction of 17 surgical specimens collected during tendon repair were examined. Small nerve fibers discovered in the patellar osteotendinous junction, with increased density at Hoffa’s fat pad, led to the hypothesis of “nerve sprouting.” This process is similar to that of regeneration after sectioning a nerve. At the osteotendinous junction, there are small nerve fibers which leave the main nerve and disseminate into fibrous tissue. Hoffa’s fat pad and the osteotendinous junction are potential sources of nociception resulting from chronic repetitive impingement and compressive forces on the tendon. The samples also revealed neuromatous changes. Nerves were disorganized at the osteotendinous zone with bundles of small nerve fibers immersed in fibrous stroma.8,11 Similarly, nerve sprouting has been observed in Achilles tendinopathy. The abnormal nerve endings discovered in the Achilles tendon were positive for substance P.9
In conjunction with aberrant nerve growth, there is also evidence of altered blood supply to the damaged tendon. Tendon innervation is crucial to normal healing. There is an increase in sensory nerves 2 to 6 weeks after an acute injury; sympathetic nerves are abundant 8 weeks later in areas of repair. Sensory neuropeptides increase blood flow by vasodilation and angiogenesis, and then later sympathetic neuropeptides downregulate blood flow as healing progresses and the metabolic requirement lessens. However, in a damaged tendon, there is increased blood flow.8,9,11
For example, increased vascular innervation is most prominent at Hoffa’s fat pad adjacent to the inferior pole of the patella in patellar tendinopathy. An increased number of microvessels are associated with the abnormal nerve supply to damaged Achilles tendons as well. Particularly, studies have found a reduced number of sympathetic nerves which modulate blood supply. Perhaps the loss of sympathetic control explains an observed increased blood flow on Doppler ultrasound of tendinopathy.10
Neuropathic pain has been studied as a mechanism of chronic tendinopathy. A study by van Wilgen and Keizer15 examined the presence of “sensitization” by testing for the presence of allodynia with light touch and pressure application to the injury site compared with the contralateral side. There is statistical significance in the fact that sensitized patients suffer more frequently from chronic injury. Frequent injuries promote functional and structural changes within the nervous system that in turn aggravate pain and disability. The authors state that if sensitization is present, antineuropathic medications such as tricyclic antidepressants (TCAs) and anticonvulsants may be effective treatment. Also, treatments that focus on improving functionality, such as eccentric training, instead of pain relief may desensitize the CNS.15
Injuries in sports can be caused by a sudden overload to structural components of the bones, joints, and soft tissue or from chronic overuse. The forces that various structures must withstand during athletic activity are enormous. For example, a runner weighing 165 lb will absorb a total of nearly 500,000 lb of force on each foot during a 1.6-km run. Various extrinsic and intrinsic factors influence the type and severity of injury listed in Table 53-2. In the majority of injuries that are not traumatic, there has usually been a sudden change in the training routine that underlies the onset of pain. Typically, either there was a recent initiation of a new sport activity or there was a recent marked increase in training load involving changes in frequency, duration, and intensity.
Factors That Influence Injuries in Healthy Athletes
Extrinsic Factors | Intrinsic Factors |
Excessive loads | Structural malalignment |
Training errors | Muscle weakness or imbalances |
Adverse environment (e.g., training surface) | Decreased flexibility |
Poor equipment | Joint laxity |
Sport rules | Gender or age |
Bone injury can occur with the extremes of repetitive forces seen in athletics and is characterized by the formation of stress fractures. The incidence of stress fractures in athletic populations of men is approximately 2% and of women from 3.8% to 10%. Up to 49% of women track runners with fewer than five menstrual periods a year develop stress fractures, suggesting that a hypoestrogenic state increases the risk. In runners, stress fractures account for nearly 25% of all injuries.16 Stress fractures are seen more commonly in different bones depending on the athletic endeavor17 (Table 53-3).
Stress Fracture Sites
Fracture Site | Return to Sport | Comments |
Lower Extremity | ||
Femoral neck | 7–12 weeks | High incidence of late nonunions and avascular necrosis Surgical management often necessary |
Femoral shaft | 8–14 weeks | Vague thigh or groin pain often only clue |
Tibia | Prolonged | Common in runners, dancers, and jumping sports; casting may be necessary, most common stress fracture in the LE |
Fibula | 6 weeks | Differential diagnosis includes compartment syndrome and peroneal nerve entrapment |
Tarsal navicular | 16–20 weeks | Commonly missed; 6 weeks of casting, followed by semirigid shoe with medical arch necessary |
Metatarsal | 4–6 weeks | March fracture; second and third rays most common; rigid shoe recommended to promote healing |
Upper Extremity | ||
Ribs | 6–8 weeks | Rare; seen in rowers and baseball players |
Clavicle | 8 weeks | Rare; found typically with throwing activity |
Scapula | 6–8 weeks | Rare; intense weightlifting is common etiology |
Humerus | 10–16 weeks | Seen in adolescent athletes |
Olecranon | 8–12 weeks | Seen in throwers and gymnasts |
Ulna | 4–6 weeks | More common; seen in a variety of sports |
Radius | 12–16 weeks | Commonly seen in gymnasts |
Metacarpals | 4 weeks | Tennis racquet gripping or ball gripping underlie injuries |
The most common joints injured in sports are the shoulder and the knee. The shoulder is the most unstable joint in the body and depends almost exclusively on ligaments and the tendons of the intrinsic muscles to maintain a balance between the extremes of ROM and stability. As a result, these ligamentous and tendinous structures are commonly injured in sports when this tenuous balance is tested to achieve optimal performance. Both acute and chronic repetitive injuries are seen. Table 53-4 lists some less common shoulder injuries in the athletic population.18
Uncommon Causes of Shoulder Injuries in Athletes
Causes | Comments |
Neurovascular | |
Suprascapular nerve compression | Commonly caused by traction or blunt trauma; seen in volleyball players |
Long thoracic nerve palsy | Scapular winging caused by serratus anterior, weakness |
Axillary nerve compression | Seen in throwing athletes |
Spinal accessory nerve injury | Trapezius weakness with shoulder sagging after trauma |
Thoracic outlet syndrome | Brachial plexus compression; often seen after clavicle fracture |
Effort thrombosis | Axillary vein injury commonly with repetitive throwing |
Soft Tissue | |
SLAP lesions | Deceleration injury to anterior glenoid labrum transmitted by biceps |
Posterior-superior impingement | Injury to posterior-superior labrum in throwing sports |
Tendon ruptures | Biceps, pectoralis major, subscapularis, coracobrachialis, and serratus |
Snapping scapula syndrome | Usually myofascial in origin |
The knee is typically injured acutely because of sudden forces that overwhelm the supporting ligaments. Often, if the loads are sufficient to disrupt the relatively tough intrinsic ligaments of the knee, the cartilaginous structures such as the menisci will also be torn. Common causes of knee pain in athletes include meniscal tears, patellofemoral pain syndrome, ligamentous injuries, patellar tendinitis, and osteochondral injury. In the juvenile population, apophysitis of the tibia (Osgood-Schlatter’s syndrome) is often seen. Table 53-5 lists some less common injuries to the knee in the athletic population.19
Less Common Causes of Knee Injuries in Athletes
Causes | Comments |
Neurovascular | |
Saphenous nerve entrapment | Fascia of sartorius and vastus medialis 10 cm proximal to medial condyle of femur |
Soft Tissue | |
Iliotibial band syndrome | Pain at site of friction over lateral femoral condyle; common in runners and cyclists |
Popliteus tendinitis | Commonly seen with downhill walking or running |
Hoffa’s disease | Infrapatellar fat pad syndrome; injured during repetitive extension |
Semimembranosus tendinitis | Pain at posteromedial corner of knee; more common with runners |
Pes anserinus tendinitis | Common insertion of sartorius, gracilis, and semitendinosus |
Tibial collateral ligament bursitis | Deep to medial collateral ligament; pain without locking or instability to valgus stress |
Just as in the occupational arena, low back pain (LBP) is common in sports. Pain can develop as a result of any of the causes seen in nonathletes. Of particular interest to adolescent athletes is the development of symptomatic spondylolysis with pars interarticularis defects. Spondylolisthesis can occur with bilateral pars defects. Excessive loading of spinal elements during growth in adolescence is believed to be harmful and puts athletes in this age group at greater risk for developing LBP than sedentary control participants. The cumulative prevalence of LBP in juveniles is 30% with only 8% having more chronic or recurrent problems.20 Among adolescent athletes referred for evaluation of LBP, up to 47% have been found to have spondylolysis. Stress loading in extension and rotation is thought to be particularly problematic, and as a result, there is a higher prevalence of spondylolysis in sports such as soccer, tennis, wrestling, gymnastics, football, volleyball, and rugby. The level most frequently involved is L5 (88.6%) with L4 seen less often.21 Spina bifida occulta has been found to be more prevalent in patients with spondylolysis.
Tendinitis and muscle strains account for 30% to 50% of all sports injuries. A recent review of the literature on the etiology and treatment of tendinitis suggests that there are few well-controlled prospective studies that can guide care.22 Nevertheless, some basic concepts are generally accepted. The forces that injure a tendon can be either extrinsic to the tendon itself, causing an impingement on the tendon, or intrinsic, related to excessive stretch forces on the tendon during activity. A common example of an extrinsic tendinitis is shoulder impingement syndrome, in which there is inadequate space between the humeral head and the acromion for free passage of the rotator cuff tendons during overhead activity (throwing and swimming), leading to direct tendon trauma. Intrinsic tendinitis commonly results from repetitive overuse or sudden excessive loading as is seen with weightlifting. Eccentric loading of the tendon applies greater forces and under many circumstances can be more traumatic. This occurs when the muscle–tendon complex is lengthening during an action. An example of acute eccentric loading to the patellar tendon occurs when landing from a jump as the knee bends and the quadriceps muscle lengthens to absorb the shock. In addition, a lengthening of the muscle to maximize force generation briefly precedes most concentric actions. This puts the maximum force on the tendon when the tendon is elongated, potentially causing damage.
Acute tendinitis usually is due to a traumatic event and may cause partial or complete rupture of the tendon. In this case, inflammation leads to healing and repair. In contrast, repetitive loading may cause chronic tendon injury or tendinosis. Histologically, there is little inflammation but instead angiofibroblastic changes. The “failed healing response” was discussed previously. The process of acute healing is normally dominated by the growth of type 1 collagen. In contrast, type 3 collagen dominates, producing a thinner, weaker structure in the process of chronic healing. Ultrasound findings include thickened tendon, local hypoechoic areas, irregular fiber structure, and neovascularization. The tendon has a 7.5 times lower oxygen consumption rate than muscle. This gives the advantage of allowing anaerobic metabolism to bear high loads for long periods of time. However, this also puts the tendon at a disadvantage because of slow healing.12–14,23
As the tendon’s structure becomes disrupted, it loses the ability to absorb forces. Risk factors for the development of tendinosis include age over 35 years, frequency of training more than three times per week, duration of training longer than 30 minutes per session, “demanding” training intensity, and female gender. With advancing age, tendons lose strength, elasticity, and the ability to repair. An aggressive training regimen does not allow time for microtrauma to repair. There is also the observation that the healing responses in load bearing, such as Achilles and patellar tendons versus non–load-bearing tendons, such as the wrist extensors, are different and possible because of varied mechanical stimulation.12,24
Ligament injuries, or sprains, commonly occur in the knees and ankles of athletes. The etiology of such injuries is almost always the result of a sudden excessive force on the ligament. Examples include a sudden valgus stress to the knee with a football tackle at the knees causing disruption of the medial collateral ligament and a sudden change in direction on a tennis court or football field with the foot firmly planted, which can lead to ACL injury. Joint stability may be significantly impaired with serious ligamentous injury and, if improperly treated, can lead to chronic stresses to the joint and supporting muscles and tendons.
Muscle injuries occur either from an acute excessive load or from excessive chronic use. Myotendinous failure is a function of the force applied and the strength and level of fatigue of the muscle. The myotendinous junction is highly infolded, increasing the contact surface area by 20 times to provide added strength. As a result, tears occur near but not at the true histologic junction. If severe enough, muscle and tendon tears can occur and be associated with hematomas. Muscles that cross two joints or have a higher percentage of type II fibers are more prone to strain patterns. Examples of such muscles include the hamstrings, the rectus femoris, and the gastrocnemius.
Eccentric actions put muscles at greater risk for strain and can cause the disruption of contractile elements at the Z lines. Delayed onset of muscle soreness is more common after eccentric loading.25 This condition usually appears 24 to 48 hours after an exercise bout and is associated with high serum levels of intramuscular enzymes such as creatine kinase.
Other causes of muscle pain in sports include muscular contusions by blunt trauma. Myositis ossificans is a complication of muscle contusion characterized by intramuscular calcifications. Exertional rhabdomyolysis can also occur, with or without direct trauma, and is potentially fatal. Complications include compartment syndrome in the affected limb caused by excessive muscle swelling and renal failure.26 Compartment syndrome can also occur in chronic situations and results from repetitive injury to a muscle group, leading to swelling and increased pressures in the muscle with exertion (often seen in the lower extremities of runners).
The differential diagnosis of acute or chronic sports injury should include neurologic processes. In fact, the five most common causes of chronic lower extremity pain are medial tibial stress syndrome; stress fracture; chronic exertional compartment syndrome; popliteal artery entrapment syndrome; and importantly, nerve entrapment. Burning pain during activity, exacerbated by exercise, with regional motor and/or sensory symptoms should include a differential of nerve entrapment. Trauma is a primary cause. Electromyography and nerve conduction studies are helpful in diagnosis.27–29
An example of nerve entrapment in the upper extremity is carpal tunnel syndrome. This is also a common injury in nonathletes. In athletes, it is caused by repetitive wrist motion, at times with prolonged dorsi- or palmar flexion. It is most often observed in those participating in racket sports, cycling, or gripping sports. It usually presents with paresthesias at the thumb, index, long digit, or forearm. There may also be hypoalgesia in a dermatomal pattern. The history usually includes nocturnal pain. Tinel’s and Phalen’s signs are less accurate than the observation of weak thumb abduction, diminished grip strength, thenar atrophy, and loss of two-point discrimination.27,30,31
An example of nerve entrapment in the lower extremities is tarsal tunnel syndrome. The tibial nerve or its branches become entrapped, usually as a result of trauma associated with a contusion or poorly fitting footwear. It may be caused by compression from a space-occupying lesion such as venous stasis, local ganglion, tenosynovitis, os trigonum, tumors, bone fragments, or accessory calf muscles. The most common cause in sports injury is biomechanical, including excessive hindfoot pronation and joint hypermobility. This may contribute by provoking a tense abductor halluces muscle, which traps the tibial nerve. Patients may relate cramping, burning, or tingling at the medial ankle or plantar foot. Symptoms worsen with activity and improve with rest, elevation, and looser footwear. Rest or night pain is common. The examination may reveal abnormalities such as forefoot pronation, claw toe, talipes calcaneus, and calcaneovalgus. Provocative testing entails sustained passive eversion and great toe dorsiflexion to exert tension on the nerve. Diagnostic ultrasound is useful to directly observe compressive mass lesions or focal change in the tibial nerve itself (see Fig. 53-4). The diagnosis includes three criteria: (1) foot pain with paresthesias, (2) positive Tinel’s sign, and (3) confirmation on electrodiagnostic studies.27–29,32,33
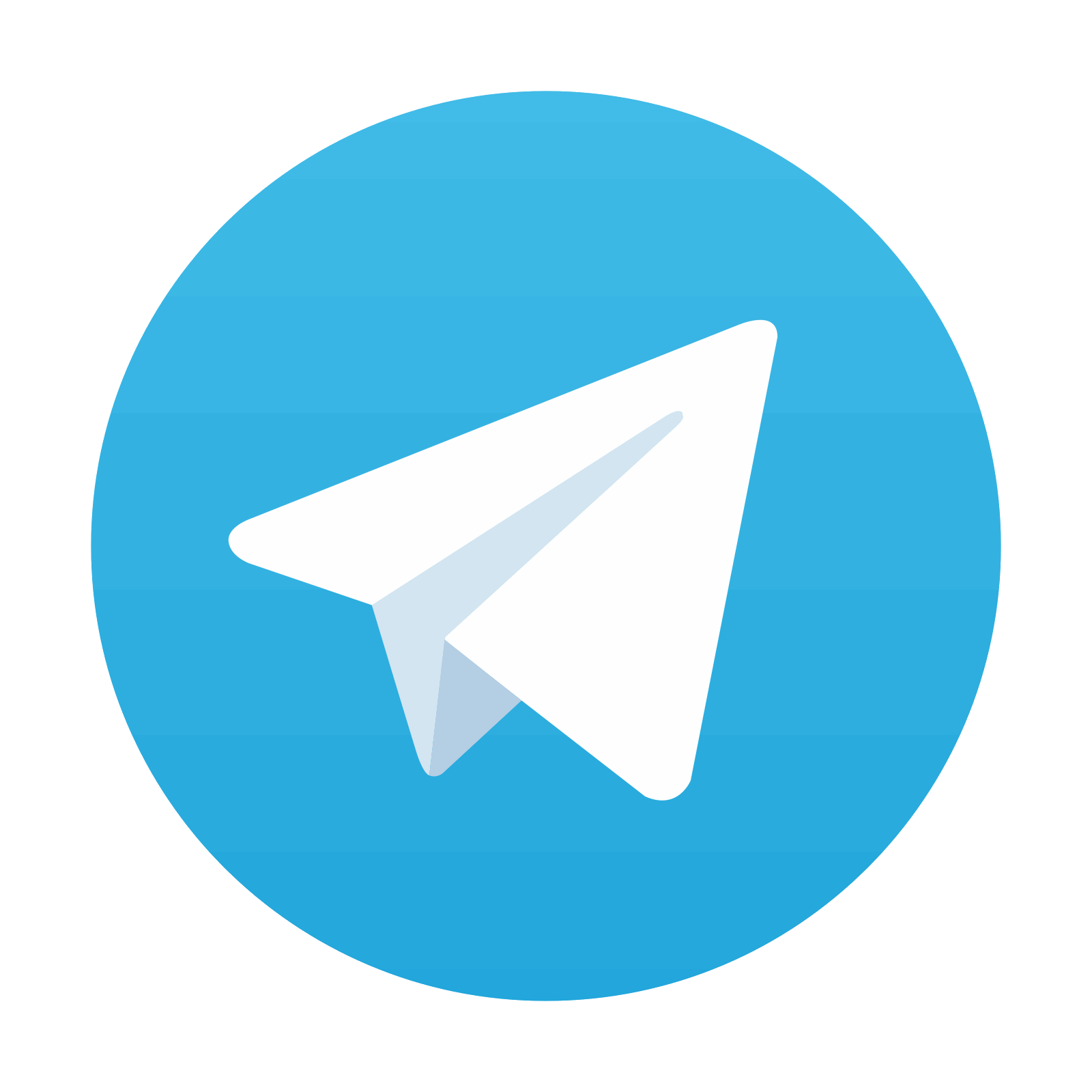
Stay updated, free articles. Join our Telegram channel

Full access? Get Clinical Tree
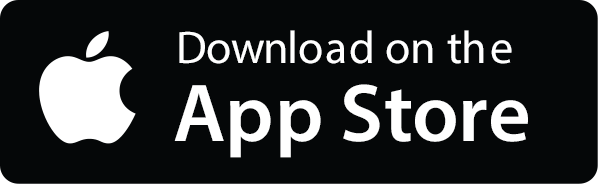
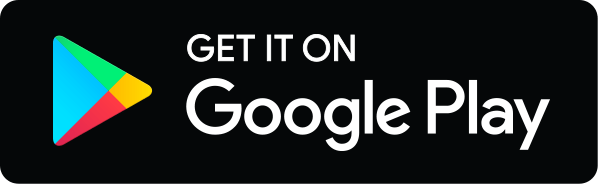